(1)
Department of Nuclear Medicine, Kuwait University, Safat, Kuwait
Abstract
A disease is defined in terms of the failure of a normal physiological or biochemical process. Nuclear medicine utilizes these processes. Its diagnostic procedures measure (a) regional blood flow, transport, and cellular localization of various molecules; (b) metabolism and bioenergetics of tissues; (c) physiological function of organs; and (d) intracellular and intercellular communication.
3.1 Radiopharmaceuticals
A disease is defined in terms of the failure of a normal physiological or biochemical process. Nuclear medicine utilizes these processes. Its diagnostic procedures measure (a) regional blood flow, transport, and cellular localization of various molecules; (b) metabolism and bioenergetics of tissues; (c) physiological function of organs; and (d) intracellular and intercellular communication.
A number of radiopharmaceuticals are used for imaging the function and structure of many organs and tissues. Others are available for the treatment of different malignancies, joint diseases, palliation of pain due to bony metastases and other conditions.
3.2 Mechanism(s) of Radiopharmaceutical Localization
The uptake and retention of radiopharmaceuticals by different tissues and organs involve many different mechanisms, summarized in Table 3.1. Many radiopharmaceuticals were designed to take advantage of the pathophysiology in order to increase the specificity of the nuclear medicine imaging techniques. Some radiopharmaceuticals are not specific for a particular disease. Hence, the cellular uptake might include a combination of different mechanisms, as in the case of 67Ga citrate. The unique chemistry of each radiopharmaceutical may determine the manner in which it is transported and retained within a specific tissue or organ. The different mechanisms of localization are discussed below, using specific examples of the more common radiopharmaceuticals.
Table 3.1
Mechanisms of radiopharmaceutical localization
Mechanism | Radiopharmaceutical |
---|---|
1. Isotope dilution | 125I-HSA, 51Cr-RBC, and 99mTc-RBC |
2. Capillary blockade | 99mTc-MAA |
3. Physicochemical adsorption | 99mTc-MDP, HDP |
4. Cellular migration | 111In- and 99mTc-leukocytes, 111In-platelets |
5. Cell sequestration | Heat-denatured 99mTc-RBC |
6. Simple diffusion | |
Diffusion and mitochondrial binding | 133Xe, 81mKr, 99mTc-pertechnegas |
Diffusion and intracellular binding | 99mTc-sestamibi and tetrofosmin |
Diffusion and increased capillary permeability | 99mTc-Ceretec and Neurolite |
7. Facilitated diffusion and transport, protein upregulation | 67Ga-citrate |
8. Active transport | [18F]-FDG, radiolabeled amino acids |
Radioiodide, 99mTcO4 –, 201Tl thallous cation | |
[18F]-FDG, radiolabeled amino acids | |
Na+/K+ ATPase pump | 201Tl thallous cation |
9. Phagocytosis | 99mTc-colloids in RES and lymph nodes |
10. Increased vascular permeability and capillary leakage | 67Ga-citrate, radiolabeled proteins |
11. Cell proliferation | [11C]-thymidine, [124I]-iododeoxyuridine (IudR), 18F-fluorothymidine (FLT) |
12. Metabolic trapping | [18F]-FDG, 99m Tc-pertechnetate |
13. Metabolic substrates | 123I and 131I as sodium iodide, 123I-fatty acids |
14. Tissue hypoxia and acidic pH | [18F]fluoromisonidazole, 67Ga-citrate |
15. Specific receptor binding | |
Somatostatin receptors | OctreoScan, NeoTect |
VIP receptors | 123I-VIP |
Transferrin receptors | 67Ga-citrate |
Estrogen receptors | 16α-[18F]fluoro-17β-estradiol (FES) |
Dopamine D2 receptors | [123I]-IBZM, 99mTc-TRODAT |
LDL receptors | 131I-6β-iodomethyl-19-norcholesterol (NP-59) |
Presynaptic adrenergic reuptake | [131I or 123I]-MIBG |
16. Specific binding to tumor antigens | |
PSMA | ProstaScint |
CEA | CEA-Scan |
TAG-72 | OncoScint |
Cell surface 40-kd glycoprotein | Verluma |
CD22 | Bexaar |
CD15 | 99mTc-fanolesomab |
Antimyosin | 111In-antimyosin |
3.2.1 Isotope Dilution
The dilution principle is based on the concept of “diluting” a radiotracer (or tracer) of known activity (or mass) in an unknown volume. By measuring the degree to which the radiotracer was diluted by the unknown volume, one can determine the total volume (or mass) of the unknown volume. The dilution principle is currently used for a quantitative determination of RBC volume (mass), plasma volume, and total blood volume.
