(1)
Department of Nuclear Medicine, Kuwait University, Safat, Kuwait
11.1.1 Respiratory Airways
11.1.2 Pulmonary Vasculature
11.1.3 Respiratory Function
11.2.2 Deep Venous Thrombosis
11.2.3 Pulmonary Thromboembolism
Abstract
The pulmonary system consists of the lungs, airways, pulmonary and bronchial circulation, and chest wall. The lungs consist of lobes, three in the right (upper, middle, and lower) and two in the left lung (upper and lower). Each lobe is again divided into segments and lobules (Fig. 11.1). The airway system consists of upper airways (nasopharynx and oropharynx) and lower airways (trachea, bronchi, bronchioles, and alveolar ducts) connected by the larynx (Fig. 11.2).
11.1 Anatomical and Physiological Considerations
The pulmonary system consists of the lungs, airways, pulmonary and bronchial circulation, and chest wall. The lungs consist of lobes, three in the right (upper, middle, and lower) and two in the left lung (upper and lower). Each lobe is again divided into segments and lobules. The airway system consists of upper airways (nasopharynx and oropharynx) and lower airways (trachea, bronchi, bronchioles, and alveolar ducts) connected by the larynx (Fig. 11.1).
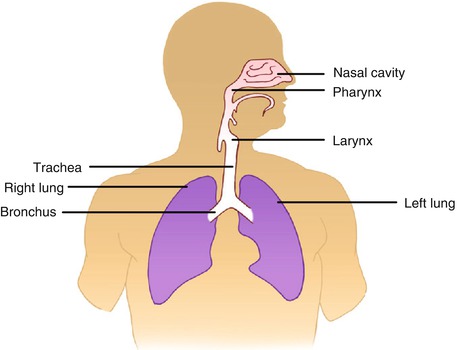
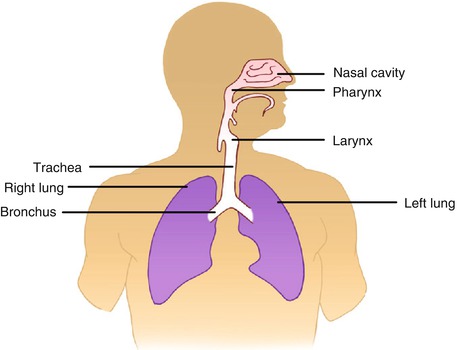
Fig. 11.1
Simple diagram of the upper and lower airways
11.1.1 Respiratory Airways
The upper airways are lined by a ciliated mucosa, richly supplied with blood, which warm and humidify the inspired air and get rid of foreign particles. The air normally flows by the way of the nose, nasopharynx, and oropharynx to the lower airways. When the nose is obstructed or additional flow of air is needed, as during exercise, air flows via the mouth and oropharynx to the lower airways. Foreign particle removal and humidification are not efficient with mouth breathing as compared with the usual breathing through the nose.
The lower airways are formed of a conducting system and a gas exchange system (Fig. 11.2). The trachea divides into two main bronchi at the carina, and each bronchus enters the corresponding lung at the hilum along with the pulmonary blood vessels and lymphatic channels. The trachea measures up to 25 cm in length and 2.5 cm in diameter. The right main bronchus extends to the right lung more vertically than the left bronchus to the left lung. This explains the more frequent aspiration of foreign material in the right side. At the hila the bronchi divide into lobar bronchi, then segmental and subsegmental bronchi, and then into smaller bronchioles, and at the 16th division, the tracheobronchial tree ends in the tiny terminal bronchioles which form the ends of the conducting airways and are followed by the gas exchange airways. The lung segments are individual units with their bronchovascular supply; hence, they can be individually resected. The airways responsible for conducting air from outside the body into the lungs are lined by ciliated mucous membranes. The cilia, which are hairlike projections, act as sweepers to prevent dust and foreign particles from passing distally into the lungs. Damage to the respiratory epithelium and its cilia allows bacteria and viruses to proliferate and induce infection.
