Part 6 Brain and Spine Imaging
Case 151
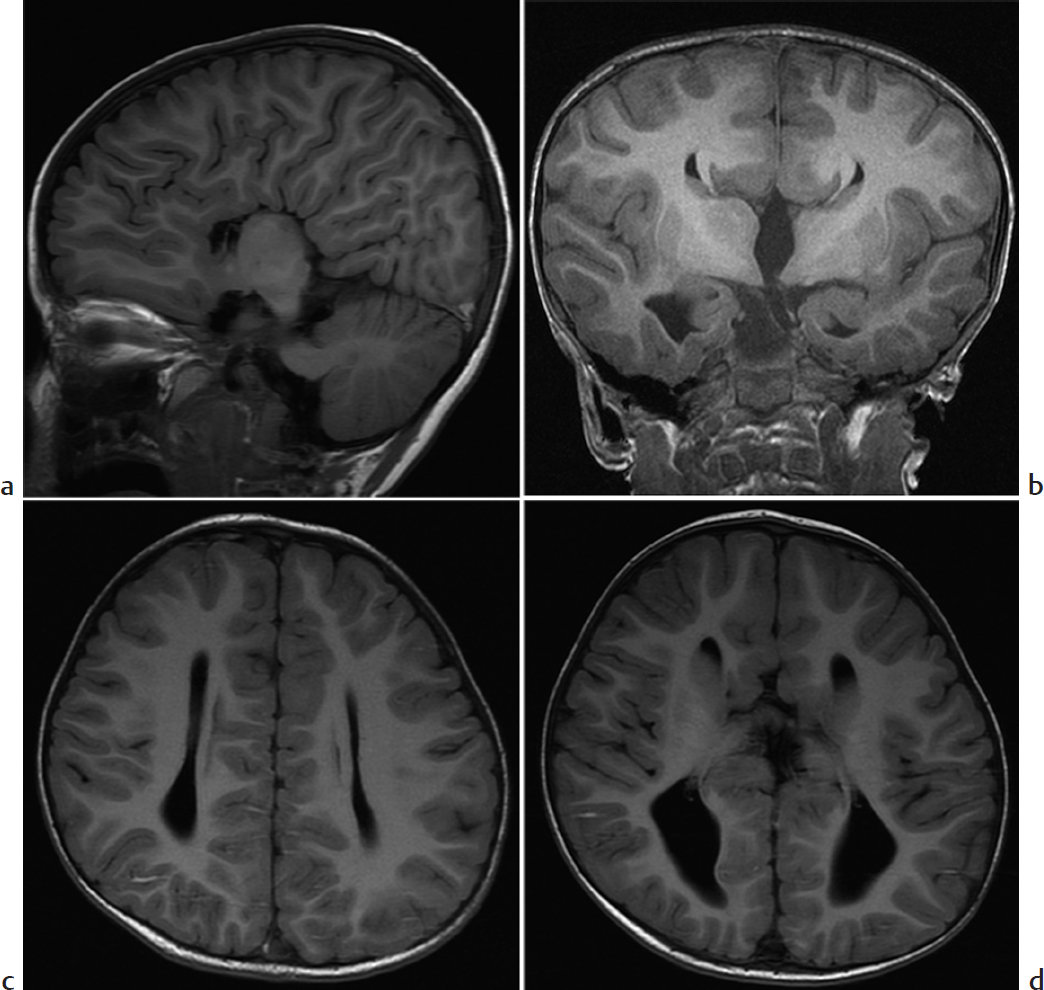
Key Imaging Finding
Absence of the corpus callosum.
Top Three Differential Diagnoses
Agenesis of the corpus callosum (ACC). ACC is caused by failure of axons to form or migrate across the midline. Most patients with isolated ACC are initially clinically normal, but subtle cognitive defects, visual problems, and seizures may develop later. US and MRI performed either prenatally or after birth show similar findings. There is colpocephaly, with parallel configuration of lateral ventricles and dilated occipital horns. Midline images show a high riding third ventricle. The corpus callosum and cingulate sulcus are absent, and the gyri radiate out from the third ventricle. On coronal imaging, Probst bundles run parallel to interhemispheric fissure and indent the lateral ventricles, creating a “bull’s horn” configuration. About 15% of cases initially thought to be isolated turn out to be associated with other abnormalities, conferring a worse prognosis. Postnatal MRI and karyotype evaluation help assess for associated anomalies.
Chiari 2 malformation with colpocephaly. This complex hindbrain malformation is closely associated with neural tube defects. Most patients are diagnosed at prenatal screening with elevated alpha-fetoprotein and abnormal US. Most others present at birth with hydrocephalus and myelomeningocele, but milder cases may present later with varying degrees of lower extremity paresis/spasticity, clubfoot, bowel/bladder dysfunction, and epilepsy. On MRI, the characteristic findings include a crowded posterior fossa, callosal agenesis/dysgenesis, tectal beaking, inferior tonsillar and vermian displacement, and medullary kinking. Prenatal US classically shows cerebellar compression (banana sign), frontal bone concavity (lemon sign), and ventriculomegaly. Prenatal folate reduces the incidence of Chiari 2 malformation. Myelomeningocele is surgically repaired within 48 hours of birth, and hydrocephalus may be treated with CSF shunt.
Secondary damage to the corpus callosum. The corpus callosum may be injured due to head trauma or in the course of neurosurgery. In addition, patients with extensive periventricular leukomalacia of prematurity—and other periventricular white matter injury—may develop severe thinning of the corpus callosum. The corpus callosum may also be so stretched by hydrocephalus that it appears extremely thin. Even after hydrocephalus has resolved, the corpus callosum may remain abnormal.
Additional Differential Diagnosis
Holoprosencephaly. This condition consists of incomplete separation of the cerebral hemispheres. In addition, there is agenesis of part or all of the corpus callosum, corresponding to the degree of anatomical severity (alobar, semilobar, and lobar).
Diagnosis
Agenesis of the corpus callosum.
Pearls
Agenesis of the corpus callosum demonstrates interhemispheric fissures radiating from the third ventricle.
Chiari 2 malformations are complex hindbrain malformations that usually occur in association with myelomeningocele.
The corpus callosum may appear defective as a result of trauma, surgery, hydrocephalus, or injury to the periventricular white matter.
Suggested Readings
Cesmebasi A, Loukas M, Hogan E, Kralovic S, Tubbs RS, Cohen-Gadol AA. The Chiari malformations: a review with emphasis on anatomical traits. Clin Anat. 2015; 28(2):184–194 Craven I, Bradburn MJ, Griffiths PD. Antenatal diagnosis of agenesis of the corpus callosum. Clin Radiol. 2015; 70(3):248–253Case 152
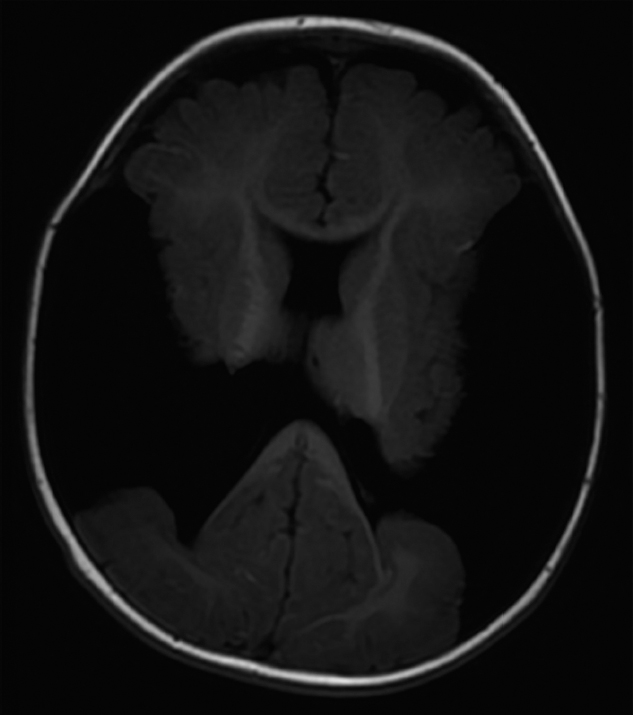
Clinical Presentation
A 7-month-old boy with developmental delay, hypotonia, and seizures (▶Fig. 152.1).
Key Imaging Finding
CSF-filled cleft/space in a young infant.
Top Three Differential Diagnoses
Schizencephaly. Schizencephaly results from early destruction of a portion of the germinal matrix and adjacent brain before the hemispheres are fully formed. This results in a gray-matter-lined cleft extending from the body of the lateral ventricle(s) to the outer cortical surface. The cleft is lined with polymicrogyric gray matter. With “closed lip” clefts, the edges of the cleft are fused in a pial-ependymal seam, with no intervening CSF (type I). CSF separates “open lip” clefts (type II); these clefts are usually large and sometimes bilateral. Septo-optic dysplasia is often associated, along with heterotopia and cortical dysplasia. Clinical manifestations include intellectual disability, seizures, and motor dysfunction. More severe malformations (open lip, bilateral, involving motor cortex) result in more severe neurodevelopmental disabilities. Patients with bilateral clefts are severely disabled. MRI and CT show gray matter lining the cleft. A dimple in the ventricle wall indicates the site of closed lip schizencephaly.
Porencephaly. This entity has many definitions, but pathologically it is defined as a cavity within the brain with minimal surrounding gliosis, or astrocyte proliferation. The cyst is lined with dysplastic white matter. Porencephaly is often congenital, due to a fetal cerebrovascular accident or infection. It may develop in early infancy, after head trauma, vascular insult, or infection. It does not usually develop in older children whose brains respond to insult with gliosis rather than necrosis. On cross-sectional imaging, porencephaly appears as one or more unilateral or bilateral cavity(ies) lined with gliotic white matter, usually communicating with the ventricular system or the subarachnoid space. There is ex vacuo dilatation of the adjacent ventricle. Cyst walls are smooth, and adjacent white matter appears bright on inversion recovery (IR) sequences.
Arachnoid cyst. During the development of the brain, the meninges in a limited area may fail to merge, forming a duplicated arachnoid layer that allows interlayer accumulation of CSF. Most occur at the sylvian fissure and are asymptomatic. Arachnoid cysts are well demarcated and hypodense on CT, and they usually follow simple CSF signal on all MR pulse sequences. Occasionally, the contents are slightly complex due to internal hemorrhage. These lesions do not enhance.
Additional Differential Diagnosis
Postinfarct encephalomalacia. A variety of parenchymal insults in older children may lead to parenchymal volume loss, usually with accompanying ventricular enlargement. The pathological process differs from that preceding porencephaly. The defect often appears wedge-shaped and does not typically communicate with the ventricle or cortex.
Diagnosis
Bilateral open lip schizencephaly.
Pearls
Schizencephaly consists of a polymicrogyric gray-matterlined cleft extending from the lateral ventricle to the cortical mantle.
Closed lip clefts are fused in a pial-ependymal seam, whereas open lip clefts have a gap filled with CSF.
Porencephalic cysts are lined by white matter, not gray matter.