It is very important that the radiotracer remains only in the blood volume to be measured. Nondiffusible intravascular agents such as 51Cr-RBCs are used to measure RBC mass, while 125I-HSA is used to measure plasma volume. The use of 99mTc-RBCs for the measurement of cardiac ejection fraction and gastrointestinal bleeding studies is another application of the dilution principle.
3.2.2 Capillary Blockade
The technique most commonly used to determine the perfusion to an organ depends on trapping the radiolabeled particles (microembolization) in the capillary bed of an organ such as lung, heart, or brain. Following intravenous injection, 99mTc-MAA particles are physically trapped in the arteriocapillary beds of the lung and block the blood flow to the distal regions. Therefore, the mechanism of localization of particles in the lungs is purely a mechanical process, called capillary blockade.
3.2.3 Physicochemical Adsorption
The 99mTc-phosphonates accumulate in hydroxyapatite crystal (containing Ca2+ and phosphate ions) matrix or in the amorphous (noncrystalline) calcium phosphate. The uptake mechanism of sodium fluoride [18F] resembles that of 99mTc-labeled diphosphonates. However, it has faster blood clearance and a twofold higher uptake in bone.
The principal uptake mechanism of the radiotracer appears to be simply “physicochemical adsorption.” However, the exact mechanisms involved in the extraction of the radiotracer from the blood through the endothelial cells, extracellular fluid, and finally to hydroxyapatite crystal are not known. Primary bone tumors such as osteogenic sarcomas avidly accumulate bone agents because of the production of bone matrix in extraosseous tissue. Metastatic deposits that produce a vigorous osteoblastic response will appear as hot spots in a bone scan, while the lesions that generate osteolytic reactions may not accumulate the bone agent [1].
The localization of bone-seeking radiotracers in increased amounts at the tumor-bone interface provides the basis for the use of radionuclides in the treatment of bone pain. Several radiopharmaceuticals (see Chap. 14) are used for relief of bone pain in patients with confirmed osteoblastic bone lesions.
3.2.4 Cellular Migration and Sequestration
111In-oxine- or 99mTc-HMPAO-labeled autologous mixed leukocytes (predominantly neutrophilic polymorphonuclear leukocytes, PMNs) are routinely used to image various inflammatory diseases and infectious processes. The inflammatory reaction is a well-described sequence of events in response to an infection (see Chap. 4).
Following intravenous administration of radiolabeled leukocytes, the labeled cells migrate to the site of infection, similar to the circulating leukocytes, because they are attracted by the immediately generated chemotactic factors, such as complement subcomponents.
In a similar manner, 111In-platelet localization at the site of active thrombus formation also involves simple cellular migration, since platelets play a major role in thrombus formation. Accessory splenic tissue can develop after splenectomy. Heat-damaged 99mTc-RBCs are more specific for the detection of accessory splenic tissue. Following intravenous administration, the spleen sequesters the heat-damaged RBCs in the same way that old and damaged circulating RBCs are normally removed.
3.2.5 Membrane Transport
Transport through the lipid bilayer or through the transport proteins may involve simple diffusion, passive transport (facilitated diffusion), or active transport mechanisms. Certain macromolecules may also be transported by vesicle formation, involving either endocytosis or exocytosis mechanisms.
