Chronic venous disorders (CVDs) refer to a wide range of venous disease entities, including mild conditions such as varicose veins and telangiectasias and more severe presentations such as edema and venous ulceration.1 Most patients with CVD have a primary disease of the vein wall with resultant valvular dysfunction in the superficial veins, leading to venous reflux.1 Chronic venous insufficiency (CVI) refers more specifically to a progressive syndrome including symptoms and signs, such as discomfort and skin changes, due to sustained venous hypertension.2 CVI is common, initially presenting as symptomatic venous varicosities that can progress to more severe conditions. The prevalence of CVI in the western world varies from 1 to 40% in females and from 1 to 17% in males, and is higher in industrialized nations.3 Reported estimated prevalence of varicose veins ranges from 1 to 73% in females and 2 to 56% in males.4 Risk factors include prolonged standing or sitting, hormonal influences, pregnancy, female gender, and advanced age.3, 5 In addition to cosmetic complaints, most people with varicose veins do experience symptoms such as lower extremity heaviness, pain, swelling, eczema, pigmentation, hemorrhage, and ulceration.6 Despite the commonness of CVI, its pathogenesis is poorly understood, and may vary among individuals with similar presenting features. The first step in evaluating patients with symptoms suspicious for CVI is to identify the anatomic level of the disease, and then determine the patterns of venous reflux to guide appropriate therapy. Success of potential treatments depends on the understanding of the pathogenesis of varicose veins, which in turn is based on the understanding of venous anatomy. This chapter reviews the relevant anatomy of the superficial and deep venous system of the lower extremity, correlating with the current understanding of the anatomic and pathophysiologic causes of varicose veins. Veins are thin-walled vessels that return deoxygenated blood to the heart and collapse when empty. The innermost layer of the vein wall is the tunica intima, which is a lining of endothelial cells. The middle layer is the tunica media, consisting of bands of smooth muscle; this layer is thin in comparison with arteries of the same diameter. The thick outermost layer, the tunica adventitia or tunica externa, is composed of connective tissue (Chapter ▶ 1, ▶ Fig. 1.2). Compared with the arterial system, there is substantial anatomic variation in the location and branching pattern of veins.7 Normal venous histology demonstrates valves in both superficial and deep leg veins, which permit unidirectional blood flow against the force of gravity back toward the heart.8 Normal vein walls contain bundles of collagen fibers that prevent overdistention and organized elastin fibers that provide elastic recoil.9 Lower extremity venous pressure is determined by the following: (1) the inflow of blood regulated by arterial mechanisms; (2) the ability of the calf muscle and venous valves to return blood to the heart; and (3) the absence of upstream venous obstruction. When these mechanisms fail, lower extremity venous hypertension and ultimately CVI ensue (▶ Fig. 2.1). 10, 11 Fig. 2.1 Schematic demonstration of determinants of lower extremity (LE) venous pressure. LE venous pressure is determined by the inflow and outflow of blood in the lower extremity, and by the hydrostatic pressure of the column of venous blood returning to the heart. The main mechanism to promote venous return occurs in the deep venous system through the action of three muscular pumps: the foot, calf, and thigh. Venous return is accomplished primarily by the pumping mechanism of the calf, with an ejection fraction of 65%, compared to an ejection fraction of 15% in the thigh. 10 The calf muscular pump generates roughly 200 mm Hg when contracting, while venous valves prevent backflow of blood. 11 Accordingly, due to the calf muscular pump, pressures ranging from 20 to 100 mm Hg are accommodated. 11 Reduction in the deep venous pressure during the postcontraction relaxation phase promotes flow from the superficial to the deep system via the perforating veins. One study showed that deficiencies in the calf muscular pump correlate with severity of venous ulceration, 12 though another study indicated that clinical severity of CVI correlated with venous reflux and not calf ejection fraction. 13 Therefore, while the muscle pump of the calf is key in returning blood to the heart from the lower extremity, it is not entirely clear whether deficiencies in pump action play a primary etiologic role in CVI. Understanding the etiology of venous disease requires a thorough understanding of normal venous anatomy. Disease can be localized as either superficial or deep to the muscular fascia. The superficial and deep systems are interconnected through the perforating veins. Proper functional antegrade flow of blood through these vessels is ensured by competent bicuspid valves, associated with an effective muscular pumping mechanism. The valves divide the hydrostatic column of blood into segments, and establish flow from superficial to deep and from caudal to cephalic. 