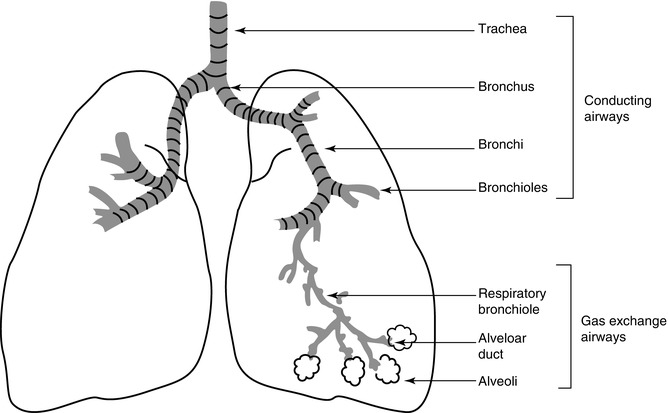
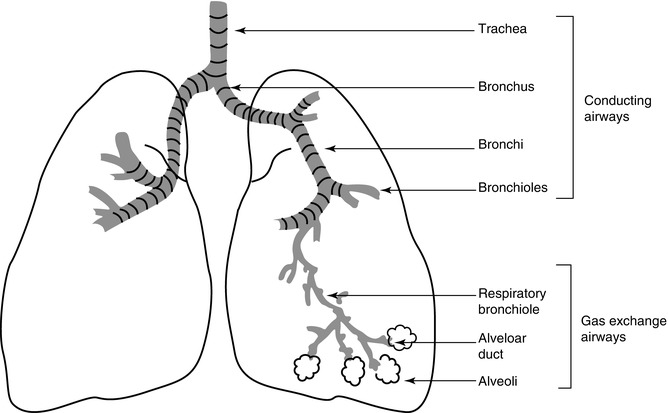
Fig. 11.2
The trachea, bronchi, and bronchioles form the tracheobronchial tree, so called since it resembles an inverted tree. The conducting system is composed of the trachea, bronchi, and bronchioles up to the 16th division and is lined by ciliated mucosa. The gas exchange system consists of the more distal bronchioles (respiratory) and the alveoli that are lined by nonciliated mucus membrane
The gas exchange airways start where the terminal bronchioles divide further into smaller, respiratory bronchioles which include increasing numbers of alveoli as the division progresses. By the 23rd division, the respiratory bronchioles end in alveolar ducts that lead to alveolar sacs which are made up of numerous alveoli. The alveoli are extremely thin-walled sacs surrounded by capillaries and are the primary site of gas exchange. At birth there are approximately 25 million alveoli; this increases to 300 million in adults. The alveoli are lined by type I alveolar cells that provide structure to the alveolar wall and type II cells that secrete a lipoprotein, the surfactant which coats the alveolar inner surface and aids its expansion during inspiration [1].
The conducting system is composed of the trachea, bronchi, and bronchioles up to the 16th division and is lined by ciliated mucosa. The gas exchange system consists of the more distal bronchioles (respiratory) and the alveoli that are lined by nonciliated mucus membrane.
Ventilation describes the process by which air flows in and out of the gas exchange airways. Ventilation is involuntary most of the time and is controlled by the sympathetic and parasympathetic autonomic nervous systems, which adjust the caliber of the airway via contraction and relaxation of the bronchial smooth muscle and control the depth and rate of ventilation.
The nose and trachea trap most particles of more than 10 μm in diameter, while the cilia of the bronchi and bronchioles pick up particles 2–10 μm in diameter that are deposited in these airways. Smaller particles remain airborne till they are deposited in the alveoli and removed by macrophages. Extremely small particles behave as a gas and are breathed out. This is the basis of scintigraphic ventilation studies using radioactive aerosols and gases. The flow of oxygen through the 99mTc-DTPA reservoir should create small aerosol particles to be airborne and deposited distally in the alveoli. Larger particles are deposited in the more proximal airways and influence the quality of ventilation studies. This also explains the longer biological clearance of aerosols compared with radioactive gases, which are breathed out without deposition.
11.1.2 Pulmonary Vasculature
The lung is supplied by two different blood circulations. The pulmonary circulation is a low-pressure, low-resistance system through which oxygen enters and carbon dioxide is removed. The bronchial circulation is a part of the high-pressure systemic circulation that supplies oxygenated blood to the lung tissue itself.
