(1)
Department of Nuclear Medicine, Kuwait University, Safat, Kuwait
13.1 Nuclear Hematology
13.2 Blood Cells
13.3 The Bone Marrow
13.4 Erythropoiesis
13.6 Ferrokinetics
13.9 Megaloblastic Anemia
13.9.2 Schilling’s Test
13.10 The Spleen
13.10.1 Spleen Imaging
13.10.2 Measurement of Splenic Function
13.11 Bone Marrow
13.12 Blood Platelets
13.12.1 Measurement of Platelet Survival
Abstract
Nuclear hematology deals with the use of radionuclides or radiopharmaceutical agents in the study of the pathophysiology, diagnosis, and therapy of hematological diseases. Radionuclides are now widely used to label the formed elements of the blood (random labels) to trace their biological distribution, function, and life span in vivo as well as to study the proliferation and differentiation of hematopoietic progenitor and precursor cells in the bone marrow (cohort labels). The other major applications of nuclear hematology include the determination of spleen size, splenic sequestration of blood cells, and investigations relating to the absorption, metabolism, and utilization of hematopoietic nutrients such as iron, vitamin B12, and folate. This chapter reviews the pathophysiological basis of the important applications of radionuclides in the field of hematology.
13.1 Nuclear Hematology
Nuclear hematology deals with the use of radionuclides or radiopharmaceutical agents in the study of the pathophysiology, diagnosis, and therapy of hematological diseases. Radionuclides are now widely used to label the formed elements of the blood (random labels) to trace their biological distribution, function, and life span in vivo as well as to study the proliferation and differentiation of hematopoietic progenitor and precursor cells in the bone marrow (cohort labels). The other major applications of nuclear hematology include the determination of spleen size, splenic sequestration of blood cells, and investigations relating to the absorption, metabolism, and utilization of hematopoietic nutrients such as iron, vitamin B12, and folate.
13.2 Blood Cells
The various blood cells develop from the stem cells by multiplication, differentiation, orderly maturation, and release of mature cells from the bone marrow to the peripheral circulation. A dynamic equilibrium is maintained between cell death in peripheral circulation and compensatory production of these cells in the bone marrow creating a steady state of “normal blood cell numbers” in physiological conditions.
The circulating blood cells are highly specialized cells with distinctive morphologies and functions. The red blood cells (mature erythrocytes), each approximately 8 μm in diameter, contain hemoglobin in a reduced (ferrous) state for successful gaseous exchange in the tissues.
The mature leukocytes (white blood cells) comprise two broad groups, phagocytes including granulocytes and monocytes, and the immunocytes (lymphocytes). Normally only the mature leukocytes are found in the circulating peripheral blood. These include mature granulocytes (neutrophilic polymorphonuclear leukocytes, eosinophils, and basophils) (Fig. 13.1), monocytes, and lymphocytes. The mature neutrophils contain several types of granules and other subcellular organelles. These include the primary or azurophilic granules and specific granules. The primary granules appear at the promyelocytic stage, gradually decrease as the cells mature, and contain many antimicrobial compounds which include myeloperoxidase, defensins, and lysozyme. The primary granules also contain organelles such as lysosomes, which fuse with phagocytic vesicles and deliver antimicrobial contents to the ingested organisms. Specific granules also called secondary granules of the neutrophils may fuse with phagocytic vesicles in inflammatory processes. The contents of these granules include lactoferrin, vitamin B12-binding proteins (transcobalamins I and III), plasminogen activator, collagenase, etc. These granules also play an important part in promoting chemotaxis and antimicrobial activities. The main functions of neutrophils consist of protective responses to microbial infections.