10 For more information and detail on the anatomy of the lower extremity venous system, please refer to Chapter ▶ 1. Approximately 90% of the venous return in the lower extremities occurs through the deep veins, via the muscular pump described earlier. The deep veins include iliac, femoral, popliteal, calf, perforators, and intramuscular veins. Calf deep veins, distinguished by valves every 3 to 5 cm, include the tibial and peroneal veins, as well as soleal and gastrocnemius muscular sinuses. The gastrocnemius veins enter the popliteal veins at the popliteal fossa ( ▶ Fig. 2.2). Fig. 2.2 Venogram of the distal right lower extremity demonstrating normal venous anatomy: popliteal vein (PV), gastrocnemius vein (GV), anterior tibial vein (AT), posterior tibial vein (PT), great saphenous vein (GSV), and Boyd’s (B) and Cockett’s (C) perforating veins. The perforating veins connect the superficial venous system to the deep venous system. The muscle veins of the calf can be considered as a separate group because of their function as calf muscle pump. The deep fascia of the leg invests all of the calf muscles very firmly and restrains outward bulging of the muscles during contraction. During relaxation, the pressure in the emptied deep veins is lower than in the superficial system and blood is drawn into the deep venous system via the perforating veins. 14 The perforating veins are also part of the deep venous system ( ▶ Fig. 2.2). Clinically important perforating veins include the veins of Cockett connecting the posterior tibial veins with the posterior arch vein or the main stem of the great saphenous vein (GSV), and Dodd’s veins which are a group of three to five perforating veins from the GSV to the femoral vein (Chapter ▶ 1, ▶ Fig. 2.8). Isolated segmental reflux in the main trunks may raise the possibility of an incompetent perforating-mediated venous reflux mechanism. Diagnosis of incompetent perforating veins is important for treatment planning and clinical success of the intervention. Incompetent perforating veins may be classified by anatomic location and level. 15 The superficial venous system, located between the subcutaneous tissue and the deep fascia, has two main divisions: the GSV and the small saphenous vein (SSV) (Chapter ▶ 1, ▶ Fig. 1.4, ▶ Fig. 1.5). This system also includes the reticular veins that run parallel to the skin surface that drains the lower extremities’ skin and subcutaneous tissue. 10, 16 Reticular veins are important in the pathogenesis of telangiectasias, but are less important in the context of CVI. 10 The saphenofemoral junction (SFJ) is the junction of the GSV and common femoral vein ( ▶ Fig. 2.3). The GSV proximal valve is located 1 to 3 cm distal to its actual entry point into the common femoral vein. In normal patients, during intermittent Valsalva’s maneuver, some blood may flow back toward the lower extremity, but never beyond the second valve. The main trunk of the GSV usually has at least six valves, 17 and lies in the subcutaneous tissue superficial to the muscular fascia in the saphenous compartment, which consists of a space outlined anteriorly by the saphenous fascia and posteriorly by the muscular fascia. 18 Fig. 2.3 Venogram of the proximal right lower extremity demonstrating normal venous anatomy: common femoral vein (CF), profunda femoris vein (PF), and great saphenous vein (GSV). Saphenofemoral junction: the GSV joins the common femoral vein (CF), 3 to 4 cm inferior and lateral to the pubic tubercle. In the thigh and calf, the GSV runs along the anteromedial aspect of the lower extremity ( ▶ Fig. 2.2). In the calf, the tributaries of the GSV consist of two main trunks: the anterior branch and the posterior arch vein, which arises behind the medial malleolus and joins the GSV distal to the knee. 19 The two major tributaries in the thigh ascend parallel to the GSV—the anterior and posterior accessory saphenous veins—and are located external to the saphenous fascia. 20 The saphenopopliteal junction is the junction of the SSV with the popliteal vein. The SSV usually enters the popliteal vein above the knee joint in a discrete loop from lateral to dorsal direction; however, there is significant variation in the termination of the SSV. 21 The SSV drains the lateral aspect of the foot and heel, but several communicating veins pass medially to join the venous arches at the medial aspect of the ankle. The SSV usually has 7 to 10 spaced valves. 17 A cranial extension of the SSV (vein of Giacomini) ascends posteriorly in the thigh to communicate with the GSV through the posterior thigh circumflex vein. 20 There are several patterns of venous insufficiency ( ▶ Fig. 2.4, ▶ Fig. 2.5, ▶ Fig. 2.6). It is important to understand the pathophysiology of venous insufficiency as these different reflux patterns may require different therapeutic approaches. Incompetence at the SFJ with truncal reflux along the GSV is one of the patterns of reflux seen with incompetent GSV at its entire length or up to the level of the thigh, with dilation of all branches ( ▶ Fig. 2.4). The tributaries can be implicated in mechanisms of venous reflux at the GSV territory, with reflux seen at the SFJ and then into an anterior tributary or reflux in the anterior tributary and then joining the GSV ( ▶ Fig. 2.5). One important pattern of venous reflux is saphenofemoral incompetence and reflux into the posterior accessory GSV connecting with the SSV ( ▶ Fig. 2.6). Fig. 2.4 Schematic illustration of different patterns of venous reflux in the great saphenous vein (GSV): incompetence of saphenofemoral junction and truncal reflux. (a) Truncal reflux along entire GSV (dilated) and its branches. (b) Truncal reflux along GSV in thigh, then following its branches; below knee, the GSV remains nondilated and competent.