Suggested Readings
Epelman M, Daneman A, Blaser SI, et al. Differential diagnosis of intracranial cystic lesions at head US: correlation with CT and MR imaging. Radiographics. 2006; 26(1):173–196 Hayashi N, Tsutsumi Y, Barkovich AJ. Morphological features and associated anomalies of schizencephaly in the clinical population: detailed analysis of MR images. Neuroradiology. 2002; 44(5):418–427 Oh KY, Kennedy AM, Frias AE Jr, Byrne JL. Fetal schizencephaly: pre- and postnatal imaging with a review of the clinical manifestations. Radiographics. 2005; 25(3):647–657Case 153
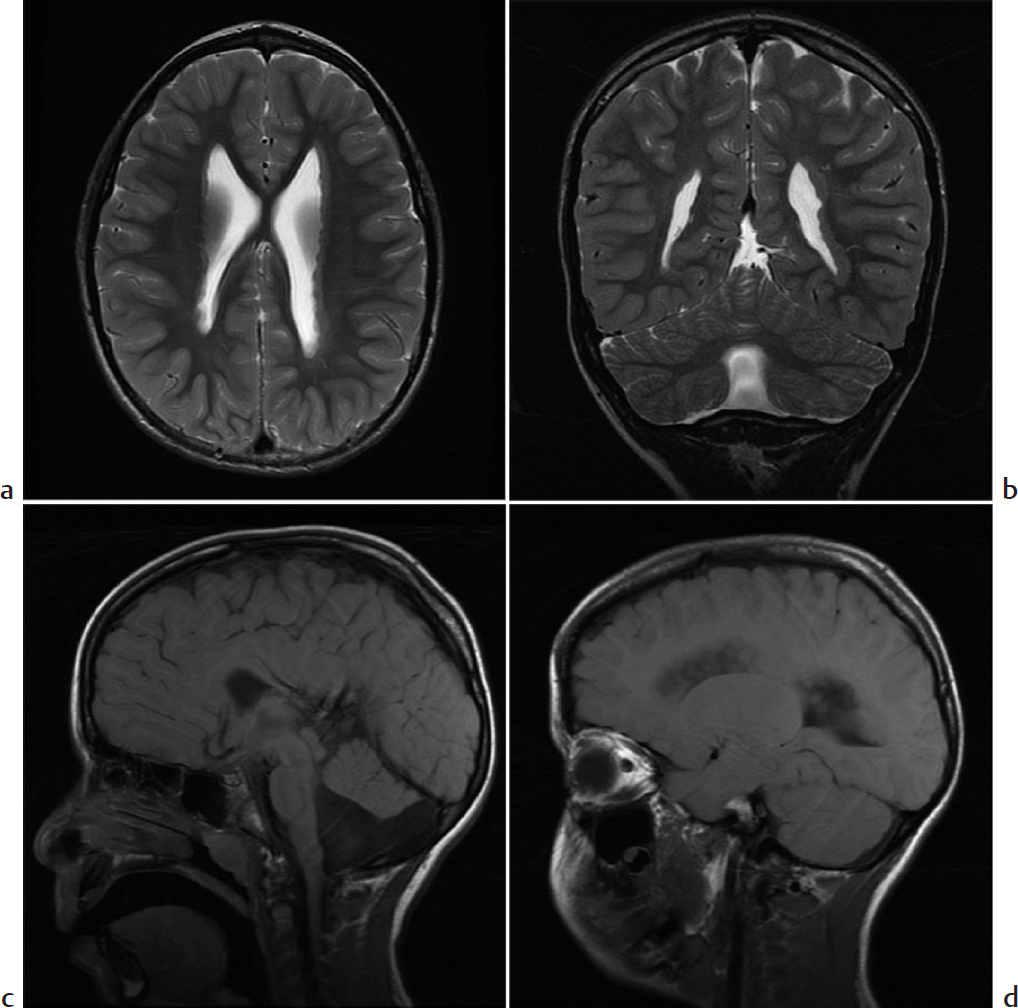
Key Imaging Finding
Nodularity of the ventricular wall.
Top Three Differential Diagnoses
Gray matter heterotopia. Gray matter heterotopia refers to anomalous gray matter deposition due to abnormal neuronal migration during fetal development. Patients may present with seizures and/or developmental delay. Heterotopic gray matter may be seen anywhere along the course of neuronal migration but occurs most often in a periventricular nodular pattern. Single or multiple nodules may also be seen in a cortical/subcortical location or on the pial surface. Diffuse patterns of heterotopia include band heterotopia and lissencephaly. Nodules are isointense to cortical gray matter on all sequences and do not enhance.
Tuberous sclerosis complex (TSC). Subependymal hamartomas are a common feature of TSC, which is an acquired or hereditary genetic phakomatosis caused by mutations in TSC1 or TSC2 genes. These genes encode harmartin and tuberin, respectively. TSC is characterized by hamartomatous growths involving multiple organ systems. These include multiple subependymal nodules and cortical/subcortical as well as cerebellar tubers, which progressively calcify in early childhood. Tubers may be T2/FLAIR hyperintense and initially hypodense on CT; if they do not calcify, they may appear cystic. Patients with TSC may develop subependymal giant cell astrocytoma (SEGA), a World Health Organization (WHO) grade 1 tumor that typically forms near the foramen of Monro and demonstrates heterogeneous T1/T2 signal, calcification, strong contrast enhancement, and progressive growth. TSC patients also commonly develop renal angiomyolipomas and cardiac rhabdomyomas. Patients often present with seizures and adenoma sebaceum.
Closed lip schizencephaly. Schizencephaly results from early destruction of a portion of the germinal matrix and adjacent brain before the hemispheres are fully formed. This results in a gray-matter-lined cleft extending from the body of the lateral ventricles to the outer cortical surface. The cleft is lined with polymicrogyric gray matter. With “closed lip” clefts, the edges of the cleft are fused in a pial-ependymal seam, with no intervening CSF (type I). CSF separates “open lip” clefts (type II); these clefts are usually large and sometimes bilateral. Closed lip schizencephaly may be quite subtle on MRI. The presence of slight irregularity in the lateral wall of the lateral ventricle may be the only manifestation on axial MRI. There may be a tiny dimple in the wall where the cleft meets the lateral ventricle, or mild nodularity adjacent to this area. Gray matter may be identified along the cleft at both CT and MRI. Septo-optic dysplasia is often associated, along with heterotopia and cortical dysplasia. Clinical manifestations include intellectual disability, seizures, and motor dysfunction.
Diagnosis
Gray matter heterotopia in a patient with Dandy–Walker variant.
Pearls
Gray matter heterotopia describes a disorder of neuronal migration resulting in nodular or diffuse heterotopic gray matter, most often appearing in a periventricular nodular pattern.
Tuberous sclerosis complex is a multisystem phakomatosis, which presents with seizures, subependymal nodules, and cerebral and/or cerebellar tubers that progressively calcify; patients are at increased risk for subependymal giant cell astrocytoma.
Closed lip schizencephaly may be quite subtle, manifesting as a small dimple at the site of the cleft or slight nodularity on either side.
Suggested Readings
Abdel Razek AA, Kandell AY, Elsorogy LG, Elmongy A, Basett AA. Disorders of cortical formation: MR imaging features. AJNR Am J Neuroradiol. 2009; 30(1):4–11 Daghistani R, Rutka J, Widjaja E. MRI characteristics of cerebellar tubers and their longitudinal changes in children with tuberous sclerosis complex. Childs Nerv Syst. 2015; 31(1):109–113Case 154
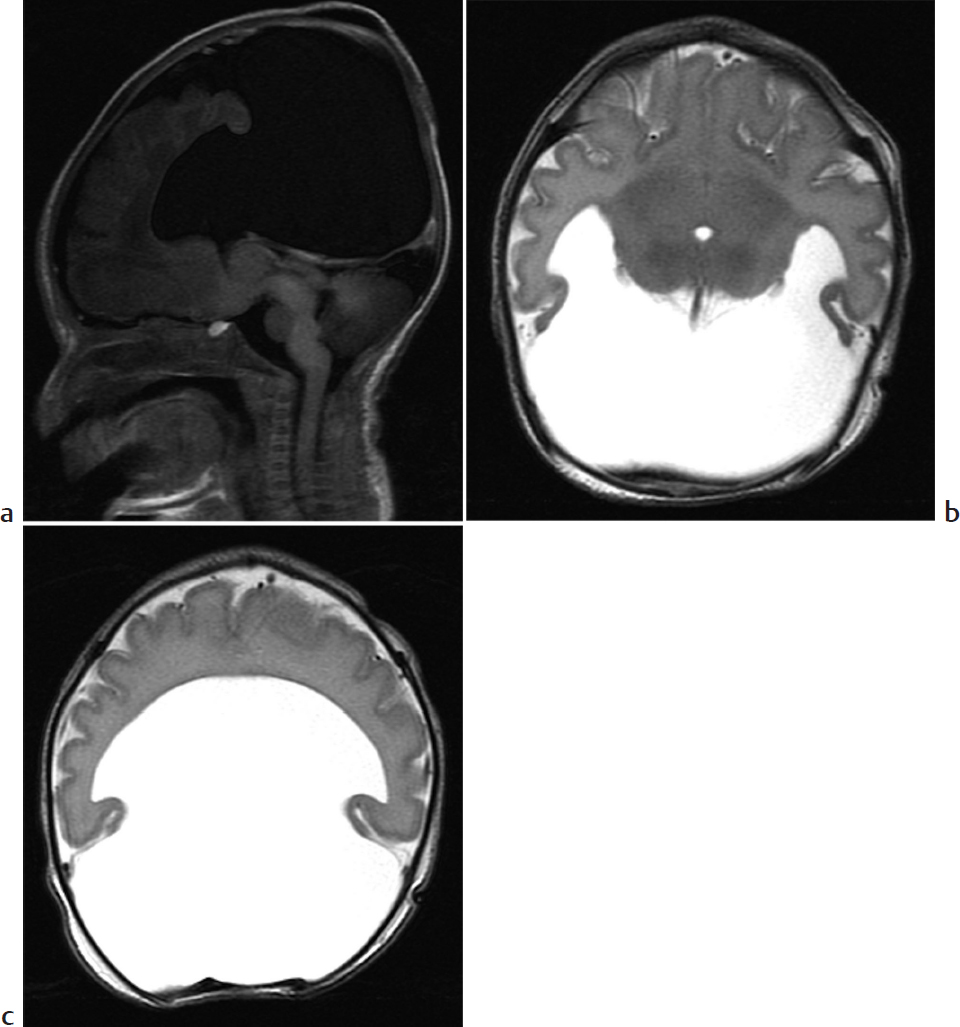
Key Imaging Finding
Large amount of supratentorial CSF.
Top Three Differential Diagnoses
Massive hydrocephalus. Obstruction, overproduction, and/or decreased absorption of CSF may cause ventricular dilatation. If hydrocephalus is severe, the brain appears as an almost imperceptible rim of cortex compressed along the contour of the skull. The presence of a midline falx and absence of thalamic fusion help differentiate this condition from holoprosencephaly, and the presence of a cortical mantle differentiates it from hydranencephaly. This distinction is important, since early shunting of hydrocephalus can allow normal intellect and motor function to develop. In newborns, aqueductal stenosis is a common cause of hydrocephalus affecting the third and lateral ventricles, but neoplasms and posthemorrhagic/infectious obstruction should also be considered. Hemorrhage and infection may also cause communicating hydrocephalus.
Hydranencephaly. A variety of insults between gestational weeks 20 to 27 can lead to massive liquefaction of the supratentorial parenchyma. The inferomedial frontal and temporal lobes as well as the thalami may be normal, the brainstem is atrophic, and the cerebellum is usually normal. The falx is present and the thalami are separate, but there is no cortical mantle, which helps differentiate this entity from massive hydrocephalus and alobar holoprosencephaly. Head circumference varies depending on whether there is also hydrocephalus. Patients are severely neurologically impaired.
Alobar holoprosencephaly. This most severe form of holoprosencephaly develops when the prosencephalon does not divide into separate hemispheres. The cerebral parenchyma is pancakelike or ball-shaped on prenatal US. It is fused anteriorly and surrounds a large dorsal interhemispheric cyst. The corpus callosum, falx, and interhemispheric and sylvian fissures are absent; the basal ganglia, hypothalami, and thalami are fused at the midline. Facial abnormalities are common, including hypotelorism, fused metopic suture (causing trigonocephaly), and cleft palate. About half of cases are associated with a syndrome, often trisomy 13. Lobar holoprosencephaly is the mildest form, characterized by separate thalami but no septum pellucidum and a variable amount of falx. Semilobar holoprosencephaly is intermediate, having fused thalami and intermediate appearance of other structures.
Additional Differential Diagnosis
Bilateral open lip schizencephaly. Large bilateral clefts may severely distort anatomy, and a large amount of CSF may accumulate in the defects. Polymicrogyric gray matter should be evident lining the clefts. A falx is present. There may be associated septo-optic dysplasia.
Diagnosis
Alobar holoprosencephaly.
Pearls
Massive hydrocephalus has a thin, sometimes imperceptible, cortical mantle, along with a falx and unfused thalami.
Patients with hydranencephaly have a falx and separate thalami, but there is no cortical mantle; furthermore, the brainstem is atrophic.
Alobar holoprosencephaly lacks a falx, and the thalamus is fused in the midline.
