Key Facts
- •
Although clinically useful, bone age measurements may be imperfect due, for example, to methodologic problems and the applicability of standard reference guides to the patient assessed.
- •
Structural scoliosis involves both lateral and rotational deformity of the vertebral column and, by definition, has a fixed component. Postural scoliosis is due to causes extrinsic to the spine itself.
- •
Leg length discrepancy can be quantified using special radiographic techniques. The process of limb lengthening can correct the disparity and its progress can be followed using radiography or ultrasound.
- •
Growth of the femoral head and acetabulum is an interdependent process, Early treatment of developmental dysplasia is necessary to avoid permanent morphologic changes that may predispose to premature osteoarthritis and severe morbidity. Hip sonography is more sensitive than physical exam for mild forms of dysplasia.
- •
Tarsal coalition (fusion of tarsal bones) affects about 1% of the population and is a cause of rigid flat foot deformity. The bridging tissue may be bone, cartilage, or fibrous tissue.
- •
Specific patterns of skeletal injury can identify instances of nonaccidental trauma.
- •
Slipped capital femoral epiphysis occurs in adolescence and is usually diagnosed on radiographs. Computed tomography or magnetic resonance imaging (MRI) may be helpful in diagnosis and in demonstrating complications such as chondrolysis, early fusion of the growth plate, or osteonecrosis.
- •
A lag of several months may occur before radiographs are positive in patients with Legg-Calve-Perthes’ disease. Bone scintigraphy and MRI may show abnormalities earlier in the disease. Several radiographic, scintigraphic, and MRI findings are useful in determining prognosis.
- •
Imaging appearances may help differentiate physiologic bowing from Blount’s disease.
- •
Interpretation of bone densitometry in children is not identical to that in adults. In children, for example, comparison is usually made to age-, gender-, and race-matched controls (the Z score) rather than to peak bone mass (the T score).
In this chapter, we present the imaging indications, workup, and characteristics of several important and commonly encountered congenital and developmental conditions, as well as of the sequelae of chronic trauma that occur during development. Also discussed are the idiopathic abnormalities collectively referred to as the osteochondroses , resulting from disordered ossification in the growing child. Nonaccidental trauma is included given the utmost importance of recognizing this condition, whereas the broad topic of acute accidental skeletal injuries is not included. Finally, we discuss the rapidly emerging field of osteoporosis and bone density measurement in children. Because many of these conditions may first manifest or have sequelae in the adult, there will be some overlap with the adult population and findings mentioned elsewhere in this book.
Emphasis is placed on appropriate indications, the most commonly employed imaging modalities, utilization of advanced imaging techniques, and technique limitations. Imaging findings that can be readily recognized, particularly on radiographs, are emphasized, especially because many of these entities can be diagnosed using this standard modality. Specific mention is made of those findings that affect clinical management. Where possible, algorithms of imaging workup are included to facilitate diagnostic evaluation.
CONGENITAL AND DEVELOPMENTAL ANOMALIES
Assessment of Skeletal Maturity
Determining an individual’s skeletal or bone age, in contrast with the chronological age, is important in understanding whether a child is growing appropriately, a metric commonly used in the fields of pediatric orthopedics, endocrinology, and forensics ( Box 25-1 and Box 25-2 ). Bone age is generally determined by comparing radiographs of the individual with those catalogued from a reference “normal” population. Because skeletal maturity follows a reproducible pattern of changes over time involving appearance of ossification centers, alterations of osseus contours, and timing and closure of the growth plates, skeletal age can be estimated by comparison with standards that have been developed from such reference populations.
ENDOCRINE
Sexual precocity:
Idiopathic
Tumors, brain (hypothalamus, pineal, other):
Tumors, other (liver, sex glands, adrenal):
Premature thelarche
Premature adrenarche
Medication with sex hormones
Thyroid:
Hyperthyroidism
CONGENITAL DISORDERS
Acrodysostosis
Beckwith-Wiedemann syndrome
Cerebral gigantism
Cockayne syndrome
Fibrous dysplasia (McCune-Albright)
Lipodystrophy
Marshall syndrome
Pseudohypoparathyroidism
Weaver syndrome
OTHER CONDITIONS
Large children
Obesity
Idiopathic
ENDOCRINE DISORDERS
Thyroid:
Hypothyroidism
Adrenal:
Addison’s disease
Cushing’s disease
Steroid therapy
Gonadal:
Hypogonadism
Pituitary:
Panhypopituitarism
Growth hormone deficiency
Laron dwarfism
Pituitary giantism
CHROMOSOMAL DISORDERS
Trisomy 21
Trisomy 18
XO
XXXXY
Most other chromosomal disorders
OTHER CONGENITAL CONDITIONS
Most bone dysplasias
Most types of primordial dwarfism (e.g., Silver syndrome)
Most congenital malformation syndromes
OTHER CONDITIONS
Congenital heart disease
Constitutional
Chronic illness (any)
Diabetes mellitus (pediatric)
Inflammatory bowel disease
Intrauterine growth retardation
Legg-Perthes
Malnutrition
Maternal deprivation
Neurogenic disorders
Renal failure
Rickets
The two most commonly utilized methods are the Greulich and Pyle method and the Tanner-Whitehouse method. The Greulich and Pyle method is the most commonly used in the United States. It should be remembered that the determination of skeletal age is an inexact science, with radiographs the mainstay of estimation.
Indications for Imaging to Determine Skeletal Maturation
There are myriad reasons why it may be necessary to determine a child’s skeletal maturation, including for the diagnosis and treatment of abnormalities of growth and maturation (e.g., a child too short or tall for age), approximation of expected adult height in the setting of a growth abnormality, evaluation of endocrinopathies (particularly involving the gonads, pituitary, and thyroid), timing and course of treatment with hormonal therapy, and timing of orthopedic surgical procedures such as correction of limb length discrepancy and scoliosis. From an orthopedic standpoint, the bone age is critical and the chronological age essentially irrelevant for evaluation and management decisions. Bone age determinations at two separate timepoints are recommended when major therapeutic decisions are contemplated.
Technique
The method of Greulich and Pyle involves only a single posteroanterior (PA) radiograph of the left hand and wrist, which is compared with standard radiographs in the atlas.
Bone age is usually determined by comparison of a radiograph of the left hand and wrist with a standard atlas of images. Normal values are within two standard deviations of chronological age.
The atlas images were derived from children of each representative age as based on a prospective study of 1000 healthy Caucasian children of higher socioeconomic status from Cleveland, Ohio, during 1931 to 1942. There are separate male and female standards. The patient’s skeletal age is assigned as that being the closest match. A patient’s skeletal age is generally considered normal if within two standard deviations of his or her chronological age. The time period constituting two standard deviations is a value that also should be included in the report, as determined by charts from the Brush Foundation that are included within the atlas. As systemic factors and illness may more greatly alter maturation of the carpal than phalangeal bones, heavier weighting is given to the appearance of the tubular bones. The Tanner-Whitehouse method also uses radiographs of the hand and wrist. This technique involves evaluation of the extent of ossification and morphology of 20 bones of the hand and wrist with assignment of a developmental stage to each bone, including the distal radius and ulna (2); the phalanges and metacarpals of the first, third, and fifth rays (11); and the carpal bones excluding the pisiform (7). The sum of the carpal scores is averaged with the sum of the remaining scores to give an overall value that is compared with a plot of standards developed from serial hand and wrist radiographs of 2564 British middle-class children obtained from 1945 to 1958.
Discrepancies between skeletal age and actual chronological age, irrespective of abnormalities such as delayed maturation, can come from natural variation of individuals in their progress of skeletal maturity, as well as discrepancy arising from the method itself ( Box 25-3 ). The accuracy of these standardized methods is the subject of much research, with potential sources of error including systematic errors of the method, such as a real difference between the reference standard population and the actual local population being evaluated, and variability in the interpretation of the radiographs by different individuals.
Reference population may be incorrect
Standards are too old
Racial and ethnic differences
Socioeconomic differences
Lack of standards at some time points
Variability in image interpretation
Individual variation
Disorders may affect local maturation (e.g., hyperemia)
In addition to differences between boys and girls, other factors may affect evaluation, including racial, regional, and socioeconomic variables that may make comparison between an individual child and the reference standard population inexact. Moreover, standards developed early in the prior century have been questioned as to their validity today, given changes over time in nutrition, the environment, and socioeconomic factors.
Some studies have suggested that the Tanner-Whitehouse method is slightly closer to true chronological age in normal children than the Greulich and Pyle method, but the former is significantly more laborious to perform with less reproducibility between observers and may not be suitable for a general hospital setting. Studies directly comparing these methods report that the Tanner-Whitehouse method gives a more advanced skeletal age than the Greulich and Pyle method or vice versa, making it important not to compare skeletal ages that are determined by different techniques. An interesting study that evaluated the actual 26 images of the “standard hands” comprising the Greulich and Pyle atlas with the Tanner-Whitehouse method showed that the Greulich and Pyle method consistently indicated a more advanced skeletal age.