2.2 Histology of the Lower Extremity Venous System
2.3 Physiology of the Lower Extremity Venous System
2.3.1 Muscular Venous Pump
2.3.2 Functional Anatomy of the Lower Extremity Venous System
Deep Venous System
Superficial Venous System
Anatomy of the Saphenofemoral Junction and GSV
Anatomy of the Saphenopopliteal Junction and SSV
Identifying the Level of Venous Disease
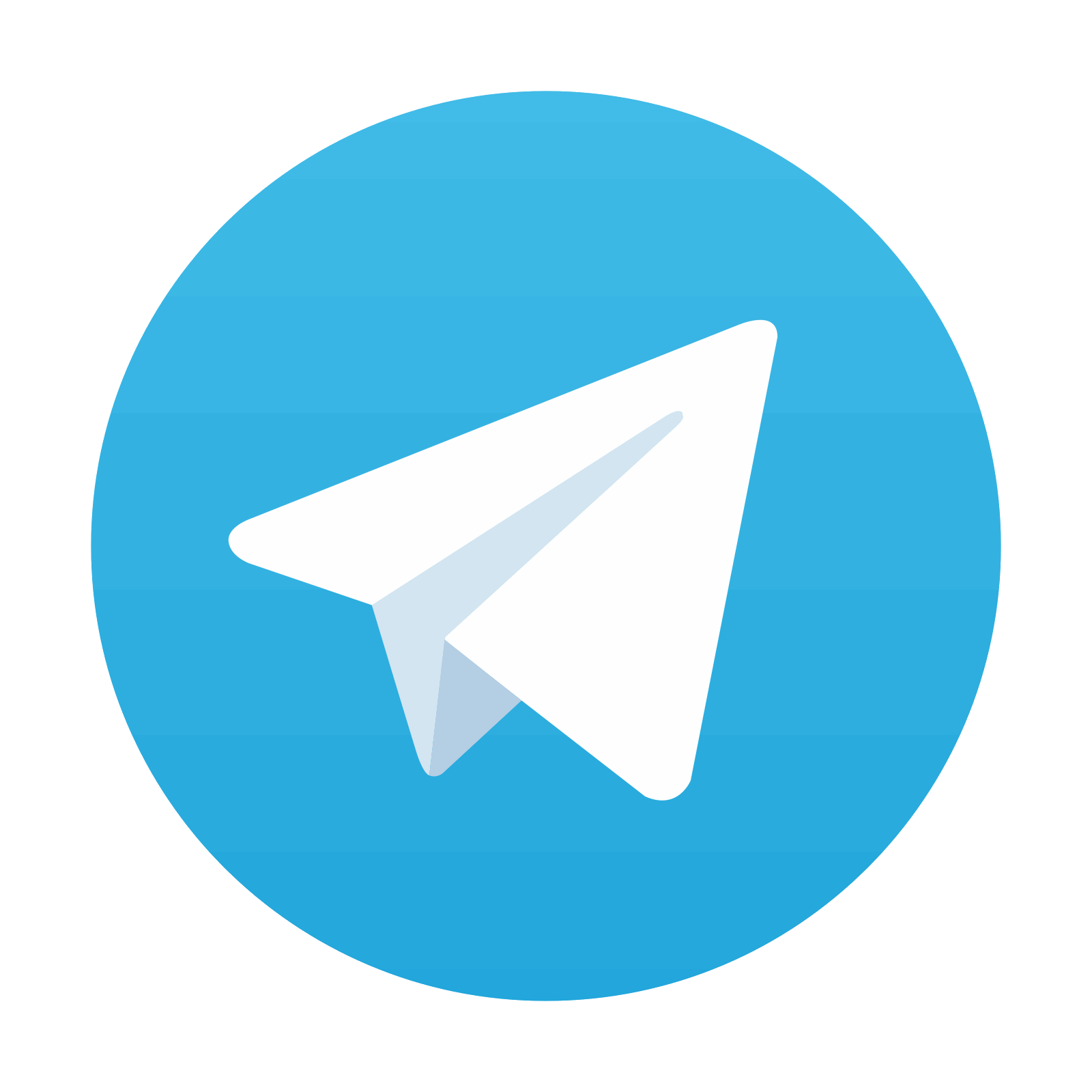
Stay updated, free articles. Join our Telegram channel

Full access? Get Clinical Tree
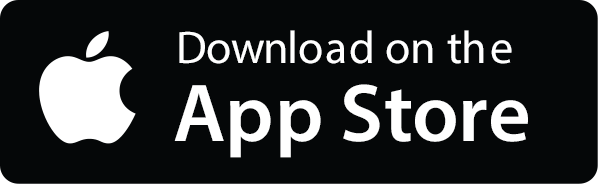
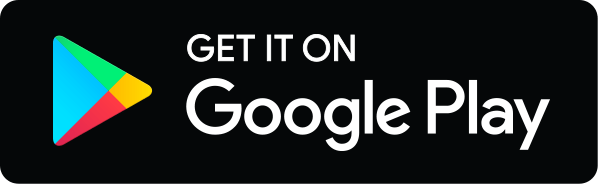