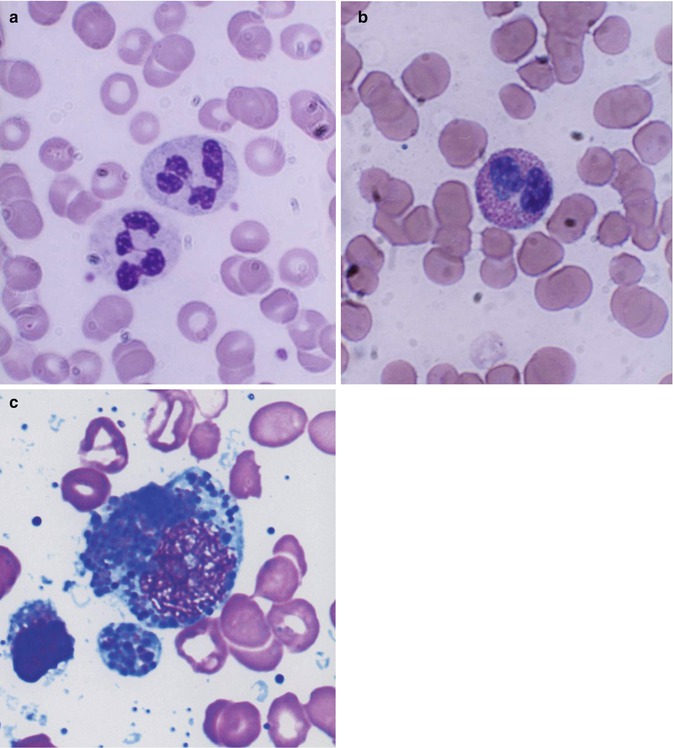
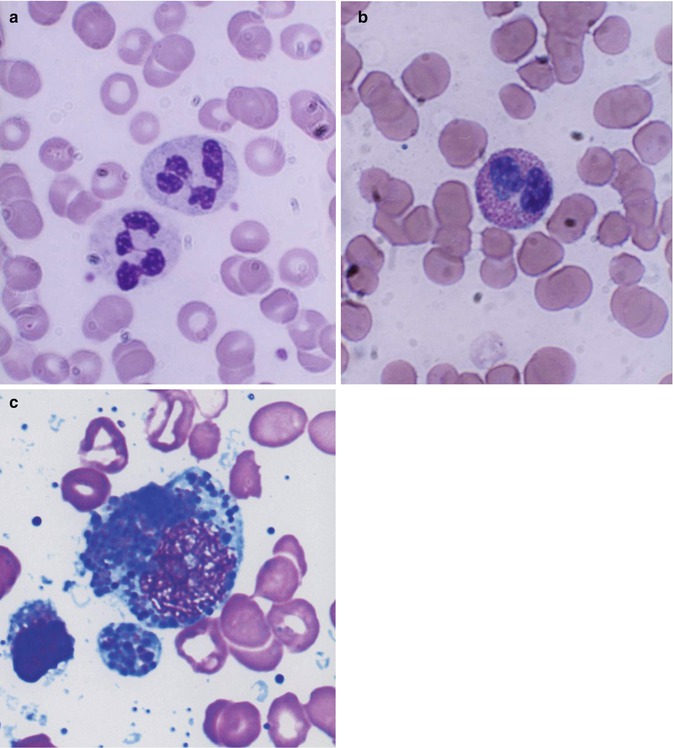
Fig. 13.1
(a) Neutrophilic polymorphonuclear leukocytes showing predominantly secondary or specific granules, which are fine and neither basophilic nor eosinophilic (neutral). Romanowsky stain. (b) Eosinophil, which is a polymorphonuclear leukocyte containing large eosinophilic prominent granules in the cytoplasm. Romanowsky stain. (c) Basophils, which are granulocytic leukocytes containing dark-colored (basophilic) granules in the cytoplasm. Romanowsky stain
Eosinophils participate in allergic reactions, in defense against parasitic infections, and removal of antigen-antibody complexes.
Basophils are round and have irregular short surface projections and many large dark-staining granules (Fig. 13.1c). Basophils are only occasionally seen in normal peripheral blood. The dark cytoplasmic granules of basophils contain heparin, histamine, and minor quantities of other biogenic amines. These cells have receptors for IgE attachment.
Monocytes belong to the mononuclear phagocyte system which comprises monocytes, macrophages, and their precursors. Monocytes (Fig. 13.2) are released from the bone marrow into the blood and after a variable, yet short stay of 20–40 h migrate to different tissues either randomly or in response to chemotactic stimuli. In the tissues, these cells transform into macrophages after activation by microorganisms or other foreign particles.
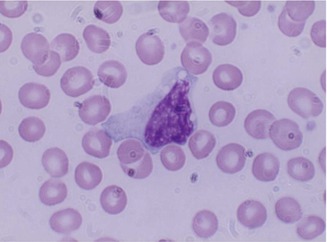
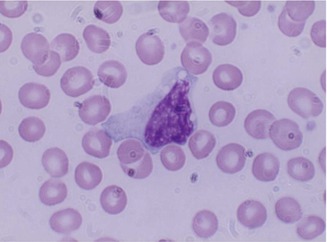
Fig. 13.2
Monocyte; generally larger than neutrophils and have delicate fine nuclear chromatin, indistinct nucleoli, and thin membrane outline. The cytoplasm is abundant with irregular outline, light blue color, and a ground glass appearance
Macrophages represent the tissue phase of the monocytes and are believed to arise by differentiation and transformation of emigrated blood monocytes in the tissues. Macrophages are large cells and irregular in shape (Fig. 13.3) with active amoeboid mobility of the cytoplasm which may contain many vacuoles.