In studies evaluating the accuracy of the Greulich and Pyle method in children of different ethnicities, bone age has been shown to vary according to ethnicity and for different age ranges. For example, in one study of U.S. children, the greatest discrepancies were shown in black and Hispanic girls and in Asian and Hispanic boys in late childhood and adolescence, in whom bone ages were shown to possibly exceed the chronological age by as much as 9 to 11.5 months. In preadolescent Asian boys, bone age lagged behind chronological age by 9.5 months. In a study of children born after 1980 in Los Angeles of either European or African descent, prepubertal children of European descent showed delayed skeletal age compared with those of African descent, whereas postpubertal European-American males had delayed maturation compared with African-American males. In another study evaluating black and white children in the late 1980s in the same geographic region from which the Greulich and Pyle standards were derived, the authors concluded that, particularly for black girls, the standards are not applicable. Such results indicate that some caution is prudent in applying these widely used standards based on Caucasian children to other populations, although no comparable nonwhite, race-specific atlases have been published. The Greulich-Pyle method has been characterized by some orthopedists as limited during puberty owing to many factors, such as lack of standards at certain timepoints (e.g., 14.5 years in boys and 11.5 and 12.5 years in girls) and the few and sometimes subtle changes in the hand and wrist during puberty. In the first 2 years of puberty, the Sauvegrain method has been advocated as an alternative technique, based on evaluation of more readily apparent dynamic changes occurring in the elbow. This method is based on evaluation of anteroposterior (AP) and lateral radiographs of the left elbow with assignment of scores based on development of the four ossification centers of the lateral condyle, trochlea, proximal radial epiphysis, and olecranon when compared with a standard diagram. A modified version of the Sauvegrain method has been recently proposed as a reproducible method that compares favorably with and can complement the Greulich and Pyle technique.
In Europe, a sonographic version of the Greulich and Pyle atlas has been proposed as a valid possible alternative for evaluating the ossification centers of the hand and wrist. This method avoids ionizing radiation by using a specialized automated ultrasound device that evaluates the distal radial and ulnar epiphyses. This technique has been reported to provide accurate bone age assessment. Sonographic evaluation of ossification of the iliac crest apophysis also has been described as a possible alternative method. These methods are not in general use, however, and their reproducibility in the non-research setting has not yet been reported.
Scoliosis
Scoliosis, the abnormal lateral curvature of the spine in the coronal plane, can be divided into structural and postural causes. Structural scoliosis involves both lateral and rotational deformity of the vertebral column, may affect the thoracic and/or lumbar spine, and, by definition, has a fixed component. Postural scoliosis is due to causes extrinsic to the spine itself; for example, leg length discrepancy resulting in compensatory curvature, or splinting as in the setting of nerve irritation.
Scoliosis is defined as a lateral curvature of the spine and may be due to structural or postural causes. Structural scoliosis involves both lateral and rotational deformity.
Structural scoliosis is idiopathic in the vast majority of cases, beginning during childhood. In the adolescent age group, idiopathic scoliosis is equal in incidence in girls and boys for minor curves but is nearly 10 times more common in girls than boys for more severe curves that require treatment ( Figure 25-1 ). Idiopathic adolescent scoliosis accounts for the majority of patients with scoliosis and is the focus of this section. Less commonly, structural scoliosis is due to one of the other four, smaller etiologic categories ( Figure 25-2 ) : congenital, as from hemivertebrae or fused ribs ( Figure 25-3 ); developmental, such as that due to skeletal dysplasia; neuromuscular/paralytic, as might be due to myelomeningocele, syringomyelia, and spinal cord tumors ( Figure 25-4 ); or secondary, as from growth asymmetry due to prior trauma, infection, or irradiation ( Figure 25-5 ) ( Box 25-4 ).
Most cases of structural scoliosis are idiopathic and begin during childhood.
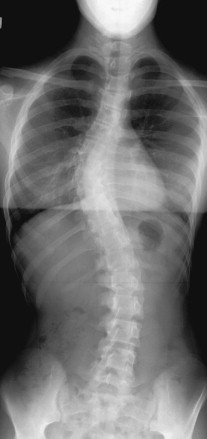
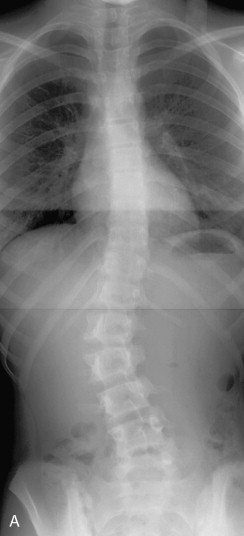
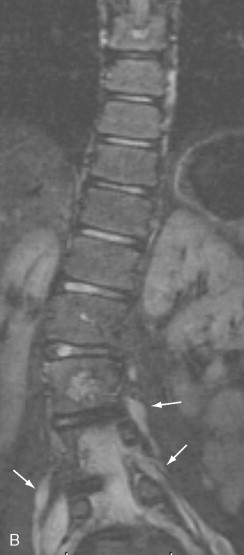
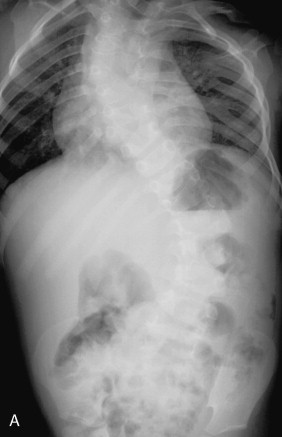
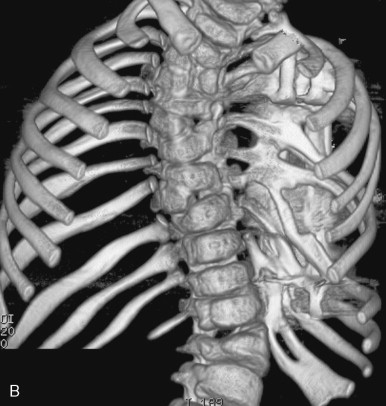
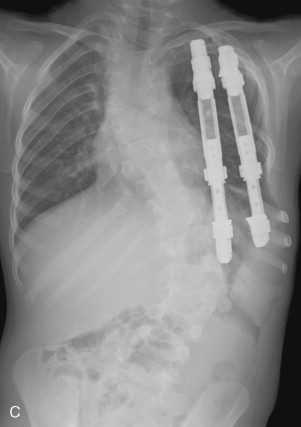
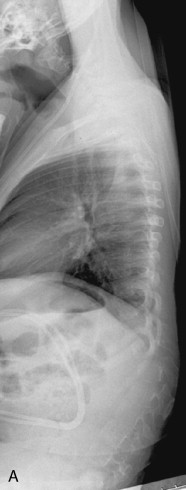
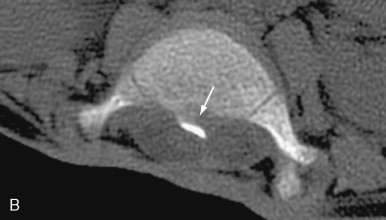
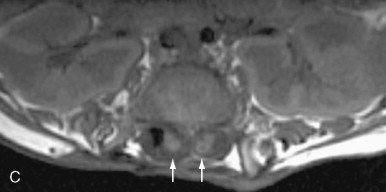
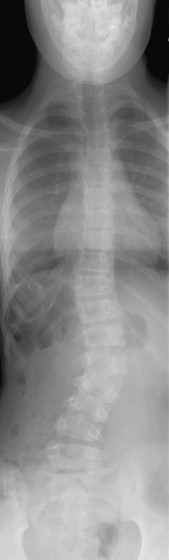
IDIOPATHIC
Infantile (0–2 years):
Resolving
Progressive
Juvenile (3–10 years)
Adolescent (10 years to skeletal maturity)
Adult
NEUROMUSCULAR
Neuropathic:
Upper motor neuron:
Cerebral palsy
Spinocerebellar degeneration:
Friedreich ataxia
Charcot-Marie-Tooth disease
Roussy-Levy syndrome
Spinal cord neoplasm
Syringomyelia (acquired)
Other
Lower motor neuron:
Poliomyelitis and other viral myelitides
Spinal muscular atrophy (SMA):
Werdnig-Hoffman disease (SMA I & II)
Kugelberg-Welander disease (SMA III)
Paralytic myelomeningocele:
Neuropathic arthrogryposis
Dysautonomia (Riley-Day syndrome)
Spinal cord traumatic injury
Other
Myopathic:
Myopathic arthrogryposis
Muscular dystrophy:
Duchenne (pseudohypertrophic)
Becker
Limb-girdle
Fascioscapulohumeral
Myotonic
Fiber type disproportion
Congenital hypotonia
CONGENITAL
Osteopathic vertebral/skeletal anomaly:
Anomalous formation:
Wedge vertebra
Hemivertebra
Anomalous segmentation:
Unilateral bar
Fused ribs only (extraspinal anomaly)
Spondylolisthesis associated
Neuropathic:
Spinal cord anomaly:
Tethered cord
Syringomyelia (congenital)
Diastematomyelia
Other
Spinal dysraphism:
Meningocele
Myelomeningocele
DEVELOPMENTAL
Skeletal dysplasia (intrinsic defect of bone growth):
Diastrophic dysplasia
Spondyloepi(meta)physeal dysplasias
Mucopolysaccharidoses:
Morquio disease
Other heteroglycanoses
Other
Skeletal dysostosis (ectodermal/mesodermal):
Neurofibromatosis
Marfan’s syndrome
Ehlers-Danlos syndromes
Other
SECONDARY/MISCELLANEOUS
Postirradiation
Postinfectious (osteopathic and nonviral)
Posttraumatic (osteopathic)
Postsurgical
Secondary to neoplasm (osteopathic, spinal canal, or beyond)
Other
The most common treatment of adolescent idiopathic scoliosis involves early identification and conservative management to prevent curve progression. Curves of 30 to 40 degrees in skeletally immature patients have a predilection to progress during adulthood at a rate of approximately 1 degree per year, making a curve of 40 to 50 degrees an important threshold to indicate need for arthrodesis and instrumentation.