The pulmonary circulation contains the vast majority of blood present in the lung, and since it has lower pressure than systemic circulation, its vessels have a thinner muscle layer. The mean pulmonary artery pressure is 18 mmHg, compared with 90 mmHg for the aorta. The gas exchange airways are served by this pulmonary circulation, which is considered a separate division of the circulatory system. The pulmonary circulation is carried through the pulmonary artery, which branches out to two main pulmonary arteries, one to each lung, entering at the hilum. It then divides progressively into smaller branches, following the branches of the bronchial tree to the smallest, precapillary arterioles, which divide to form a capillary network surrounding the alveoli. The membrane that surrounds the alveoli and contains the capillaries is called the alveolocapillary membrane [2].
The precapillary arterioles are approximately 35 μm in diameter and number approximately 300 million in adults. The capillaries, 7–10 μm in diameter, number 300 billion in adults. The more proximal terminal arterioles have a diameter of approximately 100 μm. This basic anatomical fact is important in determining the size of particles injected for perfusion studies; they should be less than 100 μm to prevent blocking of the terminal arterioles [3].
Although the pulmonary circulation is innervated by the autonomic nervous system, vasodilation and vasoconstriction are controlled mainly by local and humoral factors, particularly arterial oxygenation and acid-base balance. Vasoconstriction of the pulmonary arterial system occurs secondary to alveolar hypoxia and acidemia and by the presence of inflammatory mediators such as histamine, bradykinin, serotonin, and prostaglandin.
The bronchial circulation, on the other hand, carries approximately 5 % of the blood coming to the lungs and is part of the systemic circulation. In contrast to the pulmonary circulation, it does not participate in gas exchange. It supplies the tracheobronchial tree, large pulmonary vessels, and other structures of the lungs, including the pleurae, with blood.
11.1.3 Respiratory Function
The major function of the respiratory system is to oxygenate the blood and remove waste products of the body in the form of carbon dioxide. Oxygen in the inhaled air diffuses from the alveoli into the surrounding blood in the capillaries, where it attaches to hemoglobin molecules and red blood cells and is carried to the various tissues of the body. Carbon dioxide, on the other hand, as a waste product of cellular metabolism, diffuses in the opposite direction, from the blood in capillaries into the alveoli, and is removed from the body during expiration.
The respiration is controlled by the respiratory center in the medulla at the base of the brain. The respiratory center in the brain stem sends impulses to respiratory muscles to contract and relax. The respiratory center also receives impulses from two main types of peripheral receptors: neuro- and chemoreceptors. Neuroreceptors (lung receptors) monitor the mechanical aspects of ventilation such as the need to expel unwanted substances and expansion of the lungs. The chemoreceptors in the brain circulatory system monitor the pH status of the cerebrospinal fluid and arterial oxygen content (PO2) to regulate ventilation accordingly.
Any change in the carbon dioxide in the blood will affect the rate and depth of respiration. A slight increase in carbon dioxide concentration in the blood increases the rate and depth of respiration, such as when the individual exercises, since the accumulated waste gas must be removed from the body.
This increase in respiratory rate and depth is secondary to the stimulation of the muscles of respiration, which include the diaphragm and the intercostal muscles, by the respiratory center. Contraction of these muscles causes the volume of the chest cavity to increase, with a consequent drop in the pressure within the lungs, and forces air to move into the tracheobronchial tree. When these respiratory muscles relax, the volume of the chest cavity decreases, the pressure increases, and the air is pushed out of the lungs. When breathing is difficult, or in patients with obstructive airway disease, special muscles of expiration, abdominal and internal intercostal muscles, may be additionally needed.
11.1.4 Distribution of Ventilation and Perfusion
Normally, the lower zones of the lungs are better perfused and ventilated because of the effect of gravity. This gradient is more pronounced in perfusion than in ventilation (Fig. 11.3). This physiological fact will usually cause the perfusion to appear less than the ventilation in the lung apices on scintigraphy.