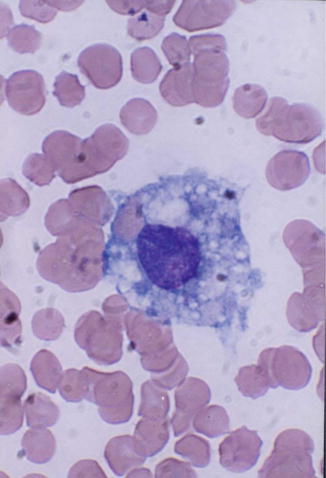
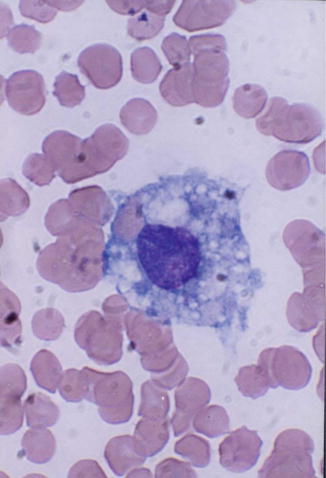
Fig. 13.3
Macrophage; a large mononuclear cells (15–80 μm in diameter) with irregular cytoplasmic membrane outline suggesting amoeboid mobility, finely stippled nuclear chromatin, and spongy vesicular nucleus
Lymphocytes are formed from lymphoid stem cells in the primary lymphoid organs (thymus and bone marrow in postnatal life and yolk sac, liver, and spleen in prenatal life) independent of any antigenic stimulation, but under the influence of several nonspecific cytokines. They are also formed in secondary or reactive lymphopoietic sites such as lymph nodes, spleen, lymphoid follicles of the alimentary and respiratory tracts, and other diffuse lymphoid tissues in response to antigenic challenges.
Lymphocytes (immunocytes) are functionally heterogeneous. The different subpopulations of immunologically competent lymphocytes have evolved in order to meet the requirements of diverse immune functions such as antigen recognition (self-/non-self-discrimination), clonal selection for antibody production, cell-mediated immune functions, immunological memory, and self-regulation. Broadly, B- and T-lymphocytes (Fig. 13.4) evolved to subserve these diverse immunological and biological activities. The various subpopulations of lymphocytes are identified and categorized by the presence of specific cell surface receptors (CD; cluster of differentiation antigens), which are detected by fluorescence-tagged specific monoclonal antibodies in a flow cytometer. B-lymphocytes are capable of synthesizing immunoglobulin (Ig) molecules. The B-lymphocytes are believed to arise from the bone marrow stem cells. T-lymphocytes also originate in the bone marrow stem cells; the prothymocytic T-lymphocytes acquire CD7 antigen on their cell surface and then emigrate to the thymus, where epithelial and dendritic cells provide a microenvironment for their further induction and maturation. A mature T-lymphocyte often contains a dense, but uneven prominent nuclear chromatin structure with one or more intranuclear indentation (Fig. 13.4b). The mature helper T-lymphocytes express CD4 and suppressor cells express CD8; other subpopulations of T-lymphocytes develop many other CD antigens.