Suggested Readings
Barkovich AJ, Raybaud C. Congenital malformations of the brain and skull. In Barkovich AJ, Raybaud C, eds. Pediatric Neuroimaging, 5th ed. Philadelphia, PA: Lippincott Williams & Wilkins; 2012:367–568 Medina LS, Frawley K, Zurakowski D, Buttros D, DeGrauw AJ, Crone KR. Children with macrocrania: clinical and imaging predictors of disorders requiring surgery. AJNR Am J Neuroradiol. 2001; 22(3):564–570Case 155
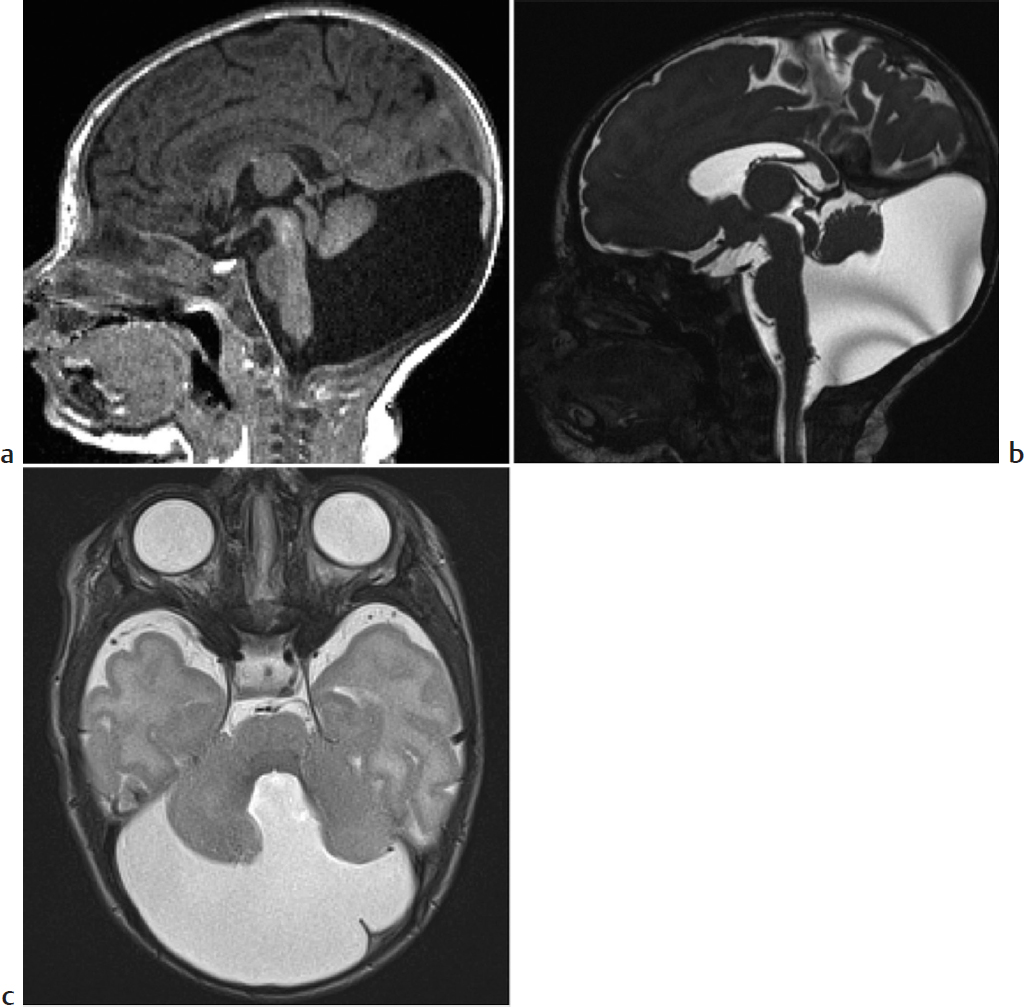
Key Imaging Finding
Posterior fossa cyst in a newborn.
Top Three Differential Diagnoses
Mega cisterna magna. Patients with mega cisterna magna have an enlarged cisterna magna but a normal cerebellar vermis and no hydrocephalus. The cisterna magna is considered “mega” when it measures ≥10 mm on sagittal midline images. The fourth ventricle generally appears normal, but the posterior fossa may be enlarged. Isolated mega cisterna magna is considered a normal variant.
Dandy–Walker malformation (DWM). This relatively common posterior fossa malformation is caused by an absent or very small cerebellar vermis, with other portions of the cerebellum also possibly affected. It is often diagnosed prenatally but may present later with developmental delay, hypotonia/spasticity, and ataxia. Classic DWM shows an enlarged posterior fossa with a hypoplastic or occasionally absent cerebellar vermis. A cystlike, dilated fourth ventricle fills the posterior fossa, and there is hypoplasia and rotation of the inferior cerebellar vermis. The severity of hypoplasia of the cerebellar vermis and hemispheres varies. DWM includes elevation of the torcula and transverse dural venous sinuses, as well as the tentorium. Associated malformations include callosal anomalies, polymicrogyria, gray matter heterotopias, and occipital encephaloceles. Hydrocephalus may precipitate diagnosis. The presence of a cerebellar vermis that has normal size and rotation excludes DWM.
Blake pouch cyst (BPC). This results from failure of Blake pouch, a membrane at the floor of the fourth ventricle, to fenestrate normally during development. The BPC is thus a fetal remnant. It herniates from the fourth ventricle through the foramen of Magendie, restricting CSF flow into the subarachnoid space. MRI may show the choroid plexus extending into the cyst, important for diagnosis. Although some patients are asymptomatic, obstructive hydrocephalus is common. Treatment is third ventriculostomy.
Additional Differential Diagnoses
Arachnoid cyst. A retrocerebellar or superior vermian posterior fossa arachnoid cyst may cause mass effect, compressing or displacing a normal fourth ventricle. There are usually no associated anomalies.
Cerebellar hypoplasia. Cerebellar hypoplasia is characterized by a small cerebellum with normal size of the fissures and cerebellar folia. Any component of the cerebellum (cerebellar hemispheres or vermis)—or indeed the entire cerebellum—may be hypoplastic. Additional features of Dandy–Walker malformation are absent.
Joubert syndrome. This is defined by the characteristic “molar tooth” appearance on MRI: hypoplastic cerebellar vermis, enlarged and elongated superior cerebellar peduncles that do not decussate and have a horizontal orientation, and a deep interpeduncular fossa. Inheritance is predominantly autosomal recessive.
Diagnosis
Dandy–Walker malformation.
Pearls
The normal cisterna magna measures less than 10 mm at the midline on a sagittal image.
Dandy–Walker malformation is defined by an enlarged posterior fossa with a hypoplastic cerebellar vermis and is commonly associated with hydrocephalus and additional congenital anomalies.
Blake pouch cyst may be recognized by the presence of choroid plexus within the cystic space.
Joubert syndrome features a “molar tooth” appearance of the cerebellum.
Suggested Readings
Barkovich AJ, Raybaud C. Congenital malformations of the brain and skull. In Barkovich AJ, Raybaud C, eds. Pediatric Neuroimaging, 5th ed. Philadelphia, PA: Lippincott Williams & Wilkins; 2012:367–568 Bosemani T, Orman G, Boltshauser E, Tekes A, Huisman TA, Poretti A. Congenital abnormalities of the posterior fossa. Radiographics. 2015; 35(1):200–220 Cornips EM, Overvliet GM, Weber JW, et al. The clinical spectrum of Blake’s pouch cyst: report of six illustrative cases. Childs Nerv Syst. 2010; 26(8):1057–1064Case 156
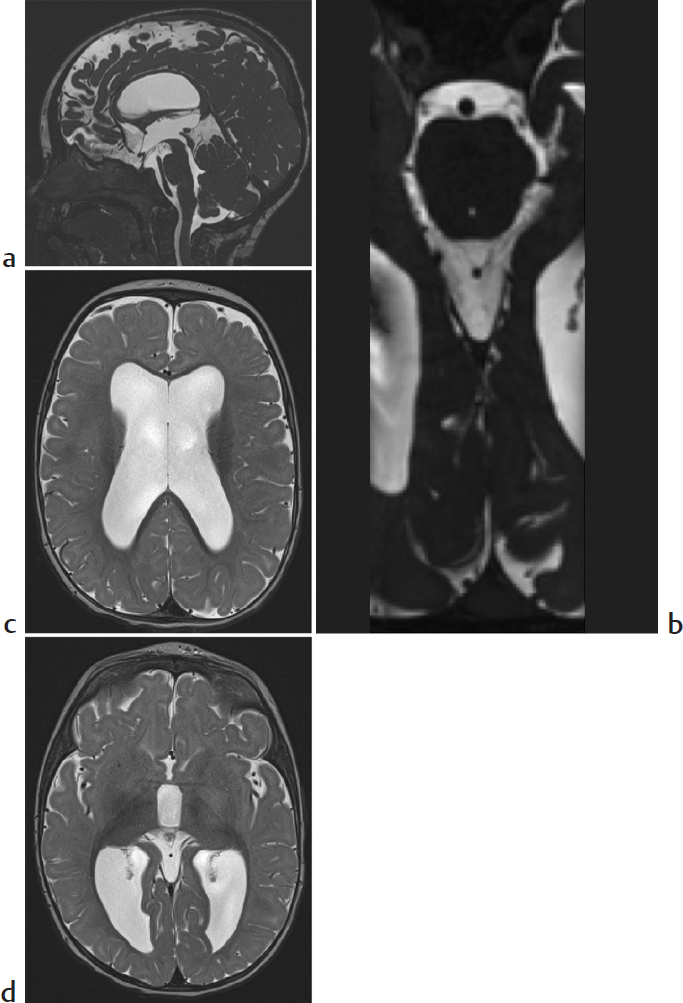
Key Imaging Finding
Hydrocephalus.
Top Three Differential Diagnoses
Post-inflammatory hydrocephalus. Intracranial hemorrhage or leptomeningeal infection may cause acquired hydrocephalus. Both entities often lead to ependymal inflammation. This—sometimes along with exudate or blood—may impair the flow or resorption of CSF, potentially resulting in communicating or, less often, noncommunicating hydrocephalus. In addition, chronic sequelae of inflammation sometimes cause fibrosis of the subarachnoid spaces, similarly impairing CSF flow and leading to delayed hydrocephalus. If only the lateral and third ventricles are enlarged, this would be considered acquired cerebral aqueductal stenosis.
Obstructive hydrocephalus. A mass or cyst may obstruct the ventricular system anywhere. However, obstructive hydrocephalus most often occurs when the mass or cyst grows into the CSF outflow tracts, especially the aqueduct of Sylvius, the foramina of Monro, Magendie, and Luschka, and the third and fourth ventricles. CT depicts mass effect and may partly delineate the offending lesion. However, MRI is far superior and is essential for accurate diagnosis. Acute hydrocephalus may develop if mass effect causes acute obstruction, leading to a rapid increase in intracranial pressure. Patients present with headache, papilledema, poor feeding, irritability, nausea, and/or vomiting. Enlargement of the temporal horns of the lateral ventricles is the most sensitive indicator of acute obstructive hydrocephalus. In addition, there may be transependymal edema due to rapid increase in intraventricular pressure, appearing as periventricular T2 prolongation on MRI and hypodensity on CT. CSF spaces may be effaced. Severe acute hydrocephalus may cause brain herniation.
Congenital cerebral aqueductal stenosis. Narrowing of the aqueduct of Sylvius may prevent outflow of CSF and lead to supratentorial obstructive hydrocephalus. To be considered congenital, there must be no causative lesion for such a mass or prior inflammation/hemorrhage. MRI is essential for excluding acquired causes of hydrocephalus and allowing definitive diagnosis of congenital aqueductal stenosis. MRI sequences should include thin section sagittal imaging through the midline. The superior portion of the aqueduct often appears funneled, and the narrowing may be focal or more extensive.
Diagnosis
Congenital cerebral aqueductal stenosis.
Pearls
Aqueductal stenosis shows dilatation of the lateral and third ventricles with a normal-sized fourth ventricle.
Acute hydrocephalus may show transependymal fluid shift, and it is important to evaluate for evidence of herniation.
Both hemorrhage and infection may cause post-inflammatory hydrocephalus.