Indications for Imaging
Imaging studies play a critical role in the diagnosis, surgical planning, and post-treatment follow-up of scoliosis. Radiographs are the mainstay of evaluation, whereas computed tomography (CT), magnetic resonance imaging (MRI), nuclear medicine exams, and ultrasound are employed in specific circumstances. MRI is the predominant and most useful ancillary imaging modality.
Radiography of the spine is indicated in those with alteration in normal alignment on physical exam, for evaluation of curvature progression, follow-up after orthotic or surgical treatment, and in those with a parent or sibling with scoliosis. The patient with scoliosis also may come to clinical attention because of symptoms such as back pain or headache or by way of identification during routine screening programs. In a retrospective series of 2442 patients with idiopathic scoliosis, 23% had back pain at presentation. Scoliosis also may be suspected in high-risk groups, such as patients who have the VATER association ( v ertebral anomalies, a nal atresia, t racheoesophageal fistula, e sophageal atresia, and r enal anomalies/ r adial ray dysplasia). Milder scoliosis may be an incidental finding on imaging studies performed for other reasons ( Box 25-5 ).
Abnormal alignment on physical examination
Evaluating curvature progression
Posttreatment follow-up
Consider in high-risk groups (VATER association)
Osteoarticular Imaging
Radiographs are the initial imaging method of choice for diagnostic confirmation and evaluation and possible operative planning. The initial study includes a frontal radiograph of the entire spine obtained while standing without shoes. A gonadal shield is used to minimize radiation exposure. The exam is performed with the x-ray beam directed from posterior to anterior to minimize radiation dose to the breasts and thyroid. A lateral view may be included on initial evaluation to evaluate associated kyphosis and lordosis, and this view also may detect other important abnormalities including spondylolisthesis or canal widening. Frontal views while the patient bends to each side laterally also are typically included on initial evaluation in order to distinguish the degree to which secondary compensatory curvatures are correctable by bending and also may be obtained at the time of preoperative planning.
Radiation dose to the thyroid and breast tissue is reduced by obtaining PA rather than AP scoliosis radiographs. Gonadal shielding is also employed.
The radiographic study is evaluated to assess degree and type of curvature, as well as whether scoliosis is related to a postural or structural cause. Important abnormalities in the vertebral bodies, ribs, and pelvis can be identified on this study. Although structural scoliosis can be surgically corrected if severe enough, treatment of postural scoliosis is aimed at correction of the underlying etiology. Because the skeletal age is important in determining the likelihood that scoliosis will worsen, its assessment is also important in management. On the scoliosis radiographs, skeletal maturity can be followed by evaluating the degree of ossification of the iliac crest apophysis (Risser’s sign), which begins with calcification of the iliac apophysis at the anterior superior iliac spine, progresses posteromedially, and ultimately terminates with fusion of the apophysis to the iliac crest. A PA radiograph of the left wrist and hand is often also requested for bone age determination.
In the coronal plane, curves are classified as cervical, thoracic, lumbar, thoracolumbar, or double curves. Thoracolumbar curves are defined as those with an apex at T12 or L1. Double curves are two separate curves in different regions. Location and apices of the curves are described. Often there is more than one abnormal curvature of the spine. The more severe curve is often the major curve, whereas curves above and below may be compensatory and shown to be correctable on bending views.
Lateral bending views can demonstrate curves that are correctable.
The Cobb method is universally used to measure the angle of concavity in the frontal plane on radiographs and is based on the principle that the upper and lower vertebra with the greatest tilt in the horizontal plane delimit the curve. The Cobb angle is that formed by a line paralleling the superior end plate of the vertebral body at the top of the curve and a line paralleling the inferior end plate of the vertebra at the bottom of the curve. Often it is easier to measure the angle by drawing additional lines perpendicular to those above, as illustrated in Figure 25-6 . The vertebra at the apex of the curve has the greatest degree of rotation. Rotational deformity of the spine is detected by noting the degree to which the pedicles move away from the edge of the vertebral body and is important for operative planning. The Cobb angle is reported to correlate well with clinical surface measurements using the scoliometer and inclinometer. Normally the spinous process is projected equidistant from each pedicle on the frontal radiograph; rotational deformity can be documented by asymmetry in this appearance.
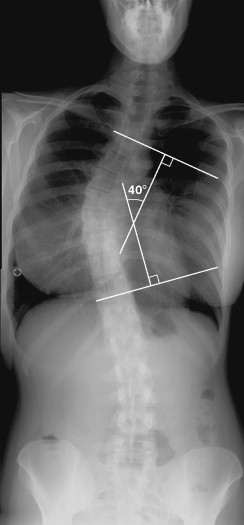
The typical adolescent idiopathic scoliosis is thoracic or thoracolumbar and convex to the right. Leftward thoracic scoliosis should raise suspicion of an underlying specific etiology.
A thoracic scoliosis that is convex to the left should raise the suspicion of an underlying abnormality.
Myopathic scoliosis, such as in the setting of muscular dystrophy or cerebral palsy, often has a wide C shape and is related to neuromuscular imbalance, most typically extending from the upper thoracic to lumbar region in association with an oblique orientation of the pelvis.
Serial radiographs are performed to assess worsening deformity under nonsurgical management. Curves tend to worsen and should be followed particularly during the adolescent growth spurt. Following skeletal maturity, scoliosis tends not to progress if it is less than about 30 degrees, although severe scoliosis can progress throughout adulthood. In the postoperative patient, imaging allows assessment of the degree of scoliotic correction, evaluation of bony fusion, and detection of complications, which include breakage of wires and rods, slippage or fracture of hooks, spondylolysis, and pseudarthrosis. MRI is limited in the postoperative patient, in whom metallic hardware for internal fixation creates susceptibility artifact that obscures the images.
Clinical evaluation plays an important role in deciding when to do advanced imaging studies, most importantly MRI, for evaluation of the neural axis. The use of MRI in the workup of presumed adolescent idiopathic scoliosis is controversial, although many studies support its use in presumed idiopathic scoliosis in the infantile (birth to 3 years) and juvenile (4 to 10 years) subpopulations. Screening in the adolescent population has been reported in a large prospective series to be of low yield as a routine measure. However, many reports advocate MRI in those patients with certain “atypical” clinical findings and symptoms: abnormal neurologic examination (e.g., abnormal reflexes, clonus, weakness, pes cavus), headache and neck pain, back or radicular pain, or rapid progression. Pain as an indicator for MRI is particularly controversial, with some authors noting that it is a common complaint in scoliosis without underlying pathology and negative MRI results are often found when pain is the sole indication for the examination. Of 560 patients in a large series with presumed idiopathic scoliosis and pain, 9% had an underlying pathologic condition.
Radiologic identification of these underlying abnormalities is important in order to identify correctable causes of scoliosis, entities that should be treated before scoliosis is treated, and conditions such as hydrosyringomyelia in which forcible surgical correction may lead to paraplegia. Taken together, these results underscore the importance of thorough history and physical exam, including neurologic exam, in deciding on referral for MRI.
In addition to abnormal clinical findings, abnormal initial radiographic findings that should prompt further evaluation include thinned pedicles, spinal canal widening, unusual curves such as left thoracic or thoracolumbar curves, short-segment scoliosis, rapid progression, and absence of the thoracic apical segment lordosis typically seen with idiopathic scoliosis (i.e., presence of thoracic kyphosis of 20 degrees or greater) ( Box 25-6 ).
CLINICAL FINDINGS
“Idiopathic scoliosis” in infants (birth-3 years) and children (4–10 years)
Atypical clinical findings or symptoms
Abnormal neurologic examination (e.g., abnormal reflexes, clonus, weakness, pes cavus)
Headache and neck pain
Back pain (controversial)
Radicular pain
Rapid progression
RADIOGRAPHIC FINDINGS
Thinned pedicles
Widening of spinal canal
Unusual curves
Rapid progression
Thoracic kyphosis ≥ 20 degrees
In patients with these atypical clinical and radiographic features, MRI findings may include hydrosyringomyelia, spinal cord tumors, tethered cord, Arnold-Chiari malformations, disk protrusion, and dural ectasia. How hydrosyringomyelia and Chiari malformations lead to scoliosis is unknown.
Patients with congenital scoliosis (scoliosis present from birth) also should undergo additional evaluation given a high association with neural axis abnormalities. In this group, diastematomyelia (split spinal cord malformation) is the most frequent intraspinal abnormality (see Figure 25-4 ). MRI has been shown useful in these patients, with other commonly encountered abnormalities including hydrosyringomyelia, Chiari malformation, spinal cord tumors, and tethered cord.
CT with multiplanar reformations and three-dimensional reconstruction is helpful for further evaluation of scoliosis in selected cases, including in the setting of complex congenital osseus deformity (see Figure 25-3 ). Such reformations also can be helpful for operative planning. As CT shows osseus detail to better advantage than MRI, some authors suggest localized CT to further characterize bony abnormalities such as segmentation anomalies. CT is also helpful in identification or confirmation of certain bone tumors that may underlie scoliosis such as osteoid osteoma.