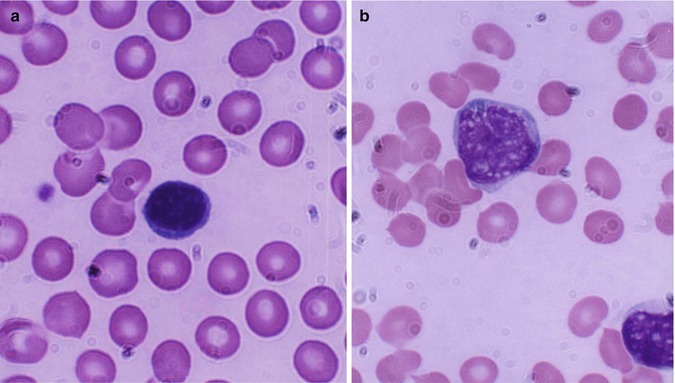
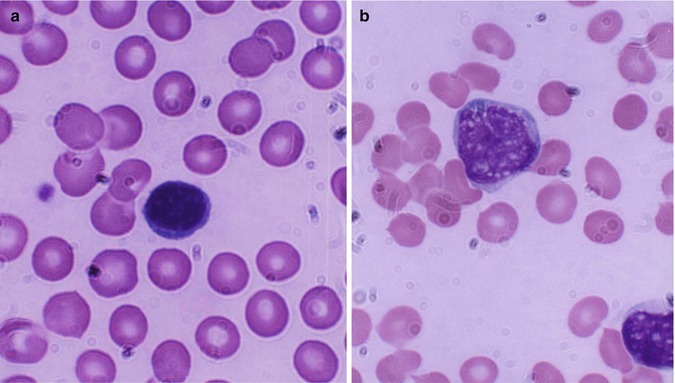
Fig. 13.4
(a) Shows a B-lymphocyte which is a relatively small round mononuclear cell (8–10 μm in diameter), has a nucleus occupying almost the whole of the cell usually with no nuclei, and has scanty cytoplasm with a bluish or moderately basophilic narrow rim of cytoplasm. (b) Shows a T-lymphocyte, which contains dense, but uneven prominent chromatin structure with a shallow intranuclear indentation
Plasma cells are a progeny of B-lymphocytes, actively synthesize immunoglobulins under appropriate antigenic stimuli during which they undergo morphological transformation. They can be morphologically distinguished from lymphocytes by their distinctive features. These cells have a well-defined perinuclear halo (clear zone) (Fig. 13.5) that contains the Golgi apparatus (Fig. 13.5b).
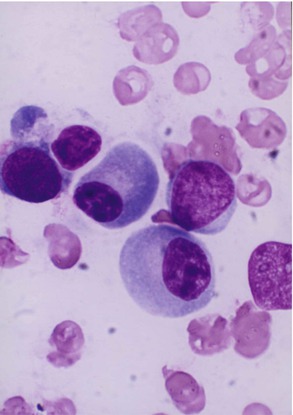
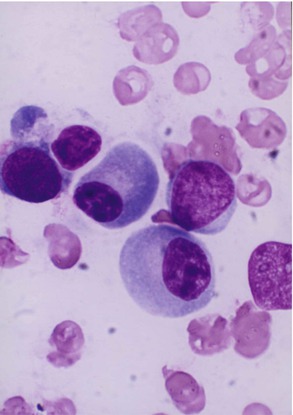
Fig. 13.5
Light microscopic morphology of a plasma cell in Romanowsky stain. The mature plasma cell shows a strongly basophilic cytoplasm and an eccentrically placed nucleus showing aggregated nuclear chromatin, suggesting a “cartwheel” appearance
13.3 The Bone Marrow
The bone marrow is the principal hematopoietic tissue in the adult human being and contains the great majority of the hematopoietic stem cells and the hematopoietic inductive microenvironment that induces differentiation of the stem cells into each blood cell type, characteristic of the diverse cell lineages such as myeloid (granulocytic, monocytic, and erythroid cell) and lymphocytic series of cells. Considerable changes are known to occur in the site and nature of hematopoiesis at different stages of development from the embryo to the adult.
In the embryo, blood cell formation starts in the yolk sac mesoderm as primitive “blood islands” comprising a cluster of specialized mesodermal cells. By the 10th week of prenatal life, a second set of hematopoietic cells develop predominantly in the liver and the spleen from the stem cells that have presumably migrated from the yolk sac mesoderm and then constitute the definitive line of hematopoietic cells (predominantly erythropoietic cells). At this point the fetal liver becomes the main site of blood formation, where erythropoiesis continues and granulopoiesis begins to appear (Fig. 13.6). The spleen is also an important organ for prenatal hematopoiesis, although it makes a much smaller contribution than the liver. During the first 2–3 postnatal years, the bone marrow shows very active hematopoiesis in all bones in the body. During childhood, there is a gradual replacement of active, hematopoietic (red) marrow by relatively inactive, fatty (yellow) marrow. This change starts in the diaphyses of long bones and extends toward the epiphyses (Fig. 13.7). In early adulthood, active hematopoietic marrow is confined to the epiphyses of the long bones and all areas of the flat bones (axial skeleton) such as the sternum, ribs, cranium, vertebrae, and pelvis. However, the distribution of the active bone marrow varies among bones and with age. The femur, for example, will show red marrow in the proximal part till adulthood. Bone marrow in the knee region is variable while none is present in the tibia in adulthood. Even in these “active” hematopoietic tissues, fat cells constitute approximately half of the total marrow tissues (Fig. 13.8). Since half of the red marrow is fatty, 75 % of the total bone marrow in the adult human is virtually adipose tissue [1]. The hematopoietic cells in the red marrow gradually recede with advancing age from about 60 % in the first decade to about 30 % in the eighth decade of life [2]. When there is an increased demand for blood cell formation in response to a hematological stress (e.g., hemolytic anemias), there is an expansion of active hematopoietic tissues to the areas containing predominantly fatty, yellow marrow and sometimes to the liver and the spleen causing “extramedullary hematopoiesis.” The hematopoietic cells in the extramedullary hematopoietic foci develop from the stem cells which migrate to those sites from the bone marrow via the circulating blood [3].