Suggested Readings
Barkovich AJ, Raybaud C. Congenital malformations of the brain and skull. In Barkovich AJ, Raybaud C, eds. Pediatric Neuroimaging, 5th ed. Philadelphia, PA: Lippincott Williams & Wilkins; 2012:367–568 Medina LS, Frawley K, Zurakowski D, Buttros D, DeGrauw AJ, Crone KR. Children with macrocrania: clinical and imaging predictors of disorders requiring surgery. AJNR Am J Neuroradiol. 2001; 22(3):564–570Case 157
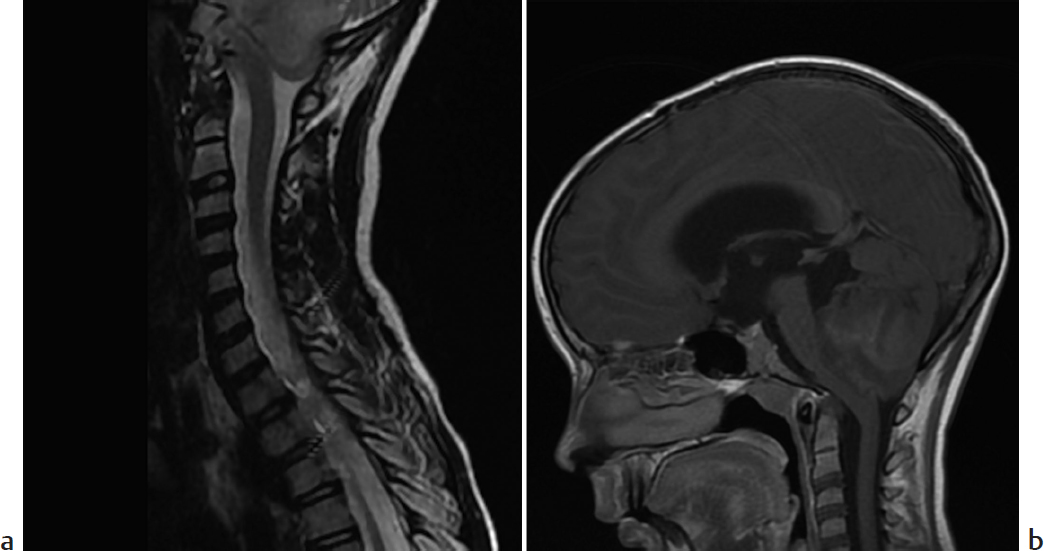
Key Imaging Finding
Tonsillar herniation.
Top Three Differential Diagnoses
Chiari 1 malformation. Most cases of Chiari 1 result from a small posterior fossa. This leads to a constellation of findings, including inferior displacement and elongation of the cerebellar tonsils, crowding of the posterior fossa, and compression of CSF spaces. Common symptoms include headache and neck pain, but up to 50% of patients are asymptomatic. On MRI, the cerebellar tonsils appear pointed and typically extend at least 5 mm below the foramen magnum (more than 6 mm is abnormal between ages 5 and 15 years). There is effacement of the foramen magnum. Cisterns are small or absent, and the fourth ventricle may appear elongated. Syringohydromyelia is common. Other associated abnormalities include basilar invagination and other osseous skull base anomalies, hydrocephalus, and fourth occipital sclerotome syndromes.
Chiari 2 malformation. This complex hindbrain malformation occurs in the setting of neural tube closure defects. Folate deficiency and genetic predisposition (the methylene tetrahydrofolate reductase [MTHFR] mutation) combine to prevent neural tube closure at around estimated EGA 4 weeks. The open neural tube allows CSF to drain freely from the spine, and as a result mesenchyme does not develop normally. This leads to a small posterior fossa. There may also be absence of the corpus callosum and septum pellucidum, gray matter heterotopia, and dysmorphic posterior gyri. Characteristic findings on MRI include a crowded posterior fossa, tectal beaking, and inferior displacement of the medulla with cervicomedullary kinking. Supratentorial findings may include colpocephaly, hydrocephalus, fenestration of the falx with interdigitation of gyri, and absence of the corpus callosum. Classic features of the fetal brain include cerebellar compression (banana sign), frontal bone concavity (lemon sign), and ventriculomegaly.
Acute herniation. The most common cause of acute brain herniation in children is a posterior fossa mass. Other etiologies include trauma, large infarcts, and inflammation. Acute herniation may be diagnosed with unenhanced CT. The cerebellar tonsils are pushed more than 5 mm inferiorly, impacted into the foramen magnum. The cisterna magna is obliterated, and hydrocephalus is common. MRI is usually needed to determine underlying etiology and assess for such complications as edema, hemorrhage, and infarcts. Other types of herniation include subfalcine, transalar, and transtentorial.
Diagnosis
Hydrocephalus and cerebellar tonsillar herniation secondary to medulloblastoma.
Pearls
Findings of Chiari 1 malformation include inferiorly displaced, elongated cerebellar tonsils, and a crowded posterior fossa; syringohydromyelia is common as well.
Chiari 2 malformation is a complex hindbrain malformation that is virtually always associated with a neural tube defect.
A posterior fossa mass is the most common cause of acute tonsillar herniation, resulting in obliteration of the cisterna magna and often secondary hydrocephalus and infarct.
Suggested Readings
Hussain SI, Cordero-Tumangday C, Goldenberg FD, Wollman R, Frank JI, Rosengart AJ. Brainstem ischemia in acute herniation syndrome. J Neurol Sci. 2008; 268(1–2):190–192 McVige JW, Leonardo J. Imaging of Chiari type I malformation and syringohydromyelia. Neurol Clin. 2014; 32(1):95–126Case 158
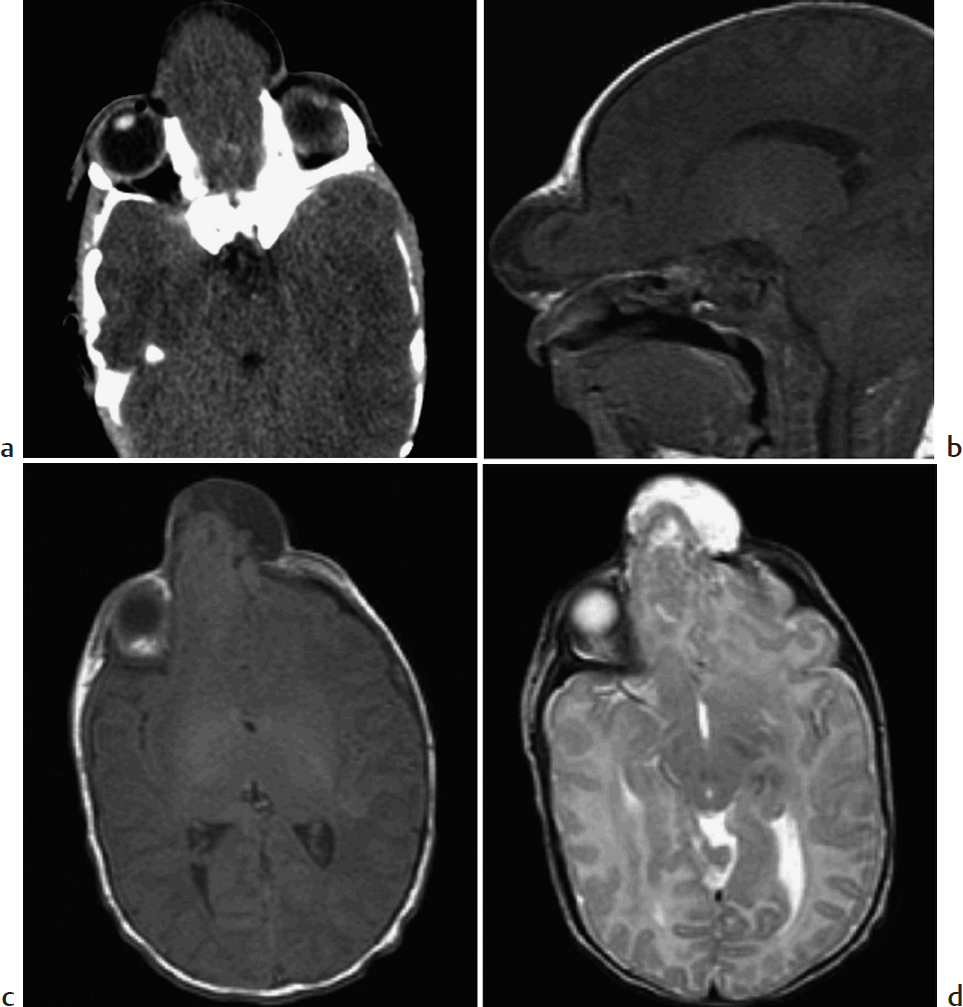
Key Imaging Finding
Congenital midline nasal mass.
Top Three Differential Diagnoses
Dermoid/epidermoid. These lesions present as a nasal mass, cyst, sinus, or draining fistula and are most common at the dorsum of the nose. They result from abnormal separation of dura and skin, which initially develop in close approximation. The dura should subsequently retract, and the foramen cecum should form a barrier between skin and dura. A nasal dermoid or epidermoid forms if the foramen does not close and/or if dural tissue does not retract. Dermoids are more common than epidermoids and are slightly more common in boys. They consist of both ectoderm and mesoderm, whereas epidermoids are ectoderm only. Dermoids may communicate with the CNS, whereas epidermoids typically do not. CT demonstrates an osseous defect. MRI depicts the defect as well as the lesion’s contents. A dermoid has variable T1 and T2 signal, since it may contain fat as well as fluid. Fat suppression techniques are useful for diagnosing fat. Epidermoids contain simple fluid and—important for diagnosis—show restricted diffusion. The rims of both lesions may enhance. Since both dermoids and epidermoids are prone to infection—including, for dermoids, meningitis and cerebral abscess—complete resection of the cyst and potential tract is mandatory.
Nasofrontal encephalocele. Formation of nasofrontal encephaloceles is similar to that of dermoids and epidermoids, but in addition the residual dura retains communication with the subarachnoid space. Only 15% of encephaloceles are anterior. Patients often also have anophthalmia, microphthalmia, microcephalus, hydrocephalus, dysgenesis of the corpus callosum, and/or cortical atrophy. Encephaloceles appear as soft, bluish masses that transilluminate and increase in size with Valsalva. They are located at the midline of the nose, glabella, or both. MRI shows a soft-tissue mass in continuity with the subarachnoid space, whose signal characteristics match those of the brain. CT demonstrates the osseous defect more clearly than does MRI. It is essential to image all nasal masses before biopsy, in order to exclude encephalocele.
Nasal cerebral heterotopia (aka glioma). Development of these unusual masses is similar to that of dermoids. However, rests of sequestered glial tissue are retained, along with fibro-vascular tissue. About 60% of these heterotopias are extranasal, and about 30% are intranasal; the rest span both regions. A few connect to the brain via a fibrous stalk that traverses the foramen cecum to end at the subarachnoid space, but most do not connect to neural tissue. Most patients present with a red or bluish, firm mass at the glabella, nasomaxillary suture, or within the nose. They uncommonly present with meningitis or CSF rhinorrhea. Nasal cerebral heterotopia appears as a soft-tissue mass at CT or MRI. Signal tends to be brighter than normal brain on T2, since the neural tissue is gliotic and dysplastic. It is important to differentiate nasal gliomas from encephaloceles.
Diagnosis
Nasofrontal encephalocele.
Pearls
Dermoids are the most common congenital nasal mass, may communicate with the CNS, and contain fluid, fat, skin, and/or bone.
Epidermoids contain only fluid, do not communicate with the CNS, and demonstrate restricted diffusion.
MRI of nasofrontal encephaloceles shows neural tissue in continuity with the frontal lobe.
Nasal cerebral heterotopias may connect to the subarachnoid space; their T2 signal is brighter than that of brain.