3.2.5.1 Simple Diffusion
Many radiopharmaceuticals localize in target organs involve a simple diffusion process. The direction of movement of the radiotracers by diffusion is always from a higher to a lower concentration, and the initial rate of diffusion is directly proportional to the concentration of the radiotracer. A net movement of molecules from one side to another will continue until the concentration on each side is at chemical equilibrium. Following the administration of gases used for ventilation studies, such as 133Xe, 127Xe, and 81mKr, through inhalation, gases are distributed within the lung air spaces by diffusion, proportional to ventilation. The gases pass from the lungs into the pulmonary venous circulation and are released through the lungs by the mechanism of alveolar capillary diffusion. Similarly, distribution of 99mTc-Technegas within the lung also involves diffusion. On the other hand, the irregular distribution of 99mTc-DTPA aerosol preparation within the lung is due mostly to gravity sedimentation depending on particle size.
3.2.5.1.1 Simple Diffusion and Intracellular Metabolism/Binding
The blood-brain barrier (BBB) plays an important role in the mechanism of localization of many radiopharmaceuticals in the brain. The endothelial cells of the cerebral vessels form a continuous layer without gap junctions, preventing diffusion of water-soluble molecules.
In certain pathological conditions, the BBB is disrupted, allowing water-soluble molecules to diffuse from the blood into brain tissue.
Brain perfusion-imaging agents such as 99mTc-HMPAO and 99mTc-ECD are lipophilic radiotracers that cross the BBB via passive diffusion. The extraction of these tracers by the brain tissue is proportional to regional cerebral blood flow (rCBF). The retention of these tracers within the neuronal tissue following diffusion and extraction is assumed to be due to intracellular binding or metabolic degradation to polar metabolites or charged complexes that cannot be washed out of the cell by back diffusion as exemplified by the cellular retention of 99mTc-ECD and 99mTc-pertechnetate in labeled RBC. With 99mTC-ECD the radiotracer freely diffuses into the brain tissue, where it is hydrolyzed by the action of esterase to an acid which is trapped in the brain tissue. For 99mTcO4, the tracer is reduced intracellulary by the circulating stannous ion, and the reduced 99mTc binds to Hgb to form 99mTcHgb that does not diffuse out of the brain tissue.
3.2.5.1.2 Simple Diffusion and Mitochondrial Binding
A number of 99mTc radiopharmaceuticals such as sestamibi and tetrofosmin have been developed for imaging myocardial perfusion. Although 99mTc-sestamibi is cationic, similar to 201Tl+, the transport of this agent through the cell membrane involves only passive diffusion [2].
The myocardial cell uptake of 99mTc-sestamibi is due to intracellular binding of 99mTc-sestamibi associated mainly with the mitochondria. Mitochondrial retention of 99mTc-sestamibi, however, is not organ or tumor specific, but appears to be a mechanism common to most types of tissue. The intracellular levels of Ca2+ in normal cells are significantly low. However, with irreversible ischemia, extracellular calcium enters the cell and is sequestered in the mitochondria, resulting in mitochondrial destruction. The increased calcium concentration in the mitochondria blocks 99mTc-sestamibi binding to the mitochondria.
These 99mTc lipophilic cationic complexes are also used for imaging of parathyroid localization, and tumor imaging and the mechanism of uptake again are associated with the mitochondria.
There are significant differences between sestamibi and tetrofosmin regarding intracellular localization based on in vitro studies. While 90 % of total sestamibi was associated with mitochondria, most of the tetrofosmin accumulated in the cytosolic fraction [3].
The transport of the tracer out of the tumor cell is mediated by P-glycoprotein (Pgp), a 17-kd plasma membrane lipoprotein encoded by the human multidrug resistance (MDR) gene, and accordingly 99mTc sestamibi is useful for imaging Pgp expression.
3.2.5.1.3 Simple Diffusion and Increased Permeability
This mechanism is illustrated with 67Ga-citrate localization in a variety of tumors and inflammatory lesions. Following intravenous administration of carrier-free 67Ga as gallium citrate, 67Ga is bound to the iron transport glycoprotein, transferrin in normal plasma, and is transported to normal tissues and tumor sites predominantly as 67Ga-transferrin complex [4]. The initial entry of 67Ga into tumor tissue involves simple diffusion of the unbound or loosely bound form of 67Ga, whereas its uptake by normal soft tissues is strongly promoted by its binding to transferrin [5]. There is increased transferrin concentration within the interstitial fluid of the tumors. The increased permeability of the tumor cell membrane compared with normal cells also accounts for increased diffusion of non-transferrin-bound gallium species into cells. The accumulation of 67Ga within tumor cells is very much dependent upon the intracellular binding of 67Ga to iron-binding proteins such as lactoferrin and ferritin or other higher-molecular-weight molecules which can chelate gallium with greater affinity, thereby preventing back diffusion of free gallium [6]. 68Ga-citrate is similar to 67Ga-citrate but is a positron-emitting radiopharmaceutical and shares the same uptake pathway. 68Ga is produced by a 68Ge/68Ga generator (t ½ 68 min, β+ 88 %) and is not dependent on a cyclotron.