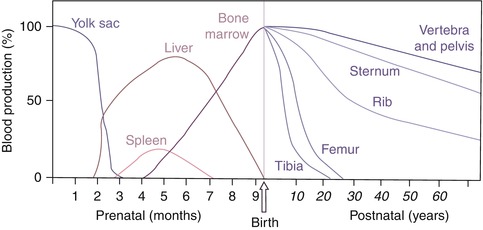
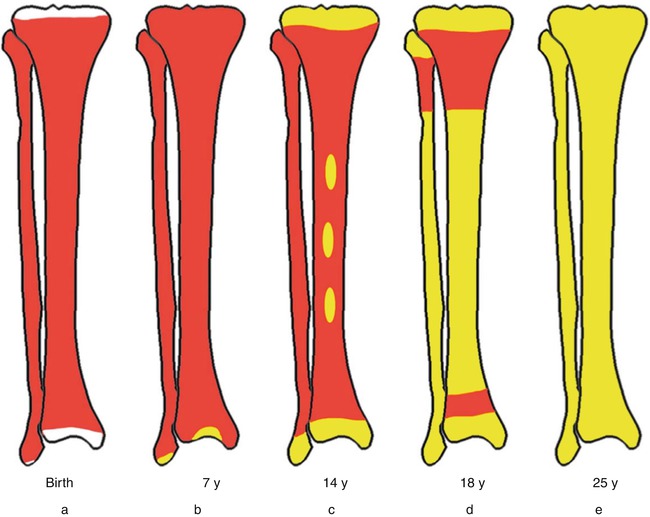
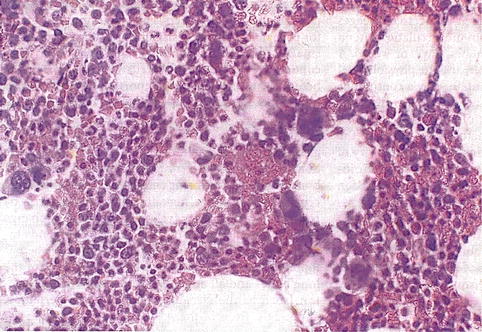
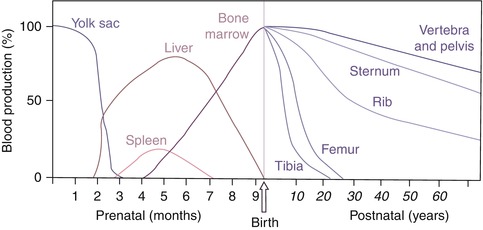
Fig. 13.6
The sites of pre- and postnatal hematopoiesis
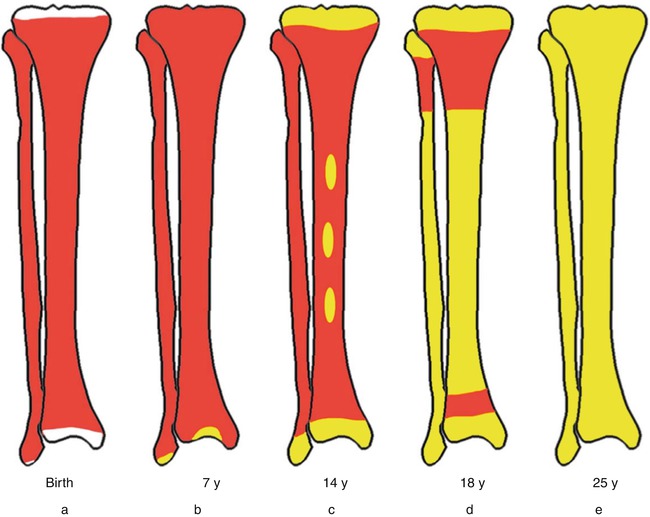
Fig. 13.7
Bone marrow distribution in long bones of the lower extremity illustrating changes during development over the years until the adult pattern is reached by about 25 years of age. a birth, b 7-year-old, c 14-year-old, d 18-year-old, e 25-year-old
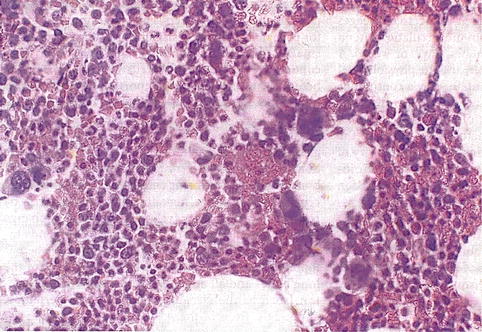
Fig. 13.8
Histological section of a bone marrow biopsy from an adult human being. At least 50 % of the marrow tissue shows fat cells and approximately 50 % hematopoietic cells. H&E
Bone marrow weighs approximately 3,000 g in normal adult men and 2,600 g in women. There is a dual blood supply to the bone marrow consisting of a periosteal capillary network and a nutrient artery that penetrates the bony shaft and divides into multiple branches in the marrow tissue (see Chap. 5 Fig. 5.1). The blood flow through the bone marrow has been estimated to be about 10 ml/min/100 cm2 in normal volunteers, as assessed with positron emission tomography using a 15O-labeled CO2 steady-state technique.
13.4 Erythropoiesis
The formation of mature red blood cells in the bone marrow occurs in different stages starting with the first stem cell progeny committed to erythroid differentiation and ending with the release of red cells into the circulation. The whole mass of erythroid tissue is conceived as a functional unit and is often referred to as the erythron comprising the mature red blood cells, the morphologically recognizable erythroid precursor cells, and their functionally defined (as erythroid colony-forming units) progenitors in the bone marrow (Fig. 13.9). The earliest recognizable erythroid precursor cell in the bone marrow is the proerythroblast (pronormoblast in the normal bone marrow) which is a large cell with dark-blue cytoplasm, a central nucleus, and prominent nuclei. This cell undergoes several divisions (usually four) and progressive maturation to give rise to the basophilic, the polychromatic, and the orthochromatic normoblasts, respectively. As these cells mature, they become smaller, the nuclear chromatin becomes more condensed, and the cytoplasm appears increasingly more hemoglobinized. The