Suggested Readings
Adil E, Huntley C, Choudhary A, Carr M. Congenital nasal obstruction: clinical and radiologic review. Eur J Pediatr. 2012; 171(4):641–650 Kadom N, Sze RW. Radiological reasoning: pediatric midline nasofrontal mass. AJR Am J Roentgenol. 2010; 194(3) Suppl:WS10–WS13 Saettele M, Alexander A, Markovich B, Morelli J, Lowe LH. Congenital midline nasofrontal masses. Pediatr Radiol. 2012; 42(9):1119–1125Case 159
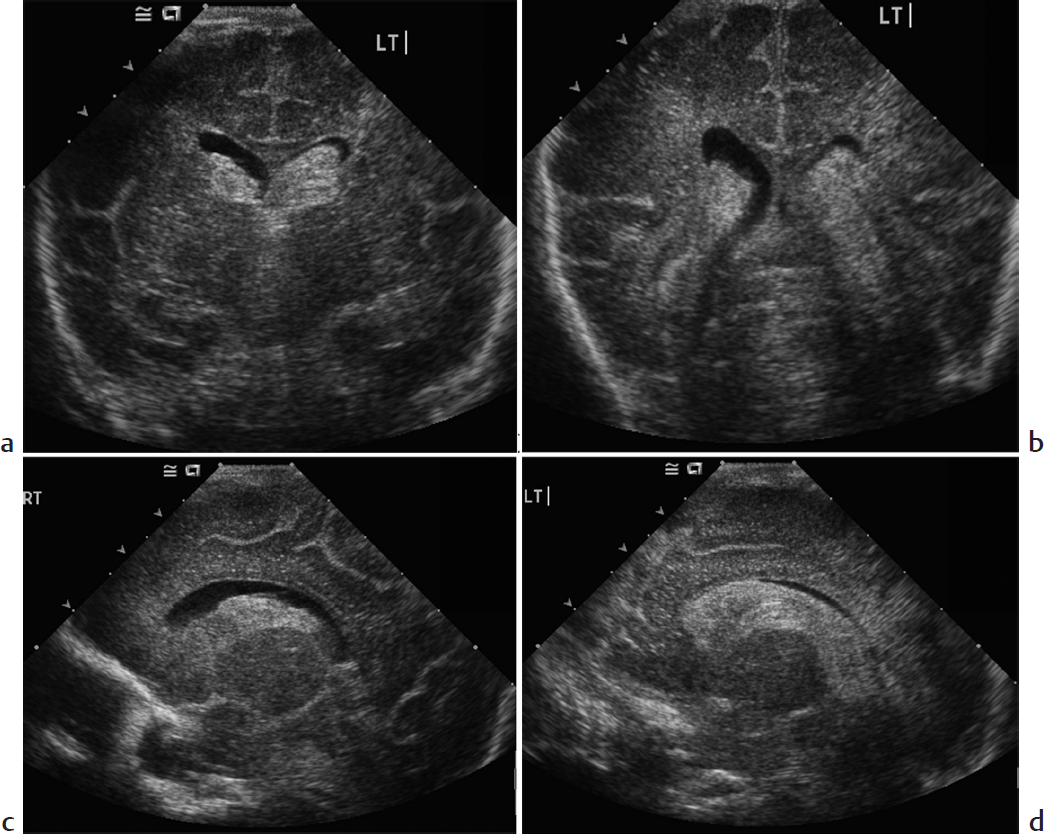
Key Imaging Finding
Periventricular/intraventricular hemorrhage in a premature infant.
Top Three Differential Diagnoses
Grade 1 germinal matrix hemorrhage (GMH). GMH accounts for about three-fourths of intracranial hemorrhage in preterm infants. It develops in about half of those who are very preterm. The germinal matrix (GM) is subependymal in location, initially located along the entire length of the lateral ventricles. By EGA 35 weeks, it is limited to the caudothalamic groove. Glial and neural cells develop in and migrate from this highly vascular area. Fragile vessels in the GM may rupture due to fluctuating blood flow in premature infants who lack cerebral autoregulation. GMH initially appears echogenic, bulging into the floor of the lateral ventricle. The hemorrhage retracts, becomes cystic, and eventually disappears. Clinical sequelae are uncommon.
Grade 2 IVH. Grade 2 IVH develops when GMH perforates the ependyma and spills into the ventricular system. The ventricles may be dilated, but they are only partly filled with echogenic blood. Blood may pool in the occipital horns. IVH is often isoechoic to choroid plexus, and the two may be confused. However, echogenic material in the frontal horns indicates IVH, as choroid plexus does not extend this far forward. As IVH evolves, the clot becomes hypoechoic and eventually contracts. If chemical ependymitis develops, the ventricular lining becomes echogenic. Hydrocephalus may develop if clot obstructs the foramina of Monro, the cerebral aqueduct, or—less often—the fourth ventricular outflow tracts. In the absence of hydrocephalus, clinical sequelae are unlikely.
Grade 3 IVH. In this case, the hemorrhage fills at least 50% of the ventricle(s). The affected ventricles are dilated, and the hemorrhage forms an echogenic cast of the ventricular contour. As with grade 2 IVH, clot may block CSF pathways and lead to further ventricular enlargement. About 50% of patients with grade 3 IVH will develop cerebral palsy and/or developmental delay.
Additional Differential Diagnoses
Grade 4 periventricular hemorrhage (PVH). Mass effect from GMH or severe ventricular enlargement may lead to blockage of parenchymal draining veins with subsequent venous ischemia. This may progress to arterial hemorrhage and hemorrhagic infarction. With venous ischemia, US shows a large GMH and extensive increased periventricular echogenicity. With hemorrhagic conversion, the hemorrhage appears more masslike. It may lead to cystic encephalomalacia and porencephaly, with resultant cerebral palsy and/or developmental delay. PVH accounts for 5% of intracranial hemorrhage.
Choroid plexus hemorrhage. Full-term infants may develop hemorrhage in their highly vascular choroid plexus. They may also develop thalamic and cerebellar hemorrhage.
Diagnosis
Bilateral germinal matrix hemorrhage (grade 1) with hemorrhage extending into the right lateral ventricle (grade 2) and filling the dilated left lateral ventricle (grade 3).
Pearls
Grade 1 germinal matrix hemorrhage is at the subependymal caudothalamic groove and bulges into the lateral ventricle floor.
With grade 2 IVH, germinal matrix hemorrhage extends into the lateral ventricle, and blood partly fills the ventricle.
Grade 3 intraventricular hemorrhage is complicated by ventricular enlargement, and blood mostly fills and forms a cast of the ventricle.
Grade 4 periventricular hemorrhage develops when germinal matrix hemorrhage or hydrocephalus leads to obstruction of parenchymal veins with subsequent hemorrhagic ischemia.
Suggested Readings
Fritz J, Polansky SM, O’Connor SC. Neonatal neurosonography. Semin Ultrasound CT MR. 2014; 35(4):349–364 Orman G, Benson JE, Kweldam CF, et al. Neonatal head ultrasonography today: a powerful imaging tool! J Neuroimaging. 2015; 25(1):31–55 Riccabona M. Neonatal neurosonography. Eur J Radiol. 2014; 83(9):1495–1506Case 160
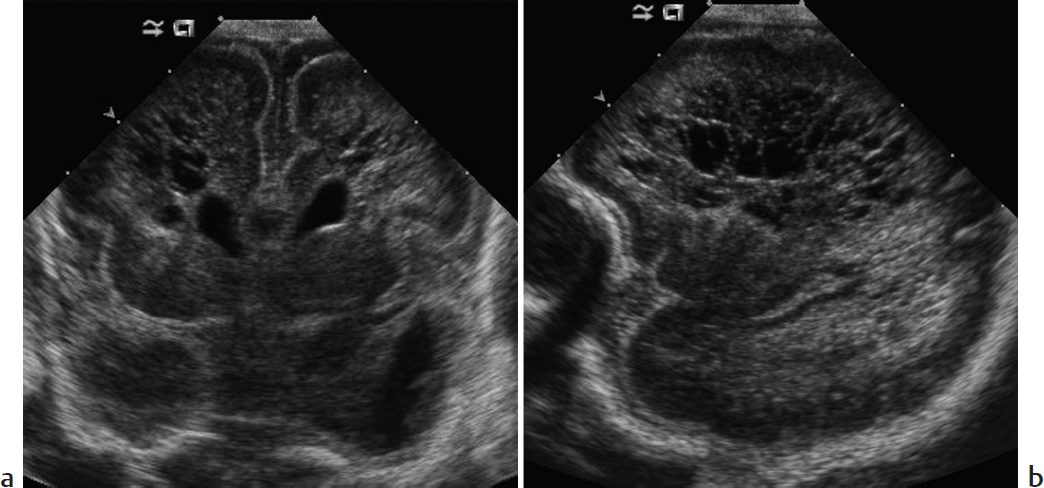
Clinical Presentation
A 4-week-old ex-26-week premature infant with apnea and bradycardia (▶Fig. 160.1).
Key Imaging Finding
Periventricular cysts.
Top Three Differential Diagnoses
Connatal cysts (aka frontal horn cysts, coarctation of the lateral ventricles). These cysts probably represent normal variants that develop if the ependymal lining on apposing sides of the lateral ventricle abuts. They form at or just below the superolateral angle of the frontal horn or, less commonly, adjacent to the anterior body of the lateral ventricle. Most are anterior to the foramina of Monro.
Subependymal cysts. Most subependymal cysts are located below the external angle of the lateral ventricle, posterior to the foramina of Monro. These cystic cavities may be seen in the general population. Some result from prenatal hemorrhage, ischemia, or infection (cytomegalovirus/rubella). Those located at the caudothalamic notch are usually seen in premature infants and represent evolving germinal matrix hemorrhage.
Periventricular leukomalacia (PVL). PVL develops in premature infants who have suffered perinatal hypoxia. Most cases occur in children born at less than 32 weeks EGA and weighing less than 1.5 kg. PVL forms in the periventricular white matter, which is the watershed zone. Most PVL develops in the peritrigonal area and anterolateral to the frontal horns, especially in the centrum semiovale and the optic and acoustic radiations. Screening US may initially demonstrate periventricular increased echogenicity that evolves to periventricular cysts by around age 2 weeks, but US may be normal. At this time, MRI may show periventricular necrosis and cavitation. MRI of toddlers with developmental delay may demonstrate undiagnosed PVL: loss of white matter volume, sulci extending close to enlarged lateral ventricles, and a correspondingly thin corpus callosum. White matter signal is increased on T2 and FLAIR due to gliosis.
Additional Differential Diagnosis
Mucopolysaccharidosis. Deficiency of lysosomal enzymes leads to mucopolysaccharide deposition in many tissues, including in the perivascular spaces of the brain. This may lead to development of cysts in the white matter, basal ganglia, and corpus callosum. In addition, the deep white matter is usually hypodense on CT, hypointense on T1, and hyperintense on T2, perhaps due to accumulation of glycosaminoglycans and gangliosides. Periventricular white matter is most often affected, but all parts of the cerebrum may be involved. Delayed myelination, hydrocephalus, and brain atrophy may be evident as well. Hurler syndrome is the most common mucopolysaccharidosis. Zellweger and oculocerebrorenal syndromes are other leukodystrophies that may develop deep periventricular white matter cysts.
Diagnosis
Periventricular leukomalacia.
Pearls
Connatal cysts usually form at or just below the superolateral angle of the lateral ventricle frontal horn.
Subependymal cysts form posterior to the foramen of Monro, below the external angle of the lateral ventricle.
Periventricular leukomalacia is usually seen in the peritrigonal area or anterolateral to the frontal horns, in the centrum semiovale.
Perivascular mucopolysaccharide deposition may lead to white matter, basal ganglia, and corpus callosum cysts in patients with mucopolysaccharidosis.