3.2.5.2 Facilitated Diffusion
3.2.5.2.1 18F-fluorodeoxyglucose (FDG)
All cells use glucose to generate metabolic energy. For brain tissue, glucose is the primary source of energy, but in the heart glucose becomes the primary source of energy for ischemic myocardium. Glucose is transported into the cell across the plasma membrane by facilitated diffusion, mediated by members of the glucose transporter (Glut) protein family (Glut1–6) [7]. Similar to glucose, FDG is also transported into normal and malignant cells by facilitated diffusion.
3.2.5.2.2 Hepatobiliary Agents
Evaluation of hepatocyte function using radiopharmaceuticals that are excreted via biliary secretion is another example of a carrier-mediated transport mechanism. Following intravenous administration, 99mTc-disofenin (Hepatolite) and 99mTc-mebrofenin (Choletec) diffuse through pores in the endothelial lining of the sinusoids and bind to the anionic membrane-bound carriers on the hepatocyte. The hepatic uptake is facilitated by carrier-mediated, non-sodium-dependent, organic anionic pathways similar to that of bilirubin.
Subsequent biliary excretion of the radiotracer is relatively passive following the flow of bile through the biliary tree. The bile may be stored and concentrated temporarily in the gallbladder or excreted directly into the intestine. Since bilirubin is excreted by the same hepatocyte transport system, higher serum bilirubin levels may have a significant effect on the biodistribution and hepatic excretion of radiopharmaceuticals.
3.2.5.3 Active Transport
Active transport involves translocating a solute molecule through a cell membrane against its concentration gradient and requires the expenditure of some form of energy. Active transport is driven by either hydrolysis of ATP to ADP (primary active transporters) or utilization of an electrochemical gradient of Na + or H + (secondary active transporters) across the membrane. If the energy source is inhibited or removed, the transport system will not function.
3.2.5.3.1 Radioiodide and 99mTc-Pertechnetate Anions
Thyroid tissue selectively traps certain anions, such as I–, TcO4–, and ClO4–, by an active transport mechanism using the same pathway; hence, they are the competitive inhibitors of each other. The clinical implication is that iodinated contrast agents or iodine-containing medications may interfere with the accumulation of 99mTcO4 in the thyroid, thereby leading to poor image quality.
However, only iodide is used by the thyroid gland to synthesize thyroid hormones, while the other anions diffuse out of the gland. In addition to thyroid tissue, the salivary glands, stomach, bowel, and genitourinary tract show significant uptake (secretion) of radioiodide and pertechnetate.
3.2.5.3.2 201Thallous Chloride
Since the thallous ion (Tl (OH) 2+) acts as an analog of the K + ion, 201Tl has been used to image myocardial perfusion in order to evaluate the extent of myocardial ischemia and/or infarction. Positron emitter 82Rb—a monocation, like potassium—is also used for imaging myocardial perfusion. The myocardial uptake of thallium and rubidium involves active cation transport mechanisms including both passive diffusion and ATP or energy-dependent pathways [8].