nucleus is finally extruded from the normoblast at the orthochromatic stage and a reticulocyte is formed, which contains some ribosomal RNA and can still synthesize hemoglobin. The reticulocytes spend about 1–2 days in the bone marrow before they are released into the circulation, where they spend another 1–2 days mainly in the spleen to mature into red cells. A mature red cell is devoid of RNA and can no longer synthesize hemoglobin.
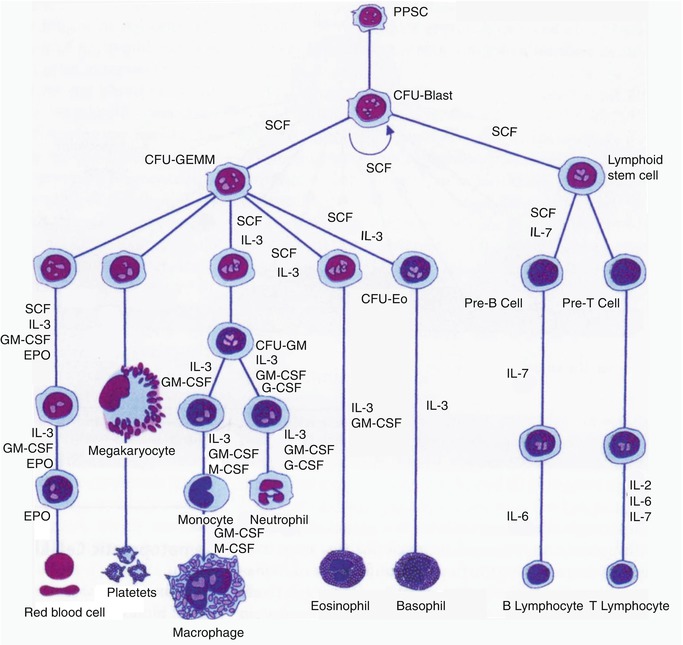
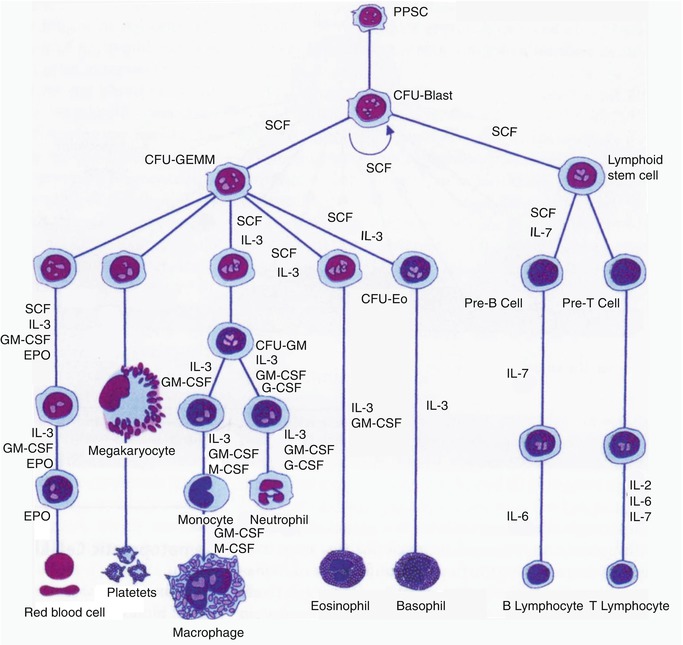
Fig. 13.9
The sites of pre- and postnatal hematopoiesis
Normal human peripheral blood contains about 1–2 % reticulocytes and no normoblasts. Under physiological conditions, erythropoiesis is a well-balanced process in which the rate of red cell production is regulated so as to maintain a steady state and a relatively constant red cell mass. The glycoprotein hormone erythropoietin is the major humoral regulator of erythropoiesis. Erythropoietin has been established as the major regulatory growth factor for erythropoiesis and is known to act on the committed erythroid stem cell, CFU-E, as well as on the other erythroid precursor cells, whereas the early stages of erythropoiesis up to the stage of BFU-E (burst-forming unit-erythroid) are independent of erythropoietin or are minimally influenced by this hormone. Hemoglobin synthesis continues throughout all stages of maturation of erythroblasts and also persists at a very low rate in the reticulocytes after the extrusion of the nucleus.
The process of differentiation of committed erythroid cell, the colony-forming unit-erythroid (CFU-E) into the various stages of erythroid precursors (i.e., pronormoblasts and basophilic, polychromatic, orthochromatic normoblasts, reticulocytes), is associated with the activation of genes for hemoglobin synthesis. Thus, this process involves genes for at least three different biochemical pathways corresponding to the three essential components of the hemoglobin molecule—globin chains, protoporphyrin, and iron.
13.5 Iron Metabolism and Erythropoiesis