Suggested Readings
Epelman M, Daneman A, Blaser SI, et al. Differential diagnosis of intracranial cystic lesions at head US: correlation with CT and MR imaging. Radiographics. 2006; 26(1):173–196 Phelan JA, Lowe LH, Glasier CM. Pediatric neurodegenerative white matter processes: leukodystrophies and beyond. Pediatr Radiol. 2008; 38(7):729–749 Simbrunner J, Riccabona M. Imaging of the neonatal CNS. Eur J Radiol. 2006; 60(2):133–151 Zafeiriou DI, Batzios SP. Brain and spinal MR imaging findings in mucopolysaccharidoses: a review. AJNR Am J Neuroradiol. 2013; 34(1):5–13Case 161
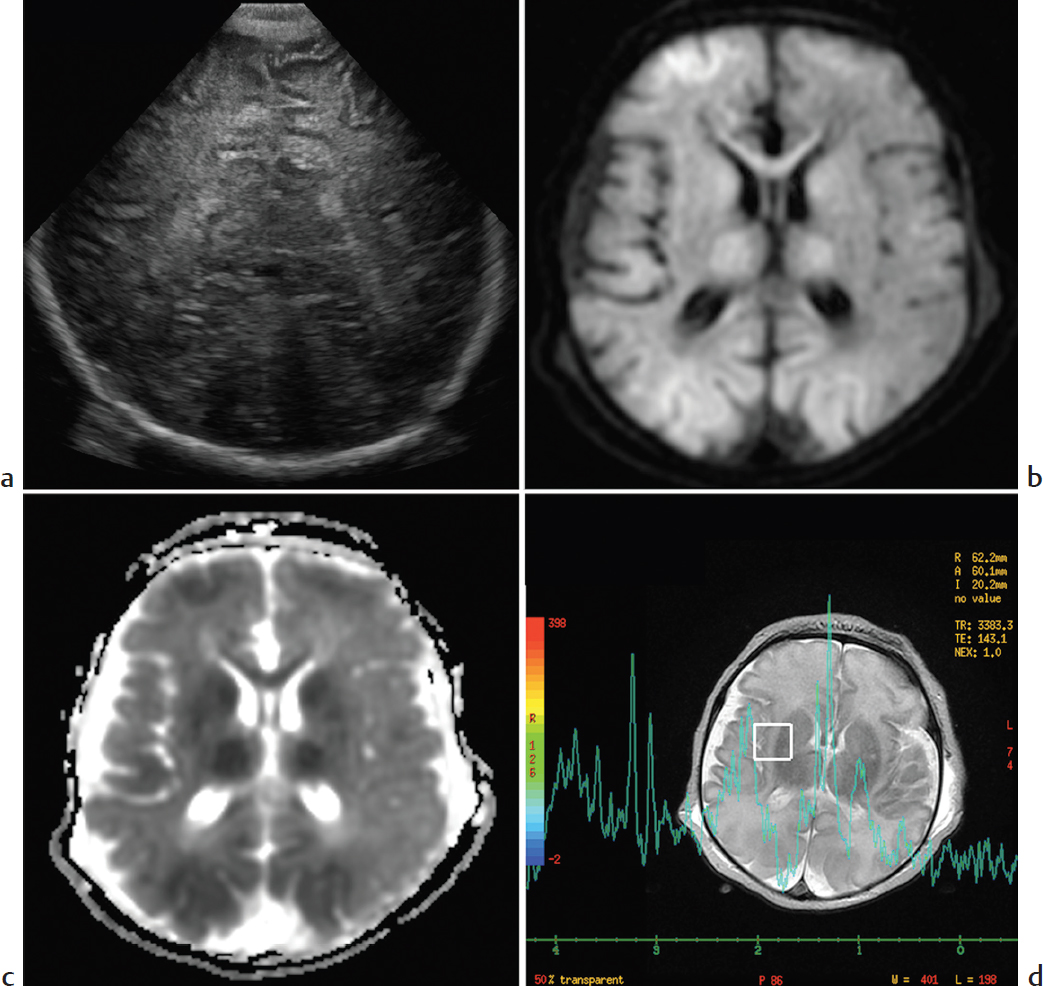
Key Imaging Finding
Restricted diffusion.
Top Three Differential Diagnoses
Diffuse hypoxic-ischemic encephalopathy (HIE). HIE can develop in a variety of settings. In the perinatal period, it often results from hypoperfusion due to failure of autoregulation. In the setting of nonaccidental trauma (NAT), it may result from strangulation or direct trauma to the brainstem respiratory center. Near drowning and other circulatory arrest may cause HIE in older children. Depending on patient age and insult severity, ischemia may be global or limited to the watershed areas and/or basal ganglia. Profound hypotension predominantly damages the most metabolically active and mature regions of the developing brain: in neonates, the brainstem, thalami, basal ganglia, and sensorimotor cortex. Milder hypotension damages the periventricular and deep white matter in premature infants and the watershed portions of the cerebral cortex (and adjacent white matter) in term infants. In older children, the basal ganglia and cerebral cortex are most vulnerable to profound hypotension, whereas milder hypotension causes watershed injury. In its early phase, the cytotoxic edema of HIE is best seen with DWI. DWI offers quantitative information about water motion and the microstructure of tissue. Diffusion is reduced immediately after traumatic, metabolic, or toxic brain injury. Restricted diffusion appears bright, whereas it appears dark on correlative ADC mapping, remaining abnormal for about 6 days. T2-W imaging becomes abnormal within about 24 hours; T1 takes longer. The presence of subdural hematoma, retinal hemorrhages, and suspicious fractures suggests NAT as etiology.
Localized infarction. Stroke is one of the top 10 causes of death in children. Half the cases occur before age 1 year, and of these half occur in neonates. Metabolic abnormalities and coagulopathies cause some strokes, but at least half are idiopathic. Clinical signs and imaging manifestations vary with patient age. Prognosis is generally better for very young children, since the plastic pediatric brain can assume functions of damaged areas. US depicts perinatal strokes as ill-defined areas of moderately increased echogenicity. MRI is more useful, especially in older children. An acute infarct follows vascular territory and appears bright on DWI within hours. T2-W images become positive within 24 hours. The appearance of pediatric stroke resembles that of adults, except in infants whose high brain water content makes identification of stroke more difficult, especially on FLAIR.
Infection. DWI depicts early cell necrosis in the setting of neonatal herpes encephalitis, which may cause multifocal destruction or predominantly affect the temporal lobes, deep gray matter, watershed areas, or occasionally the brainstem and cerebellum. Subtle T2 hyperintensity follows, with subsequent mild meningeal enhancement. Water diffusion is also decreased in brain abscesses, and this helps differentiate these lesions from cystic or necrotic tumors, in which diffusion is not restricted. Late cerebritis also demonstrates restricted water diffusion. Progressive normalization of diffusion indicates treatment is successful. If DWI remains bright, alternative therapies should be considered.
Diagnosis
Hypoxic ischemic encephalopathy in a term newborn.
Pearls
The pattern of diffuse hypoxic ischemic encephalopathy reflects the severity of hypotension and the maturity of the brain.
DWI becomes abnormal within a few hours and normalizes within about 6 days.
The high water content of the infant brain makes it difficult to identify stroke, especially on FLAIR.
Water diffusion is restricted in abscess but not in tumor, making DWI useful for differentiation.
Suggested Readings
Badve CA, Khanna PC, Ishak GE. Neonatal ischemic brain injury: what every radiologist needs to know. Pediatr Radiol. 2012; 42(5):606–619 Parmar H, Ibrahim M. Pediatric intracranial infections. Neuroimaging Clin N Am. 2012; 22(4):707–725 Schwartz ES, Barkovich AJ. Brain and spine injuries in infancy and childhood. In Barkovich AJ, Raybaud C, eds. Pediatric Neuroimaging, 5th ed. Philadelphia, PA: Lippincott Williams & Wilkins; 2012:240–366Case 162
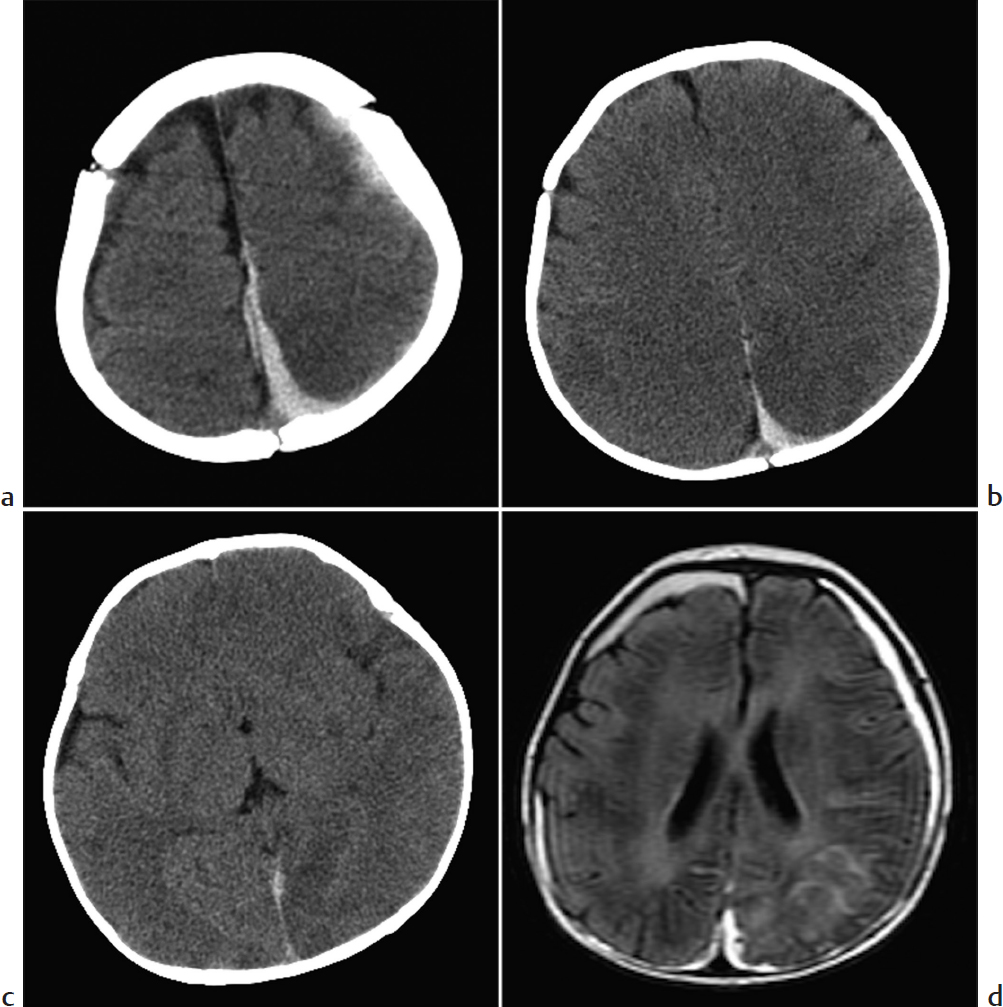
Key Imaging Finding
Subdural hemorrhage in an infant.
Top Three Differential Diagnoses
Spontaneous neonatal subdural hemorrhage (SDH). Asymptomatic SDH is common in the neonatal period. Although usually seen with instrumented vaginal delivery, it may follow any type of delivery. SDH occurs most often in the posterior fossa but may also be found along the posterior falx and over the high convexities, locations that are also common with nonaccidental trauma (NAT)-induced SDH. These SDHs resolve in about 4 weeks and do not progress to chronic SDH. They have no clinical significance.
Posttraumatic SDH. Accidental SDH results from motor vehicle collisions, falls greater than 3 feet, and other significant trauma. History facilitates diagnosis.
Nonaccidental trauma (NAT). Multifocal SDH is a common manifestation of NAT. SDH is most common at the posterior interhemispheric fissure, along the vertex (where there are fragile bridging veins), and in the posterior fossa. Although usually hyperdense on CT, SDH may be heterogeneous if clot forms rapidly, if there are two temporally different hemorrhagic events, or if bleeding develops within a collection of CSF. Disruption of bridging veins may lead to venous infarction, seen in about 10%. Signal intensity of SDH varies on MRI, depending on the stage of evolution.
Additional Differential Diagnoses
Coagulopathy. Hemophilia and von Willebrand disease are the most common coagulation disorders. Children with these diseases may present with bruising and joint swelling. Subdural and retinal hemorrhages resemble findings in children subjected to NAT.
Metabolic disorders. Infants with glutaric aciduria type I may develop both subdural and retinal hemorrhage with minimal trauma. Patients with this autosomal-recessive disease have excessive quantities of glutaric acid due to an enzyme deficiency. Macrocephaly with rapid increase in head size is typical, and infants manifest hypotonia, head lag, and other neurological abnormalities. In addition to enlarged CSF spaces, there is temporal lobe hypoplasia, delayed myelination, and abnormal T2 signal in the globi pallidi. Menkes disease may also demonstrate SDH and other extra-axial fluid collections, along with atrophy and infarctions.
Benign extra-axial fluid of infancy. These CSF collections may mimic resolving SDH but result from increased fluid in the subarachnoid space. The subarachnoid location of fluid is confirmed by visualization of vessels traversing the enlarged extra-axial space, best appreciated at US or MRI. Fluid is also increased in the ventricles and cisterns. Patients are neurologically normal, and there is usually macrocranium and sometimes also a family history of macrocranium. This condition resolves by age 1 year.