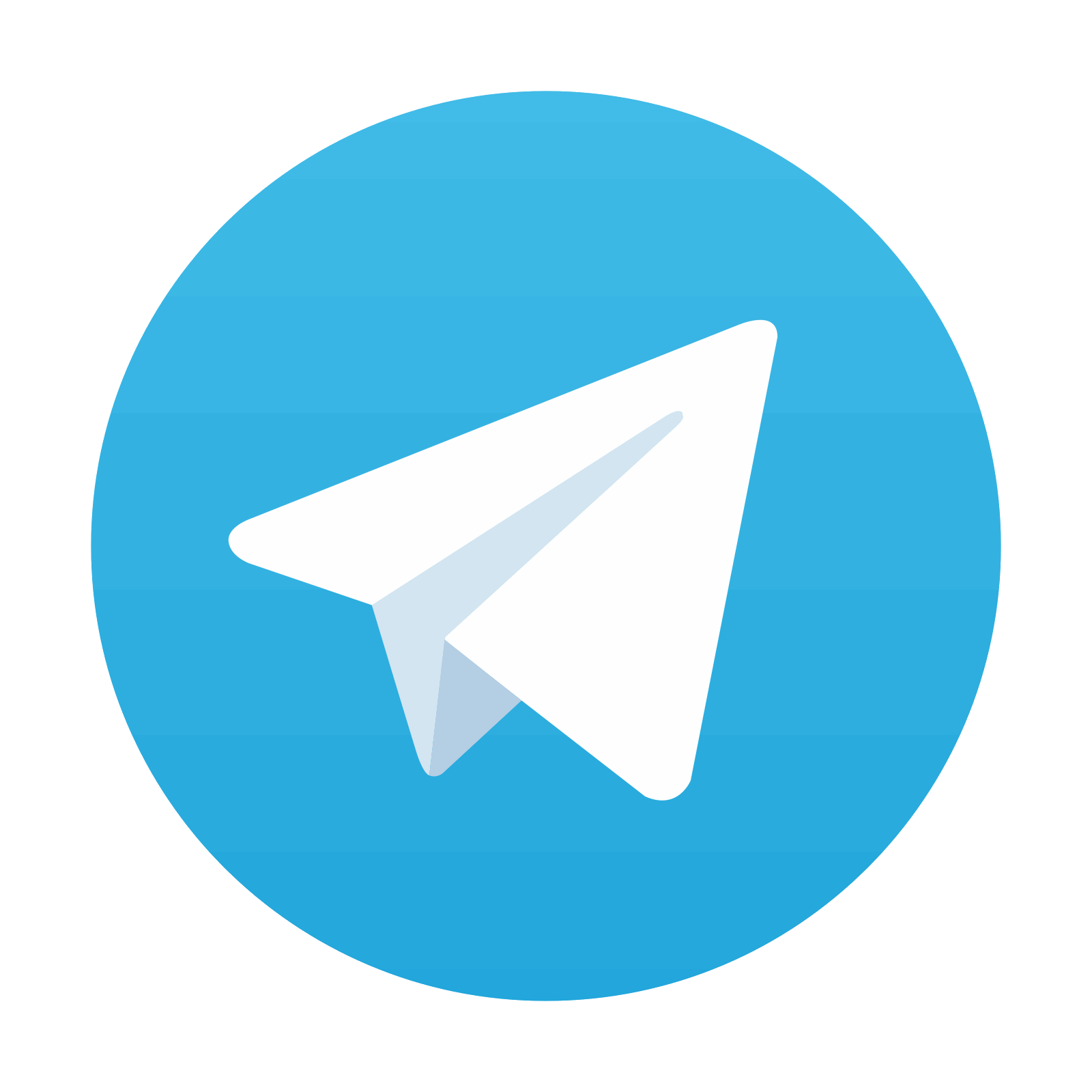
Stay updated, free articles. Join our Telegram channel

Full access? Get Clinical Tree
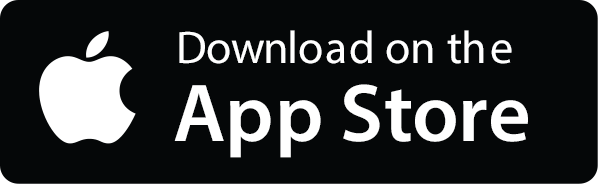
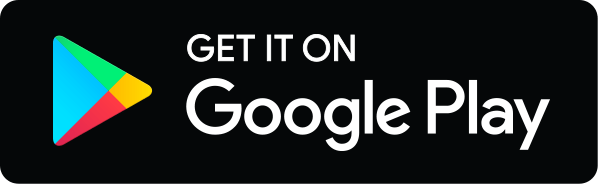