Nuclear medicine examinations are of limited use in evaluating scoliosis. Whole body Tc99m-MDP phosphate bone scanning is sometimes used to localize a bony abnormality such as a tumor or infection and may be helpful in specifying a site of abnormality in the young child who indicates back pain but cannot localize the site. Radiographs or axial imaging techniques can be performed subsequently for dedicated evaluation of the abnormal site. Bone scan does play an important role in evaluating the patient in whom infection related to internal fixation hardware is suspected, cases in which both CT and MRI may be limited by hardware artifact.
In the infant, sonography is useful for evaluation of the spinal canal and for evaluation of open and closed dysraphism that may be associated with scoliosis.
Extraarticular Findings
In the patient with congenital scoliosis, particular search should be made for the VATER association. Congenital scoliosis is commonly associated with genitourinary malformations such as unilateral renal agenesis, and all patients with such congenital spine anomalies should have evaluation of the genitourinary system such as with renal sonography.
Developmental scoliosis can be seen in patients with neurofibromatosis, Marfan’s syndrome, Ehlers-Danlos syndrome, and mucopolysaccharidoses, in whom additional clinical and rheumatologic stigmata may be encountered. Indeed, in patients with neurofibromatosis type 1, scoliosis is the most common skeletal abnormality (see Figure 25-2 ). Table 25-1 lists additional findings that may suggest a particular disease or syndrome associated with scoliosis.
Additional Findings | Diagnosis |
---|---|
Achondroplastic proportions with normal face and early progression of scoliosis | Spondyloepiphyseal dysplasias |
Arachnodactyly, sternum abnormality | Marfan’s syndrome |
Back pain in adolescent with radiodense vertebral pedicle | Osteoid osteomas |
Back pain with other neurologic signs | Osteoblastoma (or canal tumor) |
Café-au-lait spots | Neurofibromatosis 1 (or fibrous dysplasia) |
Cat-cry in an infant | Cri du chat syndrome |
Clubfoot, hitchhiker thumbs, cervical kyphosis | Diastrophic dysplasia |
Dislocated hips and abnormal clenched hand | Trisomy 18 |
Dolichostenomelia, Marfan-like trunk osteoporosis | Homocystinuria |
Facies resembling a bird | Seckel syndrome |
Interpedicle widening, central opaque spicule, and neurologic symptoms | Diastematomyelia |
Kidney surgically absent | Wilms tumor resected, postirradiation changes |
Mandible cysts and basal cell tumors, falx calcification | Gorlin’s syndrome |
Osteoarthrosis out of proportion to age | Ochronosis |
Pneumonia, chronic, in an infant | Riley-Day syndrome |
Short great toes and palpable masses beneath the skin | Myositis ossificans progressiva |
Thoracic paravertebral mass with thinned or spread ribs | Thoracic neuroblastoma |
Trunk relatively long with shortened limbs at birth | Metatropic dysplasia |
Vertebral bodies with H- or U-shaped flattening, limb shortening at birth, death in a few days | Thanatophoric dysplasia |
Leg Length Discrepancy
Pediatric leg length discrepancy is a clinical manifestation of a broad range of underlying etiologies. Leg length discrepancy is due to a structural inequality in which the femur, tibia, or both are physically shorter on one side. This should be contrasted with functional or apparent discrepancies, in which the leg lengths are actually symmetric but appear dissimilar due to other abnormalities, such as a high-riding congenital hip dislocation, pelvic obliquity, scoliosis, or contractures at the hip, knee, ankle, or foot.
Leg length discrepancy may be due to structural changes in an extremity; in some cases, equal leg lengths may appear to be disparate (e.g., due to scoliosis, hip dislocation).
True leg length discrepancy may be congenital or acquired, the latter due to trauma or infection, or to neurologic, neoplastic, dysplastic, or inflammatory etiologies ( Table 25-2 ). Asymmetry may be due to either decreased size or inhibited growth of the short limb or, conversely, to overgrowth of the long limb. For example, whereas a malignant tumor may involve and disrupt the physis to cause lower extremity shortening, a hemangioma can result in increased vascularity and, therefore, may stimulate overgrowth. Additionally, etiologies, in a simplified fashion, can be thought of as those that affect the length of the bone without affecting growth (e.g., a healed diaphyseal fracture with overriding fragments) or those affecting growth and perhaps subsequently length (e.g., osteomyelitis affecting the physeal plate). Although there are numerous etiologies, no clear underlying cause is identifiable in a large percentage of cases, which thus are considered idiopathic. In the case of fracture, the Salter-Harris classification predicts the likelihood of effect on the future growth of the bone.
The Salter-Harris classification of epiphyseal injuries helps predict the affect of a growth plate injury on epiphyseal growth.
Shortening | Overgrowth | |
---|---|---|
Congenital | Femoral deficiencies | Anisomelia (hemihypertrophy and hemiatrophy) |
Congenital coxa vara | ||
Congenital deficiencies of the leg |
| |
| ||
Neurofibromatosis | Vascular malformations | |
Traumatic | Overriding fractures | Healing fractures causing overstimulation |
Epiphyseal fractures causing growth plate damage | ||
Infection | Osteomyelitis causing growth plate damage | Osteomyelitis causing overstimulation |
Septic arthritis | ||
Neurologic | Cerebral palsy | |
Myelodysplasia | ||
Poliomyelitis | ||
Tumors or dysplasias | Malignant tumors | Hemangiomata |
Multiple exostoses | ||
Fibrous dysplasia | ||
Ollier’s disease | ||
Inflammatory | Rheumatoid arthritis Hemophilia | Rheumatoid arthritis or hemophilia causing overstimulation |
Indications for Imaging
The child with leg length discrepancy may come to clinical attention because of an obvious difference in length lengths, gait disorders, lower back or lower extremity pain, or postural problems leading to compensatory scoliosis. Imaging is generally performed in these patients after examination that includes clinical measurement of leg lengths and gait analysis and is performed for diagnostic confirmation, assessment of severity, detection of underlying contributory pathology such as tumors, and operative planning. After surgical treatment, serial radiographs to monitor progression and to detect the complications of lengthening procedures are also an important component of management. A discussion is included here on radiographic evaluation of the commonly used Ilizarov bone-lengthening device.
Osteoarticular Imaging
A few radiographic methods are used for measurement of leg length discrepancy. The teleoradiograph uses a single exposure of both limbs in their entirety onto a long film with the patient standing, a technique limited by magnification. The teleoradiograph is helpful in young children who cannot remain still for three separate exposures and, thus, cause measurement errors. The orthoradiograph avoids magnification error by utilizing three separate exposures, with resultant shorter focus-to-film distance, centered over the hip, knee, and ankle onto a single long film. Similar to the orthoradiograph, the scanogram , the most widely used method, utilizes three separate exposures as well, although the film cassette moves beneath the patient between exposures at each station to produce a smaller composite image ( Figure 25-7 ). A scout view from a CT scanner may be used for evaluating limb length in non–weight-bearing lower extremities.
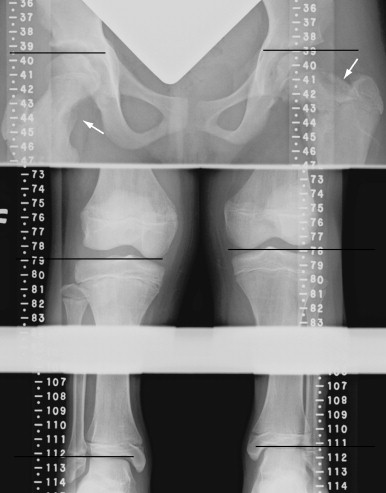
On the scanogram or CT, bony landmarks used for measurement are the top of the femoral head, medial femoral condyle, and ankle mortise. A radiopaque ruler on the image is then used to identify the levels of the joints, and comparison with the contralateral side allows assessment of the disparity in length in centimeters. Validity depends on careful patient positioning and may be misleading if there is angular deformity. In many departments, the exam is performed using digital radiography, exposing the patient to less radiation. On this study, particular abnormalities involving affected physeal plates can be noted, as the four physes of the femur and tibia contribute differently to overall leg length, an important factor in management decisions. Serial examinations are typically performed in order to plot growth patterns over time and are analyzed by standardized methods, as the treatment goal is chosen according to the discrepancy expected at the time of skeletal maturation rather than the present discrepancy. A bone age radiograph of the hand and wrist is also often performed to help predict the discrepancy at maturation in order to plan treatment, as skeletal age correlates more closely with leg growth than with chronological age.
Although radiographic measurements are considered more accurate, discrepancies in lengths by scanogram should correlate closely with clinically measured true leg lengths as determined with a tape measure from the anterosuperior iliac crest to the medial malleolar tip.
The severity of discrepancy is generally more important than the etiology in choosing one of the following treatment strategies. As a generalized guideline, inequalities of up to 2cm result in no functional or clinical adversity and are therefore not treated. Between 2 and 6cm, treatment options include a shoe lift, shortening of the long limb, or arresting growth of the longer limb (epiphysiodesis). From 6 to 20cm, lengthening is performed of the shorter limb. Greater than 20cm, a prosthesis is generally used.
Because length discrepancy is more often due to abnormal shortening of one extremity, methods to shorten the longer leg involve operation on the normal leg as a compensatory measure. Epiphysiodesis, which arrests physeal growth on the normal side, allows the other limb to “catch up,” is a low-morbidity procedure, and is the most frequently performed procedure for limb equalization. It is usually performed at the distal femur, proximal tibia, or both. It can be performed with removal of a small block of bone across the physis, inverting its orientation and reinserting it; by extraperiosteal staples spanning the physis; or by percutaneous techniques, now the favored method. Bone shortening, an additional technique, is generally performed of the femur and involves removal of bone with internal fixation.