Diagnosis
Parafalcine subdural hemorrhage and brain edema due to non-accidental trauma.
Pearls
Spontaneous neonatal subdural hemorrhage mimics nonaccidental trauma–associated subdural hemorrhage, but is often associated with an instrumented vaginal delivery.
Falls from more than 3 feet may result in accidental subdural hemorrhage.
Subdural hemorrhage in the setting of non-accidental trauma is usually along the posterior falx, vertex, and posterior fossa.
Benign extra-axial fluid of infancy is located in the subarachnoid space.
Suggested Readings
Cakmakci H. Essentials of trauma: head and spine. Pediatr Radiol. 2009; 39 Suppl 3:391–405 Girard N, Brunel H, Dory-Lautrec P, Chabrol B. Neuroimaging differential diagnoses to abusive head trauma. Pediatr Radiol. 2016; 46(5):603–614Case 163
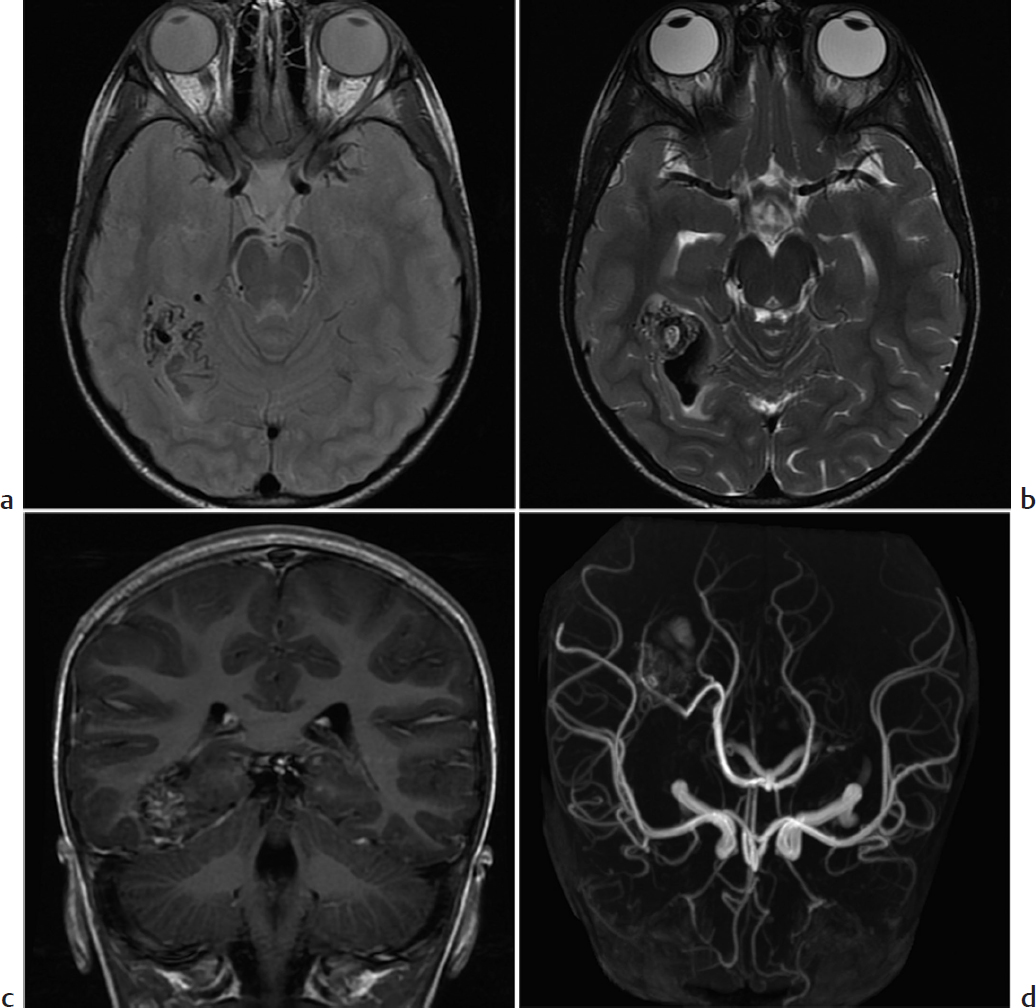
Key Imaging Finding
Parenchymal hemorrhage.
Top Three Differential Diagnoses
Trauma. This is the leading cause of parenchymal hemorrhage in children. History and evidence of trauma usually facilitate diagnosis. CT is often sufficient, but complex or questionable cases may proceed to MRI along with MR arteriography or MR venography. Appearance on T1 and T2 allows dating of parenchymal hematomas.
Vascular malformation (VM). VM is the most common cause of spontaneous hemorrhage in children. Arteriovenous malformations (AVMs) are the most common VM to bleed. AVMs consist of a compact tangle of abnormal, thin-walled vessels (nidus) connecting arteries and veins and allowing rapid arteriovenous shunting. Most patients present with seizures, headache, and/or spontaneous hemorrhage. CT is useful for screening during the first 2 weeks after hemorrhage, but MRI provides much more information. The nidus appears as a serpiginous tangle of flow voids, with feeding arteries and draining veins. Hemosiderin from prior hemorrhage appears dark on T2/T2*. Catheter angiography delineates architecture and precedes possible microsurgery, radiation, or embolization. Developmental venous anomalies (DVAs) are the most common VM, but they never spontaneously bleed and are discovered incidentally. Rarely they may cause headache or seizures and—if the draining vein thromboses—intraparenchymal hemorrhage. DVAs are most common in the frontal lobes and posterior fossa. They consist of radially oriented dilated medullary or subcortical veins that drain into a single venous structure. MRI shows a uniformly enhancing tuft of radially arrayed small vessels. Vein of Galen malformations, another VM, rarely cause hemorrhage.
Hemorrhagic mass. Ependymomas are the most common tumor to hemorrhage, but hemorrhage may also be seen with high-grade gliomas, primitive neuroectodermal tumors (PNETs), hemangioblastomas, and other neoplasms. MR angiography/venography help differentiate between hemorrhage due to tumor versus AVM or other VM.
Additional Differential Diagnoses
Hemorrhagic venous infarction. Almost half of cases of dural venous occlusion (DVO) result in infarction, hemorrhagic in 70%. This causes some neonatal grade IV hemorrhage. US diagnoses DVO in young infants, but older patients require MRI. The affected sinus is enlarged and lacks signal void on fast spin echo (FSE) imaging. It contains clot, with signal intensity varying with thrombus stage (usually T1 bright when acute or subacute). Thrombus may also be seen in deep medullary veins. It is important to evaluate children with DVO for coagulation disorders. In infants, DVO often resolves without neurological sequelae.
Spontaneous intracranial hematoma in a newborn. Most intracranial hematomas in newborns result from birth trauma, asphyxia, clotting disorder, or an underlying AVM. However, increased arterial and venous pressure and impaired autoregulation may cause spontaneous hematoma in otherwise normal infants.
Diagnosis
Arteriovenous malformation.
Pearls
Arteriovenous malformation is the most common cause of spontaneous parenchymal hemorrhage in children.
Ependymomas, high-grade gliomas, PNETs, and hemangioblastomas are prone to hemorrhage.
Venous infarction usually develops in the setting of thrombosis of the dural venous sinus.
Suggested Readings
Burch EA, Orbach DB. Pediatric central nervous system vascular malformations. Pediatr Radiol. 2015; 45 Suppl 3:S463–S472 Epelman M, Daneman A, Blaser SI, et al. Differential diagnosis of intracranial cystic lesions at head US: correlation with CT and MR imaging. Radiographics. 2006; 26(1):173–196Case 164
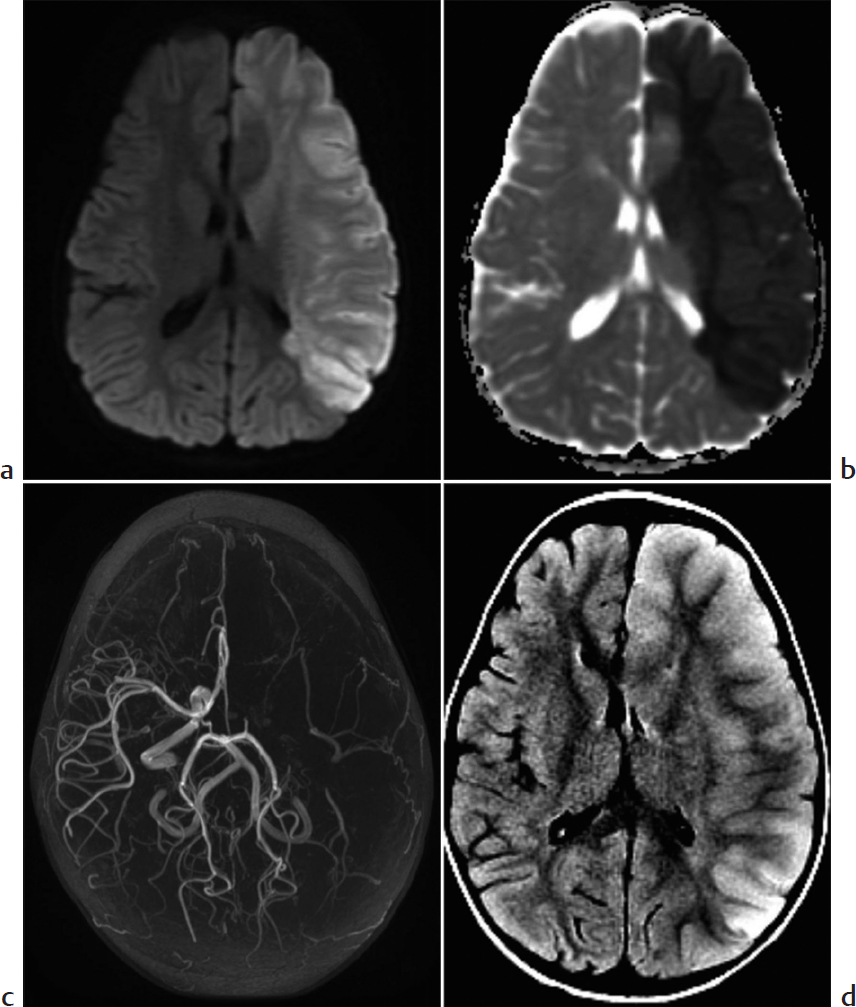
Key Imaging Finding
Cerebral infarct.
Top Three Differential Diagnoses
Sickle cell disease. Cerebral infarcts affect approximately 10% of children with sickle cell disease (an autosomal-recessive disorder of the hemoglobin β gene seen primarily in people of African descent). Red blood cells (RBCs) may become transiently misshapen and stiff, resulting in vascular occlusion or damage to the internal elastic lamina. This leads to ischemia, aneurysm formation, or vasculopathy. Infarcts are most common before age 5 years. They are usually ischemic but may be hemorrhagic in about 25%. In addition to acute infarction, vasculopathy may cause chronic arterial stenosis, often at the distal internal carotid artery or circle of Willis. Chronic occlusion results in development of lenticulostriate collaterals within the basal ganglia, leading to “moyamoya,” or the “puff of smoke” appearance on cerebral angiography. Unenhanced CT in sickle cell patients often shows diffuse atrophy, prior infarcts, and occasionally intraventricular or intraparenchymal hemorrhage. Acute infarction is seen on MRI as diffusion restriction, evidenced by hyperintensity on DWI with corresponding low signal on ADC maps. Hyperintensity on T2 and FLAIR often manifests after 6 hours. Transcranial Doppler US is used to screen sickle cell patients for increased risk of cerebral infarction. Patients then receive prophylactic chronic transfusion therapy or hydroxyurea.
Vasculitis. Vasculitis may also result in cerebral ischemia. The vasculitis may be primary or secondary to a variety of underlying systemic diseases. Though CT is often normal, there may be multifocal hypodensities in the deep gray or subcortical white matter, suggesting prior ischemia or infarction. MRI may show subcortical or deep gray matter FLAIR/T2 hyperintensities, with diffusion restriction if ischemia is acute. Rarely, hemorrhage may be evident on T2* gradient echo (GRE) sequences. Gadolinium-enhanced MRI may show patchy linear parenchymal enhancement. MR angiography is insensitive but may show concentric, smooth stenoses.