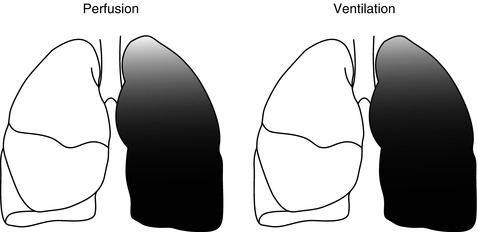
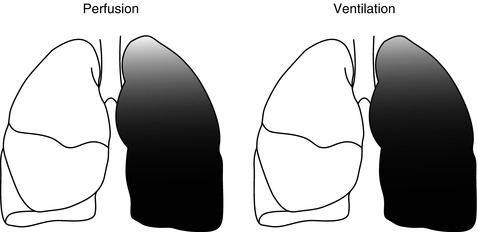
Fig. 11.3
The gradient pattern in perfusion and ventilation of the lungs (From Elgazzar et al. [31] with permission)
11.2 Pulmonary Embolic Disease
Pulmonary embolism is potentially fatal and the most common pathological condition involving the lungs of hospitalized patients. The majority of fatal emboli are not recognized or suspected prior to death.
11.2.1 Pathogenesis and Risk Factors
The vast majority of pulmonary emboli are thromboemboli originating from deep veins. Fat, air, or tumor emboli are rare [5]. Fat emboli are reported with long bone fractures and liposuction, while air emboli occur with cardiac- and neurosurgeries. Renal cell carcinoma with invasion reaching inferior vena cava is a clinical setting that may lead to tumor emboli. Data indicate that 90 % of pulmonary thromboemboli originate from the lower extremities and pelvis. The remainder come from thrombi that occur in the right side of the heart or in bronchial or cervical veins. Embolization and symptomatology are proportional to how proximal is the vein that contains the thrombus. The vast majority of pulmonary thromboemboli originating from thrombi of the lower extremities come more frequently from the thigh and pelvis (75 %) than from smaller veins of the calf and feet [6, 7]. Septic embolus refers to an infected thromboembolus which occurs either on site or secondary to detachment of an infected vein thrombus of the lower extremities. The risk of pulmonary embolus is also directly related to the presence of a residual clot at the site of a venous thrombus [8].
11.2.2 Deep Venous Thrombosis
Pulmonary emboli arise from sites of deep venous thrombosis. Therefore, those at risk for deep venous thrombosis are those at risk for pulmonary embolism. The classical risk triad elucidated by Virchow in the nineteenth century includes venous stasis, intimal injury, and alteration in coagulation. Up to 90 % of all patients with thromboembolism have no identifiable coagulopathy. Thus, in most patients, some clinical states associated with venous stasis, intimal injury, or both are the basis for an increased risk of deep venous thrombosis. These clinical states include injury to the pelvis or lower extremities; surgery involving the lower extremities; all surgical procedures requiring prolonged (at least 30 min) general anesthesia; burns, pregnancy, and the postpartum state; previous venous thrombosis with residual obstruction; right ventricular failure of any cause; occupations in which prolonged venous stasis is involved; and any cause of immobility. Other risk factors include obesity, cancer and the use of estrogen-containing medications, neoplasm, and hypercoagulability (Table 11.1).
Table 11.1
Risk factors for deep vein thrombosis and pulmonary thromboembolism
1. Postoperative state especially following operations on the abdomen and pelvis |
2. Trauma, including fractures, particularly of the lower extremities |
3. Neoplasms |
4. Prior history of thromboembolic disease |
5. Venous stasis |
6. Vascular spasm |
7. Intimal injury |
8. Hypercoagulability states |
9. Immobilization |
10. Infection of the area in the immediate vicinity of veins |
11. Heart disease, especially: |
Myocardial infarction |
Atrial fibrillation |
Cardiomyopathy |
Congestive heart failure |
12. Pregnancy |
13. Polycythemia |
14. Hemorrhage |
15. Obesity |
16. Old age |
17. Varicose veins |
18. Certain drugs such as oral contraceptives, estrogens |
19. Following cerebrovascular accidents |
The risk factors should be regarded as cumulative, not independent. These factors allow the establishment of a “risk profile” for a given patient, a profile that conditions the intensity of prophylactic initiatives. Venous thrombi appear to begin either in the vicinity of a venous valve or at the site of intimal injury. Platelet aggregation and release of mediators initiate the sequence. With stasis, there is local accumulation of coagulation factors; the coagulation cascade is activated, and the characteristic red fibrin thrombus develops. Pathologically there will be a platelet nidus from which a large fibrin thrombus extends.