In contrast, leg lengthening can be performed on the shorter leg to equalize lengths, the most commonly used method involving partially cutting the bone to be lengthened (corticotomy) and then distracting the ends via an external fixator such as the Orthofix or Ilizarov devices ( Figure 25-8 ). The Orthofix device is a unilateral fixator, while the Ilizarov is a circumferential device. The Ilizarov apparatus is both a strong and adaptable device and benefits from a relatively low rate of complications; avoidance of bone grafting, internal fixation, and multiple operations; and partial to full weight-bearing activity is allowed. The Wagner method of limb lengthening is a more involved three-step operation including open mid-diaphyseal osteotomy and external pin fixation and distraction, interposition of bone graft and application of a sideplate and screws, and then removal of internal hardware after bony maturation.
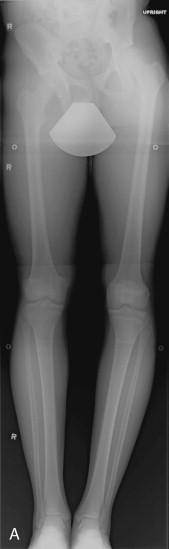
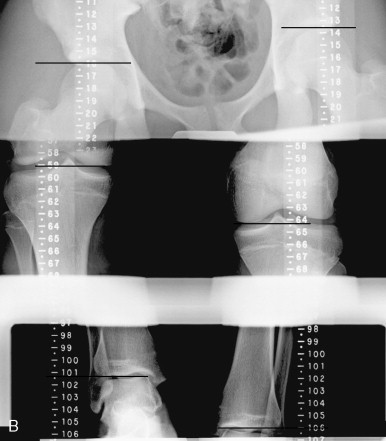
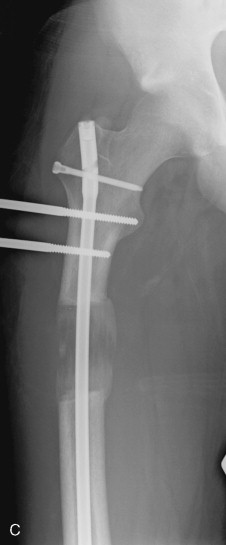
The Ilizarov method is particularly useful for complex deformities and has gained popularity as a method of choice for bone lengthening. It relies on controlled gradual tension-stress over time using an external Ilizarov apparatus, a method of distraction osteogenesis in which new bone is formed between bony surfaces that are pulled apart ( Figure 25-9 ). The Ilizarov apparatus is composed of a circular fixator frame with which distraction and compression forces are achieved by threaded rods. Angular deformities can be corrected by hinge mechanisms. A corticotomy is made with a sleeve of periosteum remaining intact to provide scaffolding onto which new bone can grow. After a latency period of days to allow osteogenesis to commence, distraction by adjustment of the rods is usually performed at 0.25mm four times per day until the desired length is obtained, and then the frame remains in place until there is adequate consolidation of the new bone.
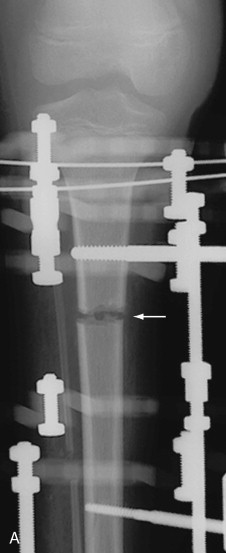
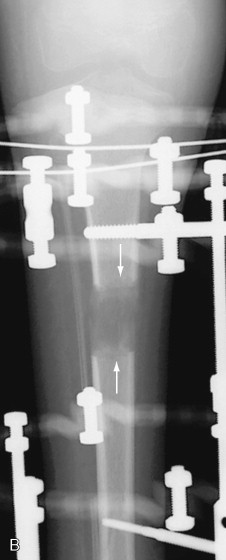
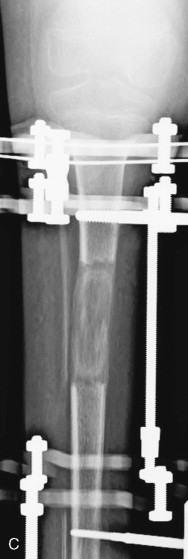
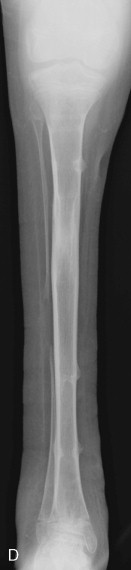
Radiographs are the standard and most useful imaging exam by which the gap of lengthening and the extent of new bone formation are documented. Radiographs are performed at 2- to 4-week intervals after hospital discharge. In the first few weeks of distraction, no new bone is seen radiographically. Thereafter a hazy amorphous opacity develops within the gap, followed by progressively increased density that assumes a longitudinal orientation and, finally, exhibits corticomedullary differentiation.
Radiographs should include the entire bone or joint to assess overall alignment of the osteotomized segments, as well as a clear view of the distraction gap. This may require centering the x-ray beam at this site for accurate measurement. Distraction performed too quickly inhibits bone formation, whereas that performed too slowly can precipitate early consolidation. The radiographs allow the orthopedic surgeon to fine-tune the tempo of distraction. A widening lucency within the gap is a sign of distraction performed too quickly. Ultrasound has been reported to show initial bone growth within the gap earlier than radiographs.
The orthopedic surgeon decides when to remove the device based on the correct length of distraction and the radiographic demonstration of new bone that has progressed to early corticomedullary differentiation. However, no standardized radiographic metrics of adequate bony consolidation have been published.
Complications of bone lengthening procedures that can be demonstrated radiographically include displacement, angular deformity, premature or delayed consolidation, fracture or deformity of the new bone within the gap, hardware fracture, pin loosening or infection (evidenced by abnormal surrounding radiolucency), delayed union or non-union, and reflex sympathetic dystrophy. A sawtooth-like lucency in the center of the new bone may be a sign of either stress fracture or delayed union, with differentiation aided by the presence of pain in the former and by follow-up imaging. Particularly in patients with congenitally short femurs, who have anterior cruciate ligament deficiency and lateral femoral condyle hypoplasia, posterior knee subluxation is a complication. Cystic degeneration within the distraction gap is best demonstrated with ultrasound and may be suspected in the setting of delayed bone formation. Believed due to lymphatic or venous congestion, cystic degeneration may require bone grafting.
Complications that have been reported after hardware removal and that are evaluable on radiographs include deformity and fracture of the callus in cases where new bone formation was not yet mature. Long-term follow-up radiographs may be warranted to evaluate for fractures at the lengthened site, as this segment of bone may be weaker than normal for years.
Other complications of lengthening will be radiographically inapparent and include muscle contracture, arthrofibrosis, cartilage damage, motor palsy, hematoma, nerve or vascular injury, and compartment syndrome. Interestingly, hypertension can develop during lengthening and is of unclear cause, resolving with shortening of the distraction gap.
Developmental Dysplasia of the Hip
Developmental dysplasia of the hip (DDH) is a broad term that includes congenital and developmental abnormalities leading to an abnormal relationship of the femoral head and acetabulum, ranging from mild capsular laxity or instability, to subluxation, to irreducible dislocation. Some forms are only shown on imaging studies as mild malformation of the acetabulum. Although the vast majority of cases are present at birth, DDH may not become apparent until months to years later. In part due to the diversity of the conditions encompassed by the term DDH , differences in definitions, and the fact that mild dysplasia often resolves on its own, the true incidence is elusive and controversial. Involvement is more commonly unilateral than bilateral.
Growth of the femoral head and acetabulum is an interdependent process, so early treatment is necessary to avoid permanent morphologic changes that may predispose to premature osteoarthritis and severe morbidity. Without normal articulation, the acetabulum will become shallow with increased anteversion and may fill with hypertrophy of a fibrofatty tissue in the joint, called pulvinar tissue . Femoral head ossification becomes delayed, and femoral anteversion and coxa valga may result.
Without the normal articulation, the acetabulum will become shallow.
Indications for Imaging
Most cases of DDH are detectable at birth, and the diagnosis is often a clinical one. On newborn screening exams, the femoral head can be manipulated in and out of the acetabulum of the infant with DDH (Barlow and Ortolani maneuvers). Other important clinical clues, generally seen at a later stage, include asymmetry of thigh or buttock folds, apparent shortening of the femur, and limited hip abduction. After the initial postnatal period, clinical detection may be more difficult due to tightening of the hip capsule and muscular contraction.
In the newborn, DDH can be detected by manipulation of the femoral head in and out of the acetabulum of the infant with DDH (Barlow and Ortolani maneuvers). Later clinical clues include asymmetry of thigh or buttock folds, apparent shortening of the femur, and limited hip abduction.
Ultrasound is often performed for diagnostic confirmation and to obtain a baseline measure of severity prior to treatment; it is also helpful in cases of indeterminate clinical findings. Early detection allows less invasive treatment and prevents the need for future surgical correction, all the more important because standard harness treatment for DDH discovered early carries a low rate of complications. Hip sonography is more sensitive than physical exam for mild forms of dysplasia.