Posterior reversible encephalopathy syndrome (PRES), or acute hypertensive encephalopathy. This is a disorder of cerebrovascular autoregulation related to acute or subacute hypertension, drug toxicity (immunosuppressants, chemotherapy), hematologic disease, or renal disease. Endothelial damage and resulting failure of autoregulation disrupt the blood–brain barrier, causing bilateral, symmetrical subcortical vasogenic edema, usually in a parietooccipital distribution—though any region of the brain may be involved. CT may show subtle bilateral, patchy, parietooccipital hypodense foci. MRI shows T2 and FLAIR hyperintensities. PRES rarely demonstrates restricted diffusion, hemorrhage, and patchy enhancement.
Diagnosis
Sickle cell disease–related acute left cerebral hemisphere infarction.
Pearls
Cerebral infarction shows high DWI and low ADC signal; FLAIR hyperintensity appears after 6 hours.
Cerebral infarction affects about 10% of children with sickle cell disease; there may be “moyamoya” or “puff of smoke” appearance due to chronic occlusion and collateralization.
Vasculitis is another common cause of pediatric cerebral infarct.
Posterior reversible encephalopathy syndrome has a bilateral parietooccipital distribution and rarely demonstrates diffusion restriction.
Suggested Readings
Abdel Razek AA, Alvarez H, Bagg S, Refaat S, Castillo M. Imaging spectrum of CNS vasculitis. Radiographics. 2014; 34(4):873–894 Aviv RI, Benseler SM, Silverman ED, et al. MR imaging and angiography of primary CNS vasculitis of childhood. AJNR Am J Neuroradiol. 2006; 27(1):192–199 Covarrubias DJ, Luetmer PH, Campeau NG. Posterior reversible encephalopathy syndrome: prognostic utility of quantitative diffusion-weighted MR images. AJNR Am J Neuroradiol. 2002; 23(6):1038–1048 Ohene-Frempong K, Weiner SJ, Sleeper LA, et al. Cerebrovascular accidents in sickle cell disease: rates and risk factors. Blood. 1998; 91(1):288–294Case 165
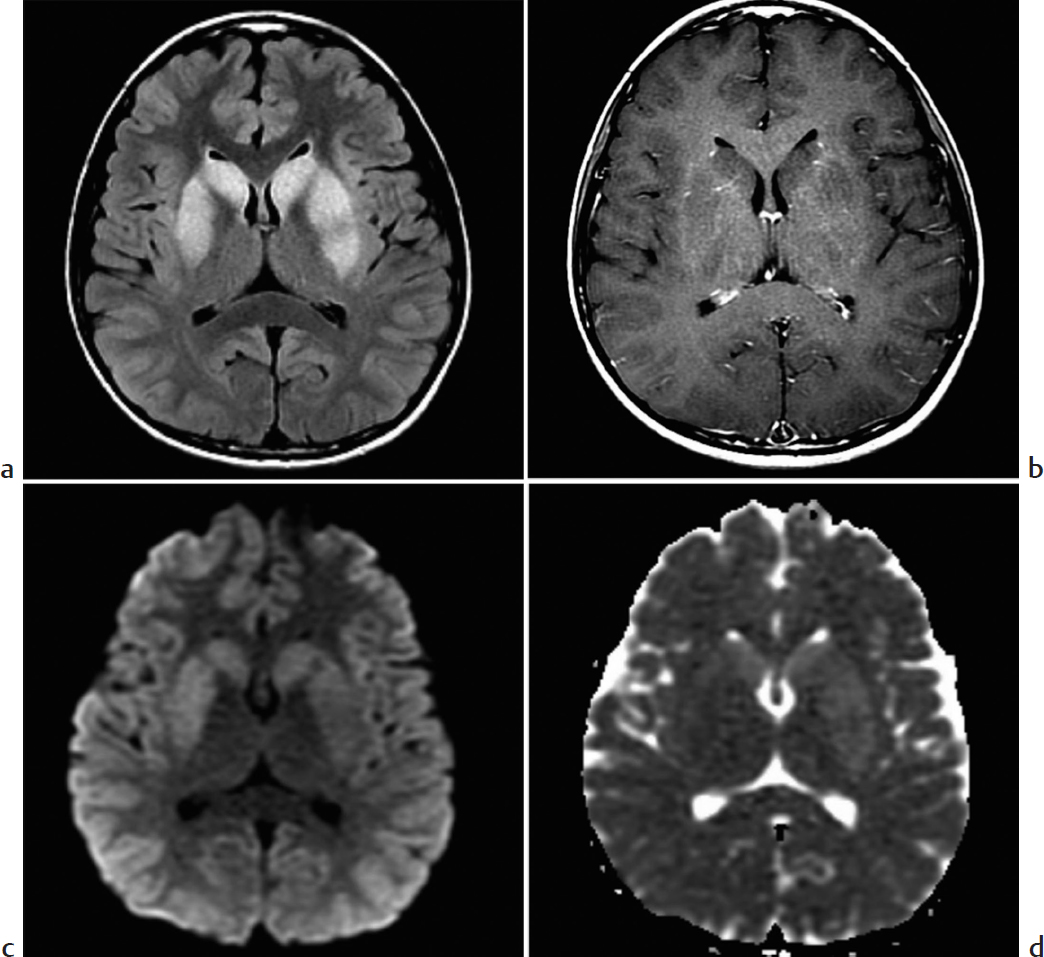
Key Imaging Finding
Bilateral basal ganglia signal abnormality.
Top Three Differential Diagnoses
Hypoxic-ischemic injury. Prolonged asphyxiation or near-drowning events may lead to profound neurological consequences with associated MRI findings. Injury occurs in the metabolically active gray matter, and thus the basal ganglia are preferentially affected, in addition to the cortex. High T2 signal is seen in the basal ganglia and thalami but may also be seen in the periphery, depending on the severity of injury. Sometimes white matter changes develop later in the process, indicating a poor prognosis.
Toxic exposure. Various toxins cause encephalopathy with associated basal ganglia abnormalities. Carbon monoxide poisoning classically causes increased T2 signal in the globi pallidi, sometimes with increased T1 signal due to hemorrhagic necrosis. There are often associated white matter lesions as well. Methanol poisoning causes increased T2 signal in the putamina, likewise with associated hemorrhage.
Thrombosis of deep cerebral veins. Arterial occlusion is uncommon in children and would not be expected to cause bilateral pathology. However, venous occlusion can produce such findings. Venous occlusion may be seen in children with inherited coagulopathies or in those that are critically ill. Both internal cerebral veins are usually affected, associated with dural sinus thrombosis. The veins are hyperdense on CT. The thalami are affected, in addition to the globi pallidi and striatum, which can be a helpful distinguishing feature.
Additional Differential Diagnoses
Inherited metabolic disorder. Several inherited metabolic disorders are characterized by abnormal basal ganglia and may not be suspected if family history is unknown or if the disorder is recessive. Leigh syndrome is an inherited mitochondrial disorder presenting in childhood with hypotonia and neurological regression. It features marked increased signal and swelling of the striatum, globi pallidi, and brainstem, along with characteristic lactate peaks on MR spectroscopy. Wilson disease is an autosomal-recessive disease that leads to copper accumulation; it sometimes presents in childhood. MRI shows high signal in the striatum with predilection for the outer rim of the putamina.
Infectious encephalitis. Several viruses produce encephalitis with deep gray matter involvement. Japanese encephalitis is caused by a flavivirus and is the most common infectious encephalitis in Asia. Asymmetrical, patchy high T2 signal is seen in the basal ganglia and thalami. There may be associated thalamic hemorrhage. Patients are typically febrile. The diagnosis is made by analysis of CSF.
Diagnosis
Hypoxic-ischemic injury.
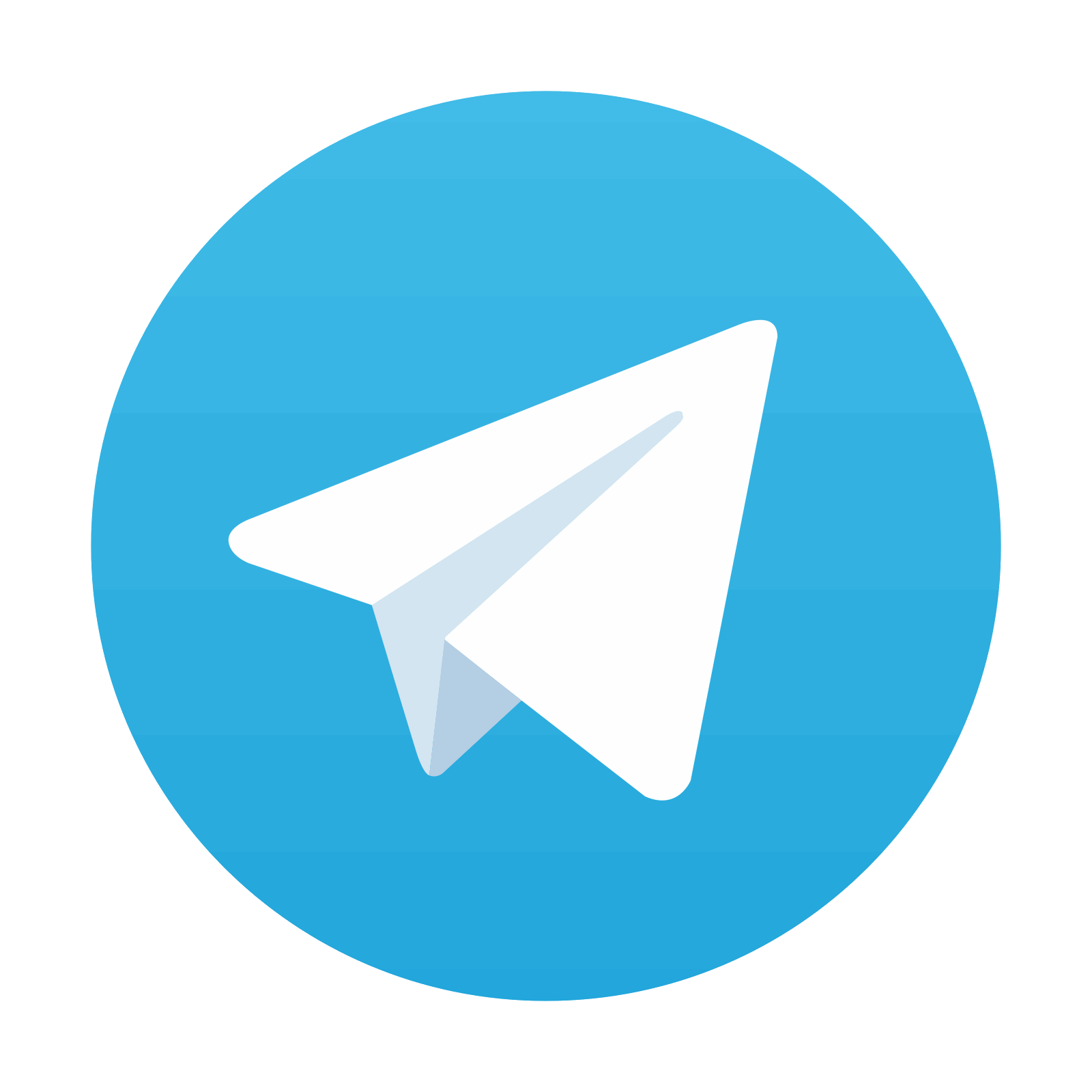
Stay updated, free articles. Join our Telegram channel

Full access? Get Clinical Tree
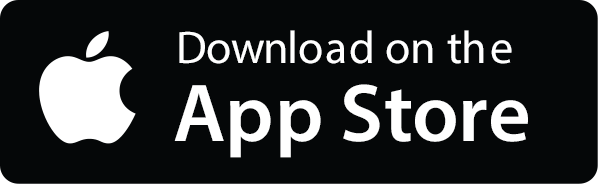
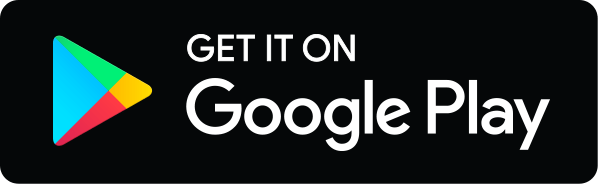