After the formation of the thrombus, one of three events can happen. First, the red thrombus grows explosively and obstructs the vein completely. Second, partial venous obstruction may occur. Blood flow therefore continues over the thrombus surface. Under this circumstance, thrombus growth tends to occur by the progressive layering of platelets and fibrin on the clot surface, pathologically seen as the lines of Zahn. Third, probably the most common scenario, a small thrombus is swept away before it reaches an appreciable size. It lodges in the pulmonary vasculature without symptoms.
Unless fibrinolytic resolution is prompt, organization of the thrombus begins within hours of formation and the thrombus is slowly replaced by granulation tissue. This process anchors the thrombus to the venous wall.
The dynamic battle between fibrinolysis and thrombus formation is fought out over a period of 7–10 days, at the end of which time either complete resolution has occurred or an endothelialized residual is present. During this period, a portion or all of the thrombus can detach as an embolus. This risk is highest early, before significant dissolution or organization occur [7].
11.2.3 Pulmonary Thromboembolism
11.2.3.1 Consequences
Pulmonary thromboemboli occur more commonly in the lower lobes because of the preferential blood flow to these regions. This also applies to the right lung because of the straighter course of the pulmonary artery. Immediately after acute embolism, there is a decrease of perfusion distal to the occluded vessel along with a transient decrease of ventilation to the affected segment. The blood flow is diverted to the other portions of the lung, and pulmonary artery pressure may increase, although cardiac output usually remains stable. The resultant tissue ischemia disturbs certain metabolic functions of the lung such as the production of surfactant. Reduction of the surfactant concentration reduces the alveolar surface tension and may cause the atelectasis that often accompanies embolism. If the embolus completely occludes an artery or an arteriole and the collateral bronchial circulation is insufficient to sustain tissue viability, infarction occurs over 24–48 h. Pulmonary infarction with coagulative necrosis results in an area of radiographic opacity that requires an average of 20 days to resolve but occurs in less than 10–15 % of patients with pulmonary embolism. There is significant inflammatory component in pulmonary infarcts which is the basis behind the significant FDG uptake in recent lung infarcts and can cause false-positive interpretation for lung malignancy [9]. More frequently, incomplete infarction with hemorrhage but without necrosis occurs. This type of injury resolves quickly and produces only transient radiographic opacities. Infarction always involves the pleural surface of the lung (peripheral) and more frequently involves the lower lobes than other sites.
The regional decrease in ventilation is due to local bronchoconstriction with a tendency for redistribution of ventilation away from the hypoperfused segment. This probably occurs due to decreased regional alveolar and airway carbon dioxide tension, which is the usual stimulus for bronchodilation. This hypocapnia is corrected quickly, since patients inhale carbon dioxide-rich tracheal “dead space air” into the alveolar zones after the embolic event, raising the alveolar pCO2 [7]. Release of neurohumoral factors, most importantly serotonin and thromboxane A2, also causes bronchoconstriction. These factors are released after embolization by activated platelets and mediate bronchospasm of small airways through their effects on the smooth muscles [10]. The ventilation of the hypoperfused areas returns to normal within several hours after acute embolism [11, 12]. This concept is the pathophysiological basis for the scintigraphic interpretation of ventilation and perfusion scans, which show segmental perfusion defects with preserved ventilation as a typical scintigraphic pattern for pulmonary embolism. Those showing only regions of matched perfusion and ventilation defects carry a low probability of pulmonary embolism if no chest X-ray abnormalities are noted at the same sites, since this pattern is more likely associated with nonembolic conditions and is more typical of parenchymal lung disease. Because patients with pulmonary emboli usually arrive at the hospital after normalization of the ventilation at the site of pulmonary emboli, the mismatching pattern is typical of pulmonary emboli.
An increase in the resistance of the pulmonary arterial circulation, due primarily to mechanical blockage by numerous small emboli in the pulmonary vasculature and also to humorally mediated vasoconstriction, may follow pulmonary emboli. These hemodynamic consequences may include increased pulmonary arterial resistance with elevated pulmonary arterial and right ventricular systolic pressures and hypoxemia. When pulmonary hypertension occurs, it indicates that at least 25 % obstruction of pulmonary vascular tree as assessed by angiography [13]. The higher the degree of obstruction, the more severe the abnormalities of the cardiopulmonary hemodynamics become. When over 50 % of the pulmonary vasculature is included (massive pulmonary embolism), acute pulmonary hypertension and/or right ventricular failure (cor pulmonale) occur [13].