Iron metabolism is intimately related to hemoglobin synthesis and thus to erythropoiesis. Iron is the most abundant mineral micronutrient present in the human body. Although iron is one of the most common elements in the earth’s crust, iron deficiency anemia is the most common type of anemia all over the world. The total body iron content in normal adult men is approximately 50 mg/kg body wt., and in women, this is about 35 mg/kg body wt. Two thirds of the total body iron exists as hemoglobin in the erythrocytes. Plasma iron is derived mainly from the reticuloendothelial (RE) cells and the macrophages which acquire iron by engulfing senescent and effete erythrocytes at the end of their life span (erythrophagocytosis). The iron so obtained as a degradation product of hemoglobin by the RE cells generally becomes deposited as ferritin and hemosiderin (siderotic granules). Although monocytes lack transferrin receptors, these cells express these receptors, when they are transformed into macrophages. Only a small proportion of plasma iron comes from dietary sources after their absorption through the duodenum and jejunum. The plasma iron pool is the most important source of iron supply to the various body cells. Iron in the blood plasma is bound almost exclusively to the specific binding protein, transferrin, a globulin which is synthesized in the liver and has a half-life of 8–10 days. Transferrin is the exclusive transport protein for iron delivering it to the cells of the erythroid series and to a smaller extent to other cells. Each molecule of transferrin can bind two atoms of iron and is normally one third saturated with iron (i.e., transferrin saturation 33 %). Normal plasma contains 2.0–4.0 g transferrin per liter. In an average normal subject, the plasma iron concentration is approximately 18 μmol/l (100 μg/dl) and total iron-binding capacity (TIBC) is approximately 56 μmol/l (300 μg/dl). The total transferrin-bound iron in the plasma amounts to approximately 4 mg; this cycles seven times each day to provide about 28 mg of transferrin-bound iron to be reutilized over and over again for hemoglobin synthesis and thus complete the internal iron cycle. Transferrin delivers iron to the red cell precursors and to a smaller extent to other cells by binding to a specific cell surface receptor (transferrin receptor, CD71). The rate of iron uptake by the erythroid cells is related to the number of transferrin receptors on their surface. The mature erythrocytes have very few or no transferrin receptor on their surface and, therefore, cannot take up iron from the plasma. The erythroid precursor cells appear to shed their receptors gradually as they mature.