Risk factors for DDH include female gender, first-born child, breech position, positive family history, oligohydramnios, and postural molding conditions such as torticollis or foot deformity. DDH is reported to occur in up to 23% of infants of breech presentation. Many of these risk factors appear to be conditions in which in utero positioning is restricted.
Although ultrasound is performed in some European countries such as Switzerland and Germany as part of universal newborn screening, in the United States typically only those with abnormal physical exams or high risk factors are evaluated with imaging. As such, sonography is not recommended by the American Academy of Pediatrics (AAP) as a routine newborn screening exam, as this has not been of proven benefit.
The use and timing of imaging studies is somewhat controversial. In the infant with a normal clinical exam and no risk factors, periodic clinical exam suffices without ultrasound. For a female breech infant, a particularly high-risk group, imaging evaluation is recommended. In the neonate with an abnormal exam, urgent attention including referral to an orthopedic surgeon is warranted. If there is a hip click or equivocal exam but not a positive Barlow or Ortolani sign, these infants should be reexamined in 2 weeks and either referred to an orthopedist if the exam is positive or referred for ultrasound in 2 to 3 weeks or for orthopedic consultation if still inconclusive.
The American College of Radiology regards radiography of both hips in the AP view more appropriate than bilateral ultrasound in patients with clinically suspected DDH who are older than 4 months.
It should be noted that imaging is usually not performed during the first 2 weeks of life due to normal physiologic laxity, since minor evidence of instability detected sonographically most often resolves without treatment. There is no way to differentiate at this early time those who will require treatment from those who will not.
Osteoarticular Imaging Findings
Ultrasonography is the widely accepted method of choice for screening and evaluation of DDH in the newborn for several reasons. First, in infants, the entire proximal femur is composed of cartilage, which is readily visualized by sonography but radiographically imperceptible. Sonography does not use ionizing radiation, and it allows morphometric and dynamic assessment, with direct visualization of the effects of stress maneuvers. Conventional radiographs are used instead once femoral head ossification has occurred, and evaluation can be performed by radiographic reference lines constructed on a frontal view of the pelvis. CT, MRI, and conventional or cross-sectional arthrography are advanced techniques most helpful in preoperative and postsurgical imaging.
Ultrasound is performed in patients younger than 6 months of age and is generally not helpful after 1 year of age due to ossification of the proximal femur. Using a high-frequency linear transducer, a coronal image is obtained of each hip in the supine or lateral position at rest with the ultrasound transducer positioned laterally in the mid-acetabular plane ( Figure 25-10 ). On this view, the alpha angle is measured, described by a line drawn along the iliac wing and a second line that parallels the osseus acetabular roof ( Figure 25-11 ). Normally this angle is 60 degrees or greater in a mature hip, and smaller angles indicate a more shallow osseus acetabulum. Coverage of the femoral head by the acetabulum should be at least 50% in normal cases. The beta angle, less frequently used, is formed by a line drawn along the lateral straight edge of the ilium and the fibrocartilaginous labrum, which should be less than 55 degrees in a mature hip. This angle is an index of cartilaginous acetabular development. In the axial plane with hip flexion, both static and dynamic views are obtained In this plane, subluxation and dislocation are shown to better advantage, and force is applied to the femur similar to the Barlow maneuver while observing the femoral head for abnormal posterior subluxation over the ischium. Dislocation is typically superiorly and laterally.
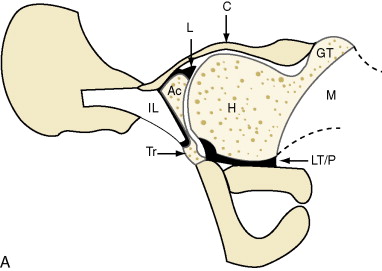
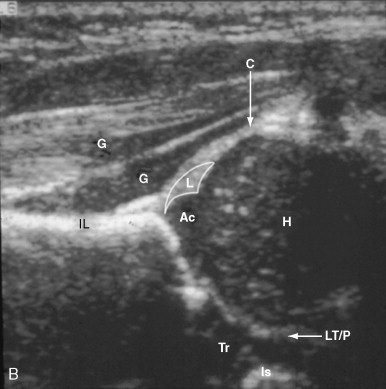
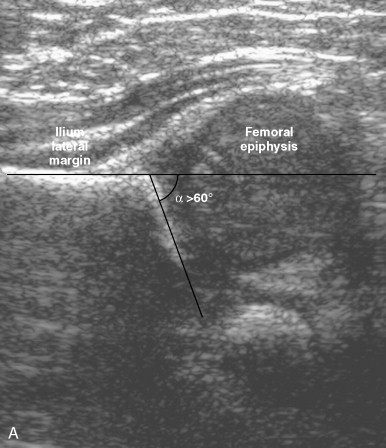
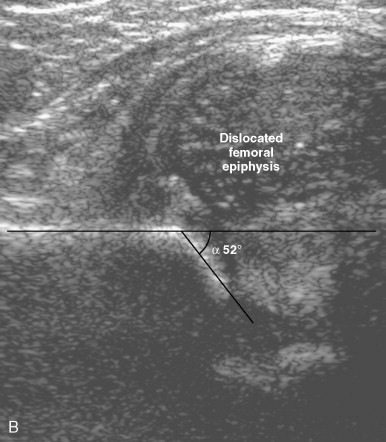
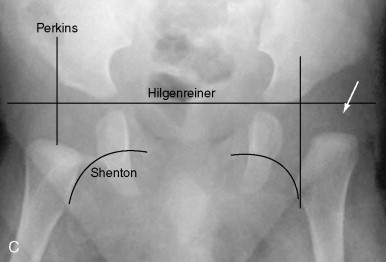
On coronal images, the promontory, that portion of the ilium that begins to deviate medially to form the acetabular roof, is normally sharply angulated. In DDH, it may appear rounded or flattened. In the premature infant, however, this rounded appearance may simply represent immaturity, and a follow-up scan is often helpful.
As normal development of a cup-shaped acetabulum requires the presence of an articulating spherical femoral head, subluxation or dislocation that goes untreated will result in a dysplastic contour of the acetabulum. For this reason, and because it cannot be determined which patients will progress and which will stabilize spontaneously, treatment for all cases of DDH must be initiated early, typically by maintaining the legs in abduction in a Pavlik harness. It is helpful to determine the position of the femoral head while in the harness using ultrasonography. Follow-up sonography can be performed to evaluate progress during harness treatment. Use of ultrasound while under harness treatment appears to maximize the effectiveness of the harness, aiding clinical decisions about adjustments and weaning and alerting the clinician if this treatment modality is failing. Stress maneuvers are not performed during treatment.
The proximal femoral ossification center begins to appear between the fourth and seventh months of life, such that radiography becomes more reliable than ultrasound for evaluation. The typical radiograph of a child diagnosed late will show a shallow acetabulum, decreased femoral head coverage, disruption of Shenton’s line (a smoothly curved line along the femoral neck and inferior cortex of the superior pubic ramus), and delayed appearance of the proximal femoral ossification center. Several radiographic lines are helpful for radiographic diagnosis of DDH ( Figure 25-11 ).
CT is the standard examination for evaluation of the reduced hip when a plaster cast has been placed after closed or open surgical reduction because of late diagnosis or severe disease. Due to concern about gonadal radiation dose, limited CT with low-dose technique can be performed. CT also has been shown useful in evaluating the morphology of the untreated dysplastic acetabulum in preparation for or after surgery, especially when combined with three-dimensional (3D) reconstructions, given the complex structure of the acetabulum. For patients undergoing evaluation for pelvic osteotomy, a procedure to improve the biomechanical forces across the hip joint to minimize development of osteoarthritis, preoperative CT is helpful. CT details the shape, contours, and orientation of the acetabulum and gives information about the fit of the femoral head so that the most appropriate type of osteotomy can be chosen.
In the preoperative setting, MRI provides the additional benefit of illustrating the acetabular labrum, as well as barriers to adequate reduction including an excessively large pulvinar, ligamentum teres thickening, or interposition of the labrum or capsule. Morphology of the acetabulum, femoral head, and femoral and acetabular anteversion also can be characterized.
MRI also has been shown useful to confirm postoperative reduction. In severe cases requiring operative closed or open reduction, a plaster spica cast is placed with the hip in 90 degrees of flexion and controlled abduction. Postoperative imaging is critical to ensure that the hip has not redislocated, and axial gradient echo MRI has been shown useful and avoids the ionizing radiation of CT ( Figure 25-12 ). Radiographs are limited in this setting by overlying plaster material. Ultrasound only can be performed if a window as been cut in the cast, which may weaken it. Osteonecrosis (avascular necrosis, AVN) of the femoral head, a known complication of excessive abduction in a spica cast, is best demonstrated by MRI. To minimize motion artifacts during MRI, some authors suggest performing imaging in the postoperative period while the child is still recovering from anesthesia.
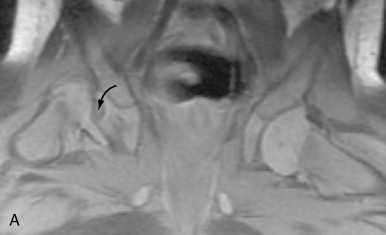
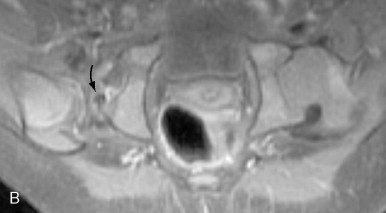
In an adolescent to adult population evaluated for acetabular osteotomy for correction of symptomatic hip dysplasia, a recent study suggests that multidetector CT arthrography may be more sensitive than 3D gradient echo MRI for evaluation of higher-grade articular cartilage lesions with substance loss.
Femoral Anteversion
Femoral anteversion is a developmental deformity in the proximal femur of unknown etiology in which there is increased anterior angulation of the femoral neck with respect to the coronal plane. It is the most common cause of intoeing in children older than 3 years. Normally the femoral neck is directed about 34 to 40 degrees anterior to the femoral shaft at birth, decreasing to 10 to 15 degrees by adolescence. Abnormal femoral anteversion refers to abnormally increased anterior angulation of the proximal femur for the child’s age. With larger angulations, the entire limb becomes medially rotated in order to maintain the femoral head–acetabular relationship (the synonymous term medial femoral torsion ).
In the vast majority of cases, abnormal anteversion will correct by early adolescence without intervention. The only treatment, surgical correction with a femoral derotation osteotomy, is generally considered if the deformity is persistent, severe, and produces cosmetic and functional impairment. Performing osteotomy to prevent subsequent osteoarthritis as an adult is considered controversial, as the causal relationship of abnormal anteversion on development of osteoarthritis is in dispute.
Indications for Imaging
The younger child with abnormal femoral anteversion typically is brought to the clinician because of intoeing or because he or she may trip and fall more often than other children, perceived as clumsiness. Imaging is warranted in the child in whom abnormal anteversion is suspected based on clinical and exam findings and in the postoperative patient after osteotomy.
Anteversion is also associated with several conditions in which the torsion angle may need to be measured for management decisions, including cerebral palsy, poliomyelitis, myelomeningocele, Legg-Calve-Perthes’ disease, developmental dysplasia of the hip, and fracture. The converse situation, retroversion, is seen in slipped capital femoral epiphysis.
Osteoarticular Imaging
In the past, a number of fluoroscopic and radiographic methods were utilized to measure femoral anteversion, including biplane radiography with calculation using trigonometric equations. Currently, CT is the standard method for direct assessment and has been shown to be more accurate and reliable than radiographs. The anteversion angle is measured as the angle that the femoral neck makes with a transverse plane projected through the femoral condyles, viewing the femur on end. Although numerous methods of calculation have been proposed and debated, a commonly used and widely cited method is described here. Selected CT slices are obtained from two different scans, one through the femoral neck and the other through the distal femur at the condyles. Proximal images should include the femoral neck at a level including the upper margin of the greater trochanter, whereas distal images should be measured at a level just below the upper pole of the patella near the distal condyles. The angle of anteversion is that angle formed by a line drawn through the femoral neck and a line through the transcondylar axis. The transcondylar line is that which bisects a line tangential to the anterior margin of the condyles and a line tangential to the posterior margins ( Figure 25-13 ).
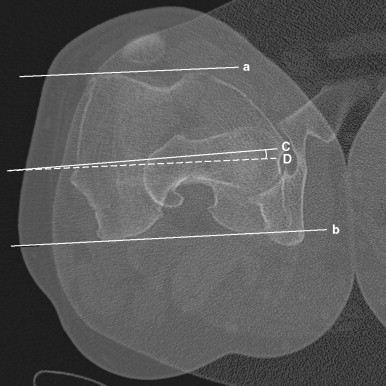
In patients with cerebral palsy, femoral anteversion is a common problem owing to muscular imbalance and delayed weight bearing. Although many have proposed the need for 3D CT reconstruction for evaluation in these patients in order to compensate for difficulties such as in positioning the spastic patient or for anatomic variables such as an increased shaft-neck angle, this topic is debated.
Because MRI and ultrasound do not involve ionizing radiation, they are tempting alternatives for measurement of anteversion. Although MRI has been reported to yield results similar to those of CT in children and adults, it is not used routinely, perhaps because of its limited availability, increased exam time, and increased cost. Direct measurements performed by ultrasound have been reported to be unreliable in measuring anteversion.
Genu Valgum (Knock Knees)
Genu valgum is a common entity that is most often physiologic in nature and part of the normal developmental change in lower extremity alignment that occurs during childhood. The overwhelming majority of cases are physiologic variants that will resolve. Less commonly, focal or systemic pathologic conditions are the culprit, resulting in valgus that is more inclined to progress and require treatment. It is important to understand the normal developmental sequence, which begins with maximal bowing (genu varum) in the newborn period, straightening between 20 and 22 months of age, and then reversal of this configuration into maximum valgus angulation of 10 to 15 degrees at approximately 3 years of age. Then gradual reduction of valgus alignment to the normal “adult” level of 5 to 7 degrees occurs by 6 to 7 years of age. Minimal change in valgus is normally observed during adolescence. By definition, pathologic genu valgum exists when the tibiofemoral angle is more than two standard deviations above normal. Several graphs of normal values have been published.
In normal developmental, the knees show maximal bowing (genu varum) in the newborn period, straightening between 20 and 22 months of age, and reversal into maximum valgus angulation of 10 to 15 degrees at approximately 3 years of age, followed by gradual reduction of valgus to the normal “adult” level of 5 to 7 degrees by 6 to 7 years of age.
Pathologic genu valgum can be idiopathic, posttraumatic (from inadequate reduction or physeal damage and growth arrest), metabolic, neuromuscular, postinfectious (from growth plate disruption or hyperemia resulting in asymmetric growth), or from generalized inherited disorders ( Figure 25-14 ). The presence of unilateral valgus deformity should raise suspicion of underlying tumor, infection, prior fracture at the distal femur or proximal tibia, physeal trauma, prior surgery, metaphyseal dysplasia, fibular hemimelia, or multiple epiphyseal dysplasia.
Unilateral valgus deformity requires investigation for an underlying cause.
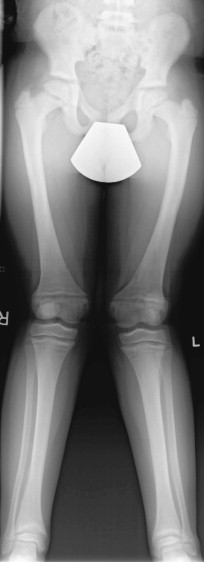
Indications for Imaging
The child with physiologic valgus will typically be less than 7 years of age, be of normal stature, will have symmetric involvement of the lower limbs, and will have a tibiofemoral angle of under 15 degrees. Assessment of genu valgum on clinical exam includes measurement of the tibiofemoral angle and intermalleolar and intercondylar distances, for which tables of normal ranges have been reported. Clinical assessment should include observation of gait, particularly the stance phase in order to detect presence of a medial thrust at the knee, the presence of which indicates that the medial ligamentous and muscular knee restraints have become insufficient to resist deformity just after heel strike. A medial thrust is seen in pathologic but not physiologic states.
In patients with clinical findings compatible with physiologic genu valgum, radiographs are typically not necessary. However, radiographs have been recommended in cases of suspected pathologic genu valgum; for example, when deformity is severe or asymmetric, there are other musculoskeletal abnormalities, there is a positive family history, the tibiofemoral angle is greater than 15 to 20 degrees, or the patient is of short stature ( Figure 25-15 ).
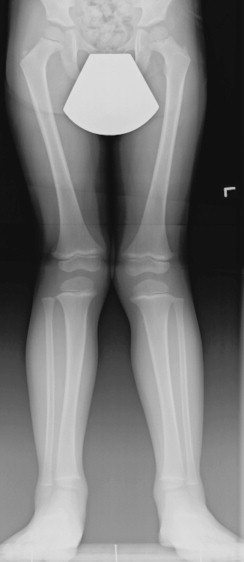
Osteoarticular Imaging
A single long-cassette anteroposterior radiograph during weight bearing is obtained of both lower extremities, including the hips, knees, and ankles. The image is taken with the knees facing forward, disregarding the positioning of the feet in order to get a true understanding of the mechanical axes. A lateral radiograph may be helpful to detect abnormalities in the sagittal plane if such deformity is suspected. The tibiofemoral angle is measured as the angle formed by lines drawn along the long axes of the femoral and tibial shafts. Specific causes of pathologic valgum should be evaluated, such as angular deformity from prior fracture or physeal injury. Patients with idiopathic genu valgum may show flattening of the lateral femoral condyle.
Clubfoot
Clubfoot, or talipes equinovarus, describes a complicated malalignment of the foot and ankle that is composed of equinus of the hindfoot (fixed plantar flexion of the talus), varus deformity of the heel, and varus deformity of the forefoot. There also may be cavus (raised longitudinal arch of the foot) and forefoot adductus, depending on severity.
Clubfoot has been reported to occur two times more commonly in boys than girls and has an overall incidence ranging from 0.6 to 6.8 per 1000, depending on race. Genetics have been reported to play an important role in clubfoot such that the occurrence rate is 17 times higher for first-degree relatives and six times higher for second-degree relatives of affected persons.
There are four main classes of clubfoot. The first class is idiopathic, occurring in otherwise normal children and requiring intensive treatment for resolution. The second class is postural in nature, resolving entirely with manipulation or one or two casts. The third class is neurogenic clubfoot, which occurs in patients with myelomeningocele. The fourth class is that seen in patients with certain syndromes.
Indications for Imaging
Mosca states that the diagnosis of clubfoot can and should be made on clinical grounds alone, with no clear consensus as to the role of radiography in diagnosis and management. Clinical criteria for grading the severity of clubfoot have been described. Radiographs in this setting are limited because there is little ossification in the bones of the newborn foot, and, moreover, there is delayed ossification in the setting of clubfoot. In addition, the ossific nuclei are not located centrally within their cartilage anlagen, making assessment of the true relationships of the structures difficult. Finally, it is arduous to reproducibly position the clubfoot for radiographs, rendering assessment and comparisons difficult. Nevertheless, some authors propose that radiographs may be helpful for confirming correction or to assess residual deformity in patients who have undergone treatment; intraoperatively to confirm adequate correction; postoperatively to confirm maintenance of correction; and when there is recurrence or secondary deformity.
Osteoarticular Imaging
Radiographic technique and patient positioning for evaluation of clubfoot are very important and the subject of extensive descriptions and controversy, with the foot ideally positioned in as close as possible to the maximally corrected appearance. Radiographs should be focused on the hindfoot, as measurements in this region are the main purpose of this view.
On the AP view, in normal individuals, a line drawn along the longitudinal axis of the midtalus should intersect or pass just medial to the first metatarsal, whereas in clubfoot this line will lie more laterally ( Figure 25-16 ). A line drawn along the longitudinal axis of the midcalcaneus should normally overlie the fourth metatarsal head but will fall more laterally in clubfoot. The angle formed between these lines, the talocalcaneal angle, will be abnormally small in clubfoot due to hindfoot varus. On the AP radiograph, features that should be evaluated include abnormal medial displacement of the cuboid on the calcaneus, the talocalcaneal angle (typically < 20 degrees in clubfoot), and the talus–first metatarsal angle (up to 30 degrees valgus normally and mild to severe varus in clubfoot).
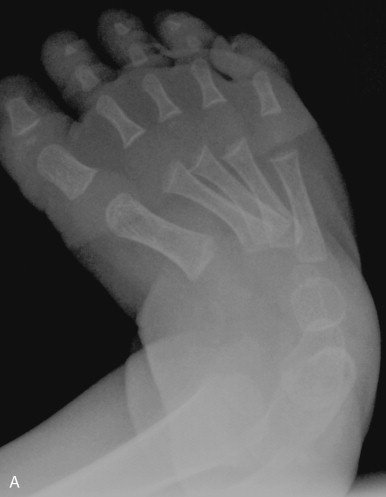
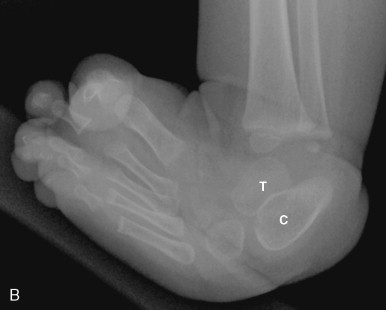
On the lateral radiograph, the normal angle formed by lines drawn through the midtalus and the midaxis of the calcaneus should be acute, whereas in clubfoot these approach parallelism due to the equinus deformity with elevation of the posterior calcaneus ( Figure 25-16 , B ). A line drawn through the midtalus will coincide with a line drawn through the first metatarsal (in children older than 5 years), whereas in clubfoot these will form an obtuse angle. The talocalcaneal (usually < 25 degrees in clubfoot) and talar–first metatarsal angles should be measured. A line drawn along the inferior cortical surface of the calcaneal body normally forms an obtuse angle with a line drawn along the inferior fifth metatarsal cortex, an angle that increases in clubfoot. Plantar flexion of the forefoot on the lateral view may be due to contracted soft tissues or to a triangular shape of the navicular . These angles change with age, and graphs depicting the range of normal values in children have been reported.
Radiographs obtained in long-term follow-up after correction have been reported to show residual abnormalities related to incomplete correction or post-treatment changes, including an inferiorly wedged appearance of the navicular on the lateral view, dorsal displacement of the navicular, flattening of the trochlear surface of the talus, decreased overall length of the talus, shortening of the calcaneus, decreased talocalcaneal angle on both the frontal and lateral views, and lateral wedging of the distal tibial epiphysis. Some of these changes have been postulated as due to sequelae of dorsiflexion during cast correction.
European centers have reported the use of ultrasound for preoperative and postoperative evaluation of clubfoot to evaluate the talonavicular joint, as the navicular can be medially displaced relative to the talar head, and deformity at this joint has important ramifications for the success of treatment.
3D analysis based on CT also has been reported for evaluating the complex intraosseus and interosseus relationships that comprise the clubfoot deformity. The talocalcaneal angle is considered an important parameter for evaluating the corrected clubfoot, and CT with 3D reconstruction has been reported to more accurately reveal the talocalcaneal angle than AP radiographs in this population. Hindfoot correction using this measurement was reported to be misleading by radiographs compared with 3D CT in 75% of patients in one study.
MRI is not routinely performed for evaluation of the idiopathic clubfoot. Thus far, reports utilizing MRI have been primarily in a research setting, contributing to understanding of the pathoanatomic relationships in clubfoot. Deformities that have been reported include a medial deviation of the talar head and neck, a parallel appearance of the talus relative to the calcaneus, and internal rotation of the calcaneus relative to the talus with resultant lateral deviation of the posterior calcaneus. MRI shows the cartilaginous as well as the ossified structures in order to more fully evaluate alignment of hindfoot and midfoot structures. One example of the importance of assessing the cartilage was illustrated by a study showing an abnormally smaller angle due to internal rotation of the cartilaginous talus relative to the bimalleolar plane than in normals, compared with a similar angle when using the osseus talus for measurement. Based on this finding, some authors suggest that preoperative MRI may be helpful when contemplating surgical medial talar de-rotation.
Extraarticular Findings
Clubfoot can be associated with several syndromes, including arthrogryposis, constriction bands (Streeter’s dysplasia), prune belly, tibial hemimelia, Mobius syndrome, Pierre Robin syndrome, diastrophic dwarfism, Opitz’s syndrome, Larsen’s syndrome, and Freeman-Sheldon syndrome.
Although many texts recommend screening hip radiographs in children with idiopathic clubfoot to evaluate for occult but associated developmental dysplasia of the hip, both of which may be related to in utero crowding, the actual rate of hip dysplasia in this population has been reported to be less than 1% in one study.
Tarsal Coalition
Tarsal coalition is abnormal fusion of two tarsal bones. In 90% of cases, fusion occurs between the talus and calcaneus or the calcaneus and the navicular. Coalition is most commonly congenital as the result of failure of segmentation, although it also may be acquired as the sequela of surgery, trauma, infection, or articular disorders. An autosomal dominant inheritance pattern with variable or near-full penetrance has been suggested. It is estimated to affect about 1% of the population and is bilateral in 50% to 60% of cases. The tissue forming the coalition may be osseus, cartilaginous, fibrous, or a combination thereof.
Tarsal coalition (fusion of tarsal bones) may be fibrous, cartilaginous, or osseous and most often is talocalcaneal or calcaneonavicular.
Indications for Imaging
Tarsal coalition generally becomes symptomatic in adolescence when coalitions tend to ossify. Some will present in adulthood. Not uncommonly, the onset of symptoms may coincide with increased stress or trauma. Complaints and findings include vague midtarsal pain, recurrent ankle sprains, worsening pain with activity, eversion of the foot and pain on inversion (peroneal tendon spasm), decreased subtalar movement, and painful flatfoot. These findings and symptoms are not pathognomonic for coalition, and imaging studies are used for diagnosis of coalition and exclusion of differential considerations such as infection, trauma, or arthritis. Finally, coalition may be asymptomatic and discovered incidentally.
Although radiographs are often the initial examination performed in the setting of foot pain, the complicated anatomy of the hindfoot often necessitates advanced imaging techniques, particularly for talocalcaneal coalition. Radiographs alone are more sensitive for detecting calcaneonavicular than talocalcaneal coalition. After radiographs, CT is more commonly used than MRI, although both have high sensitivity and perform better than radiographs alone. The combination of radiographs and CT can identify most coalitions. Bone scan or arthrography is rarely utilized.
Osteoarticular Imaging
Radiography is often the initial evaluation, a study that includes standard AP and lateral weight-bearing views, as well as a 45-degree internal rotation lateral oblique view. If the radiologist is aware of the suspicion of coalition, a special Harris view of the calcaneus is considered the most helpful radiographic view for talocalcaneal fusion, although the technique is somewhat challenging and may require images at multiple degrees of angulation. Several radiographic signs have been described that facilitate identification, although it should be kept in mind that radiographs may appear normal, particularly in cases of talocalcaneal coalition.
In talocalcaneal coalition, abnormal fusion is usually seen at the middle facet of the subtalar joint ( Figure 25-18 ). Much less commonly, fusion may occur at the posterior subtalar joint. Classically described signs, evident on the lateral view, are the C-sign, describing the continuous C-shaped cortical contour of the fused abnormal medial talus with the inferior outline of the sustentaculum tali ( Box 25-7 ), and the “talar beak” sign, an exophytic and often triangular-shaped protuberance at the anterosuperior aspect of the talar head resulting from periosteal elevation with osseus repair due to abnormal subtalar joint motion. Although the C-sign is helpful when present and has a reported sensitivity of 40% to 98%, a higher rate of false negative exams has been reported in patients younger than 12 years and in those with posterior rather than middle subtalar fusion. Moreover, the C-sign has been reported to be possibly a specific (although insensitive) marker of flatfoot deformity and may be neither specific nor sensitive for subtalar coalition itself.