11.2.3.2 Resolution
Pulmonary emboli may, spontaneously or with treatment, fragment into smaller portions that travel distally and block smaller arterioles (Fig. 11.4). This may create new, smaller perfusion defects that are more peripherally located in comparison to the original defect caused by the original embolus. This pattern should not be mistaken for recurrent pulmonary emboli on a follow-up scan. If this pattern is the only interval change with no other defects seen in areas other than those in the vicinity of the distribution of the original embolus, it does not suggest recurrent emboli [12].
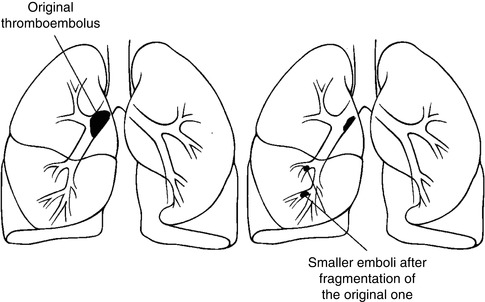
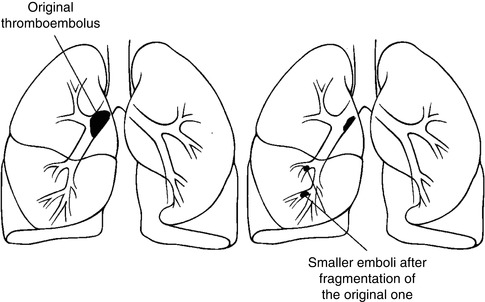
Fig. 11.4
The phenomenon of fragmentation of the thromboemboli (From Wiener et al. [14] with permission)
The resolution of pulmonary thromboembolus may start within hours. It can be seen on perfusion scans as early as 24 h and is progressively noted up to 3 months, with insignificant change after 6 months (Fig. 11.5). This is the basis of the recommendation that follow-up ventilation and perfusion scans is performed three months after the initial incident for the evaluation of resolution and function as a baseline for future incidents to differentiate between acute and unresolved old emboli. This resolution is dependent on the age of the patient, with complete resolution in young age-groups and less complete and less significant resolution in older age-groups [15, 16].
11.2.3.3 Chronic Pulmonary Thromboembolism
Incomplete resolution of acute pulmonary embolism is frequently observed and may rarely result in chronic thromboembolic pulmonary hypertension [17]. Chronic thromboembolic disease is characterized by intraluminal thrombus organization and fibrous stenosis or complete obliteration of pulmonary arteries. The consequence is an increased pulmonary vascular resistance resulting in pulmonary hypertension and progressive right heart failure. Pulmonary endarterectomy is the preferred treatment [18].
11.2.3.4 Recurrence
Pulmonary thromboemboli recur in up to 50 % of patients [19], although the incidence in treated patients was only 8.3 % among PIOPED (prospective investigation of pulmonary embolism diagnosis) patients [20]. The vast majority of deaths among pulmonary embolism patients are due to recurrent emboli. In the PIOPED study population, it was found that nine of ten people who died had a recurrent pulmonary embolus [21]. Recurrence has been reported to occur at the same site as the original thromboembolus [22].
11.2.3.5 Diagnosis
The clinical diagnosis of pulmonary thromboembolism is difficult and unreliable, due to the nonspecificity of its symptoms and signs as well as the laboratory and chest X-ray findings [4]. Chest X-ray, however, must be obtained since it may show many parenchymal diseases and must be available for lung scan interpretation. Pulmonary embolism may also be asymptomatic. In the literature, only 24 % of fatal emboli were diagnosed antemortem (Table 11.2) [31]. Data indicate that the mortality of pulmonary embolism is more than 30 % if untreated. Promptly diagnosed and treated, emboli have a mortality of 2.5–8 % [11, 16, 21]. Pulmonary angiography is the most accurate modality for the diagnosis of pulmonary emboli with an accuracy of 96 % [32]. However, angiography is invasive and is not suitable as a screening imaging modality for the disease.
Table 11.2
Cases with antemortem PE diagnosis
Author | Year | No. (%) of cases with antemortem PE diagnosis |
---|---|---|
Stein and Henry [23]
![]() Stay updated, free articles. Join our Telegram channel![]() Full access? Get Clinical Tree![]() ![]() ![]() |