The transferrin molecules with iron bound to them form a complex with the transferrin receptors on the cell surface and are then interiorized by endocytosis into vesicles. Normally, 80–90 % of the iron that enters the cell is taken up by the mitochondria and is incorporated into heme. Most of the remaining iron becomes bound to ferritin and remains in storage as siderotic granules.
13.6 Ferrokinetics
Iron-turnover studies with intravenously injected radio-iron (59Fe) provide the best obtainable indications of the movement of iron in the metabolic cycle, of the degree of total erythropoietic activity, and of the intensity of effective and ineffective erythropoiesis. However, injected iron is also taken up to some extent by the RE cells in the liver, spleen, and bone marrow, where it may be deposited as storage iron in the form of ferritin and hemosiderin, and also by the circulating reticulocytes. Through plotting measurements on graphs and calculations using several formulas. Ferrokinetic data yield several types of semiquantitative and quantitative information:
1.
Plasma iron clearance (i.e., the clearance of injected radio-iron from the plasma)
2.
Plasma iron turnover or plasma iron transport rate (PIT)
3.
Iron utilization by newly formed red blood cells (RCU)
4.
Surface counting to measure the uptake and turnover of iron by various structures and organs (sacrum, liver, and spleen)
5.
Erythrocyte (red blood cell) iron turnover (EIT)
In these studies, approximately 5–10 ml of plasma is obtained aseptically from freshly collected heparinized blood of the patient. Radioactive iron (59Fe) in the form of ferric chloride is added to the plasma which is then incubated at room temperature for about 15 min. The radio-iron becomes bound to the plasma transferrin, which is then injected intravenously into the patient. A 2-ml sample of blood is collected from the patient at 5 min, and another four to five samples are collected into heparin or EDTA at intervals of 15 min for 1–2 h after the injection of radio-iron, then one sample daily for 10–15 days thereafter. The 59Fe radioactivity in the plasma and red cells of these samples is measured, and the plasma iron concentration and volume of packed red cells are determined.
13.7 Life Spans of Red Blood Cells
The mature red blood cells in the human are highly specialized cells with a relatively simple architecture consisting of a plasma membrane which encloses a solution of proteins, enzymes, and electrolytes. More than 90 % of the intracellular protein consists of the unique oxygen-carrying chromoprotein, hemoglobin; the remainder contains numerous enzymes including those responsible for anaerobic glycolysis (Embden-Meyerhof pathway) and also those responsible for the hexose monophosphate (HMP) shunt pathway.
Measurement of red cell life span can provide useful data in those cases of anemia in which hemolysis remains a possibility but cannot be clearly revealed by other laboratory investigations. This is performed by labeling autologous red cells of patients in vitro with radioactive chromium (51Cr), hexavalent sodium chromate (Na2 51Cr4). The population of red cells of all ages is randomly labeled with 51Cr-labeled sodium chromate (Na2Cr4), which passes through the surface membrane of the red cells and becomes reduced to a trivalent form and binds to proteins. The trivalent chromium is not reutilized nor transferred to other cells in the circulation [32].
Following the injection of 51Cr-labeled red cells, an interval of 10 min (60 min in persons with cardiac failure or splenomegaly) is allowed for the mixing of radiolabeled red cells with the rest of the circulating red cells before a sample of venous blood is collected from a vein other than that used for the initial injection. The 51Cr radioactivity in this sample is taken as the baseline for expressing the radioactivity of samples collected on subsequent days. One part of the labeled cell suspension initially injected into the patient is also retained as a standard for determination of red cell volume or red cell mass, if this was not done earlier. Subsequent samples are collected from the patient daily for 3–4 days and on alternate days for about 10 days; then two specimens are collected per week for another 2 weeks (International Committee for Standardization in Hematology) [34]. Such measurements are performed until the radioactivity has declined to 50 % of the baseline value. 51Cr survival on any day (e.g., day “t”) is given by the following formula:
