Fig. 32.1
(a) Classic lateral portal film outlining the posterior fossa boost for a patient with medulloblastoma (lower): composite craniospinal, posterior fossa, and primary site dose distribution for a standard-risk patient with medulloblastoma treated on the St. Jude SJMB96 protocol
This study included patients with a variety of embryonal tumors and presentations including average and high-risk medulloblastoma, supratentorial PNET, and atypical teratoid rhabdoid tumor. All patients received similar post-irradiation high-dose chemotherapy with peripheral stem cell support and all patients received postoperative craniospinal irradiation that was uniquely risk adapted. For example, patients classified as average-risk (no evidence of metastases, residual tumor <1.5 cm2), regardless of embryonal tumor type or location, received 23.4 Gy craniospinal irradiation, 36 Gy posterior fossa irradiation (infratentorial tumors only), and primary site irradiation using an anatomically confined 2 cm clinical target volume margin surrounding the postoperative tumor bed and residual tumor and 0.3–0.5 cm planning target volume margin. Patients with high-risk tumors received 36–39.6 Gy craniospinal irradiation and primary site irradiation using a similarly defined clinical and planning target volume margins. This small and step-wise reduction in primary site targeting did not affect the rate or patterns of failure for patients with average-risk medulloblastoma. The 3-year event-free survival was reported at 78 ± 6 %. Although the gross tumor volume was significantly smaller than the anatomic posterior fossa in these patients, with the additional 2 cm clinical target volume margin and 0.3–0.5 cm planning target volume margin, the final target approached, and in some cases exceeded, the anatomic posterior fossa for these same patients. The volumetric details from this study provided some insight into the effect of the actual postoperative primary site volume, location, and targeting guidelines on our ability to further reduce the volume of irradiation after the craniospinal component. Despite the small reduction in the volume of irradiation, the decline in IQ for patients with average-risk medulloblastoma, ages 3–7 years, was significantly improved to approximately –2.4 points per year. Indeed, the psychology data from this study forms the largest data set for patients treated with craniospinal irradiation for medulloblastoma and provides data from which parents and caregivers may estimate the effects of craniospinal irradiation. In summary, eliminating posterior fossa irradiation and treating the primary site with a limited margin after craniospinal irradiation does improve cognitive outcomes for patients with medulloblastoma.
The next step taken by the St. Jude consortium and separately by the Children’s Oncology Group was further dose and volume reduction. The recently completed St. Jude trial known as SJMB03 (clinicaltrials.gov, NCT00085202) includes identical risk-based craniospinal dosing and post-irradiation chemotherapy as the SJMB96 trial with newer targeting guidelines for the primary site boost. The clinical target volume margin is an anatomically confined margin of 1 cm surrounding the postoperative tumor bed and residual tumor. The planning target volume margins remain 0.3–0.5 cm. The Children’s Oncology Group is conducting a medulloblastoma trial (ACNS 0331, clinicaltrials.gov, NCT00085735) for patients 3–8 years of age and 8–21 years of age (Fig. 32.2). The younger children are enrolled on an ambitious four-arm trial with two steps of randomization. Postoperatively patients are randomized to 23.4 Gy craniospinal irradiation with concurrent vincristine versus 18 Gy craniospinal irradiation and boost treatment of the anatomic posterior fossa to 5.4 Gy (total 23.4 Gy posterior fossa) and concurrent vincristine. Patients are further randomized to primary site treatment that may include 55.8 Gy to the conventional posterior fossa volume or 55.8 Gy conformal primary site irradiation using a 1.5 cm clinical target volume margin. All patients receive post-irradiation chemotherapy consisting of cisplatin, CCNU, cyclophosphamide, and vincristine every six weeks for a total of nine cycles. The chemotherapy regimen was designed based on the equivocal results from the A9961 intergroup study that compared platinum, CCNU, and vincristine to cyclophosphamide, cisplatin, and vincristine. The older patients all receive 23.4 Gy craniospinal irradiation and concurrent vincristine followed by a randomization to 55.8 Gy to the conventional posterior fossa volume or 55.8 Gy conformal treatment of the primary site using a 1.5 cm clinical target volume margin. They will then receive post-irradiation chemotherapy identical to the younger patients.
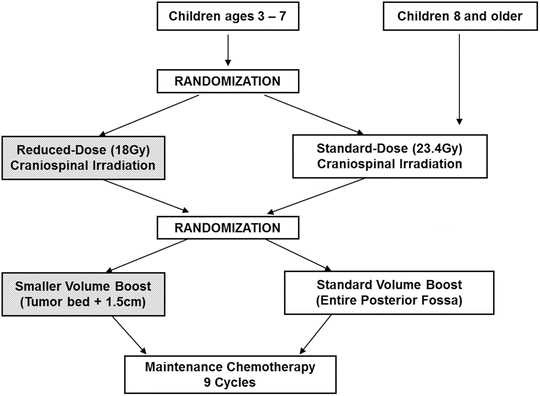
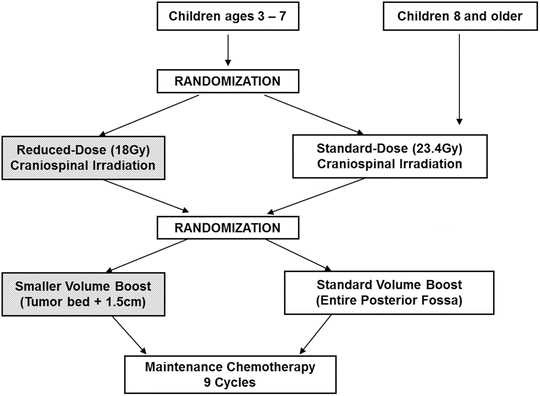
Fig. 32.2
Schema from the COG standard risk medulloblastoma trial ACNS0331 (clinicaltrials.gov, NCT00085735)
A number of important questions should be answered after completion of the Children’s Oncology Group study in late 2013:
In the context of conventional chemotherapy is it possible to eliminate irradiation of the entire anatomic posterior fossa?
Will 18 Gy craniospinal irradiation prove to be as effective (similar disease control) as 23.4 Gy craniospinal irradiation?
Are the volumetric differences between the different treatment arms sufficiently large to detect a difference in cognitive or audiometric outcomes?
Figure 32.3 shows a graphical representation of the dose distribution for the total brain volume that would be expected for a typical patient planned and treated using conformal radiation therapy on the Children’s Oncology Group trial. There are several regions of these curves that are notable moving from lowest to highest doses. The total brain is expected to receive the entire craniospinal dose as prescribed, 18 or 23.4 Gy. The dose to the total brain falls steeply and the curves separate according to those who receive conventional posterior fossa irradiation (parallel opposed portals in the example) or those who receive conformal treatment of the primary site—who tend to have an increase in the volume that receives the intermediate doses. The curves come together at the point where 30 % of the total brain receives approximately 40 Gy, and for the remainder of the high dose region, the curves diverge based on conventional targeting and treatment of the posterior fossa versus conformal treatment of the primary site. These curves demonstrate the volumetric effects of craniospinal dose reduction, the volumetric effects of conformal irradiation, and finally volumetric effects of the reduction in the targeted volume from the anatomic posterior fossa to the primary site with the prescribed clinical target volume margin of 1.5 cm. Based on the improvement shown for the SJMB96 trial over conventional radiation therapy, one would anticipate an improved outcome for those treated with 18 Gy craniospinal irradiation and perhaps those treated on the other arms.
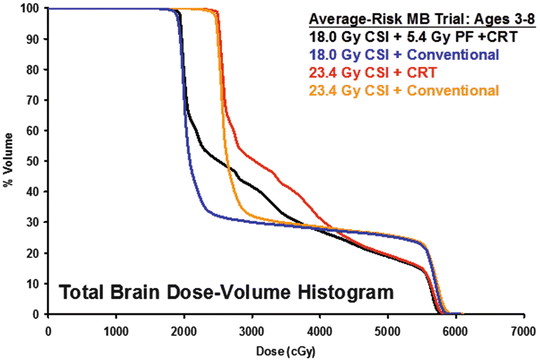
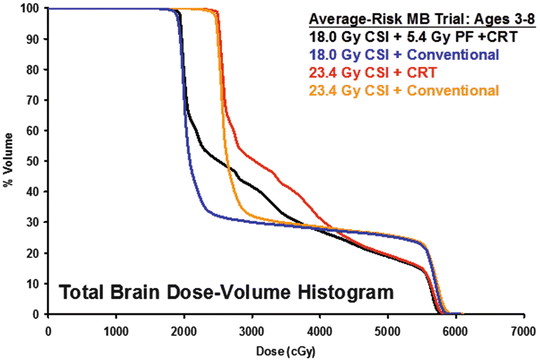
Fig. 32.3
Graphical representation of the whole-brain dose distribution for representative cases planned for treatment on each of the four arms of the COG standard-risk medulloblastoma trial ACNS0331 (clinicaltrials.gov, NCT00085735)
Emerging data strengthen the association between the distribution of dose to regional volumes of normal brain and decline in cognitive function in several domains. The negative impact on IQ and academic test scores associated with increasing mean dose was shown for the entire brain volume, supratentorial brain volume, left and right temporal lobes, and left hippocampus (IQ, math, and reading scores) [10]. Figure 32.4 shows example IQ scores modeled based on SJMB96 data. Proton therapy is an excellent measure to reduce extra-CNS and CNS dose in these patients: Photon dose volume data associated with a decline in IQ are graphically inferior to proton dose volume data for similar patients [11].
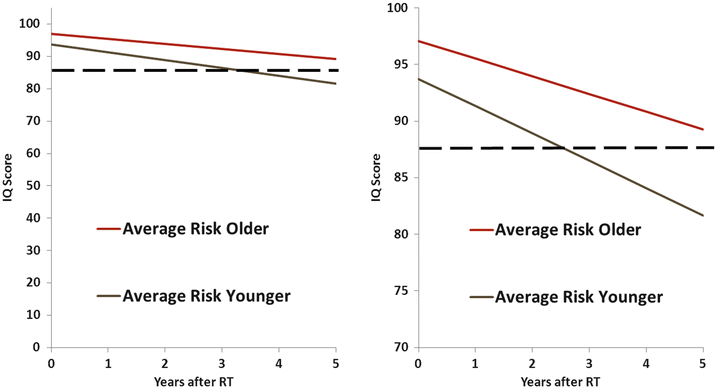
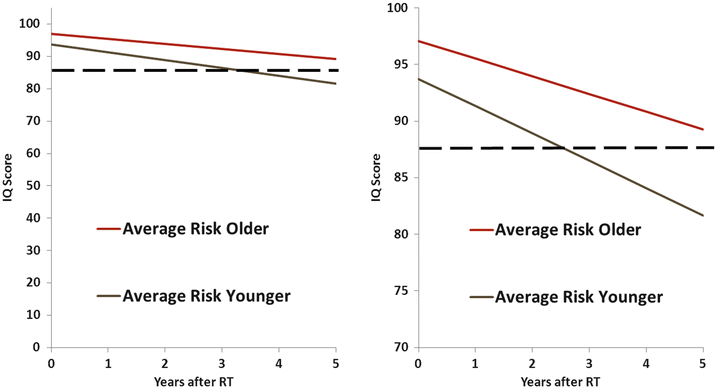
Fig. 32.4
Modeled IQ scores in children with medulloblastoma treated with craniospinal irradiation on the SJMB96 trial (clinicaltrials.gov, NCT00003211)
Early data from a prospective proton therapy trial for patients with medulloblastoma with a median follow-up of 3.2 years demonstrates a 3-year progression-free survival of 83 % and 76 %, respectively, for standard- and high-risk children [12]. Fifty-one children underwent neurocognitive evaluations at baseline and 31 received follow-up testing. Full scale, verbal, and performance IQ evaluations were not significantly different, but there was a decline in processing speed. Long-term neurocognitive outcome data is awaited to be able to compare proton data with that of modern photon data for which we have longer follow-up.
Neurovascular Effects
An important and devastating side effect of radiation therapy is CNS necrosis. Among 148 patients who received 23.4 Gy craniospinal irradiation and 88 patients who received more than 36 Gy craniospinal irradiation, the cumulative incidence of CNS necrosis at 5 years was 3.7 % (SD, 1.3 %) [13]. Those patients for whom CNS necrosis was observed had the largest infratentorial volumes receiving dose in excess of 50, 52, and 54 Gy, providing new limits of tolerance for children with medulloblastoma and other CNS embryonal tumors treated with aggressive surgery, craniospinal irradiation, and intensive chemotherapy.
Endocrine Effects
Baseline and prospective endocrine evaluations were performed for children on the risk-adapted SJMB96 trial described above. The 4-year cumulative incidence of growth hormone deficiency, thyroid-stimulating hormone (TSH) deficiency, adrenocorticotropic hormone (ACTH) deficiency, and primary hypothyroidism was 93 ± 4 %, 23 ± 8 %, 38 ± 6 %, and 65 ± 7 %, respectively [14]. Radiation dosimetry to the hypothalamic-pituitary axis was associated only with the development of TSH deficiency; the 4-year cumulative incidence was 44 ± 19 % and 11 ± 8 % for those receiving a median dose to the hypothalamus of <42 Gy compared to ≥42 Gy, respectively.
Treatment for Young Children
Reintroducing radiation therapy to very young children under the age of 3 years with embryonal tumors has meant omitting craniospinal irradiation from their treatment regimen. The obvious advantages of CSI elimination, combined with conformal radiation therapy (CRT) of the posterior fossa and/or primary site, are apparent. The Children’s Oncology Group Study P9934 demonstrated the advantage of the addition of CRT to postoperative chemotherapy when compared postoperative chemotherapy alone for children with nonmetastatic medulloblastoma between the ages of 8 and 36 months [15]. Seventy-four children on this trial underwent maximal surgical resection followed by four cycles of induction chemotherapy (cyclophosphamide, vincristine, cisplatin, and etoposide) followed by age and response-adjusted CRT to the posterior fossa (18 or 23.4 Gy) and tumor bed (cumulative 50.4 or 54 Gy) and maintenance chemotherapy (four alternating cycles of cyclophosphamide and vincristine followed by oral etoposide). The 4-year EFS and OS was 50 ± 6 % and 69 ± 5.5 %, respectively, which compared favorably to results from Pediatric Oncology Group (POG) 9233 trial which included postoperative multiagent chemotherapy alone and resulted in EFS and OS of 25 ± 5.5 % and 46 ± 6 %, respectively. It is important to note that the P9934 study included more patients with a gross total resection and desmoplastic or nodular histology, which are known to be good prognostic factors compared to POG 9233. Of note there was no significant or consistent decline in either cognitive or motor function that could be attributed to the chemotherapy or CRT. The majority of children did exhibit a significant delay in development at baseline, most likely due to the tumor itself.
The current trial sponsored by the Children’s Oncology Group (ACNS0334, clinicaltrials.gov, NCT00336024) for children with high-risk primitive neuroectodermal tumors randomizes children under the age of 3 years who have undergone maximal surgical resection to the addition of methotrexate to an intensive induction chemotherapy regimen of vincristine, etoposide, cyclophosphamide, and cisplatin. The induction regimen is followed by second surgery if less than a complete response or consolidation chemotherapy with stem cell rescue. Radiotherapy is not a part of this clinical trial but age, risk, and response-adapted guidelines are suggested after the completion of consolidation. CSI is avoided for patients without metastatic disease and the target volume includes the tumor bed with a 1 cm clinical target volume margin with a total dose of 50 Gy (45 Gy < 24 month) for complete response or 54 Gy (50 Gy < 24 month) for partial response or stable disease. Patients presenting with metastatic disease are recommended to receive reduced dose CSI of 18 Gy followed by the same tumor bed boost as above.
Primary Brain Tumors That Require Focal Irradiation
The ICRU-50 Report Guidelines published in 1993 [16] and more recently the ICRU-62 [17] Report Guidelines published in 1999 have been taken to heart by those involved in the design and conduct of brain tumor trials for children. The definitions of the gross tumor volume (GTV), clinical target volume (CTV), and planning target volume (PTV) follow closely those of the ICRU with one notable exception. The GTV includes the tumor bed in addition to the residual tumor. In the ICRU-50 report, the tumor bed is not meant as part of the GTV and the CTV margin is the margin surrounding the GTV in consideration of microscopic extension. Because margins are not assessed for brain tumors, all margins of the tumor bed are considered to be at risk and likely to harbor microscopic disease with extension into surrounding normal tissues. Until the day when neurosurgeons biopsy the operative cavity and the pathologists determine margins or the neuroradiologists have the means to identify subclinical microscopic disease, the margin of the tumor bed will be considered to be the basic volume at risk and defined as the GTV.
The governing principals of target volume definitions and beam’s eye view treatment planning, whether forward or inverse, are similar in children and adults. After the target volume definition, the next step in the planning process is the definition of normal tissue volumes or so-called critical structures. These volumes take on additional meaning in the treatment of younger patients where there is an incentive to minimize dose to normal tissues and spare these patients from the late effects of radiation on the central nervous system. For children destined to receive central nervous system irradiation, planners should have at minimum, dose volume data for the entire brain, supratentorial brain or temporal lobes, hypothalamic-pituitary unit, cochleae, optic chiasm, cervical spinal cord, and brainstem. These patients are at risk for radiation-related CNS effects including abnormalities in neurovascular function affecting hearing, vision, and gross neurologic function, cognitive effects involving learning, memory, attention, behavior, academic achievement, and global IQ, endocrine function affecting growth hormone secretion, thyroid hormone secretion, ACTH secretion, and the gonadotropins. Patients who receive hypothalamic irradiation are at risk for hypothalamic obesity. Patients treated with large volume cranial, superficial cranial, or craniospinal irradiation are at risk for abnormalities in growth and development including deformity of the calvarium, facial bones, and spine [18].
The true value of past, present, and future 3D treatment trials will be the correlation of 3D radiation dosimetry with functional outcomes. Three-dimension radiation planning has greatly improved our understanding of radiation dose distributions in tumor and normal tissues. The most practical way for those involved in treatment planning to view dose volume data is with the 2D dose volume histogram (DVH), which is a reduction of the 3D dose volume information based on the assumption that all elements of the brain volume in question are functionally similar. In other words, the dose-volume histogram lacks spatial information. The cumulative DVH graphs the percent volume of a normal tissue structure receiving a specified dose in a cumulative manner. While this is helpful to the planner, the most important graph is the differential DVH, which represents the percent volume of the normal tissue structure (y-axis) receiving the given dose on the x-axis. Integrating these data one can determine the distribution or burden of radiation therapy to the normal tissue volume in question and when using advanced modeling procedures, one may integrate dose over specified intervals and treat these data as clinical variables in a multivariate analysis. Most modeling procedures have used specific doses chosen along the dose volume curves and entered these values into one of the many models meant to determine the probability of a specific effect.
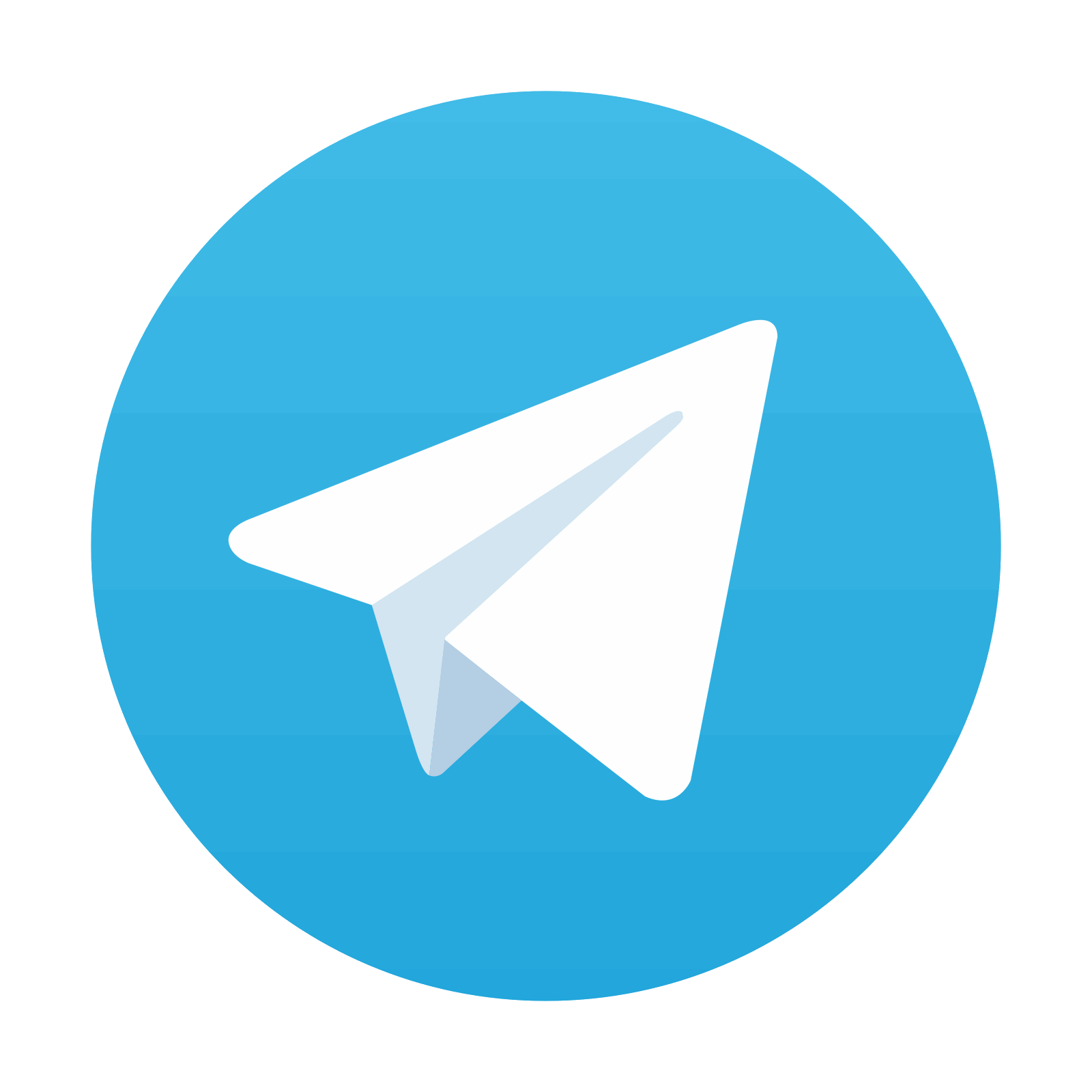
Stay updated, free articles. Join our Telegram channel

Full access? Get Clinical Tree
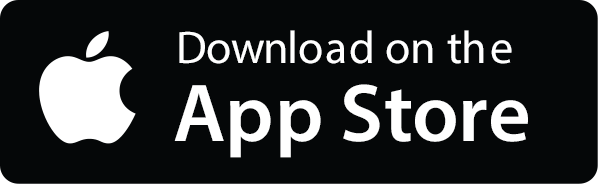
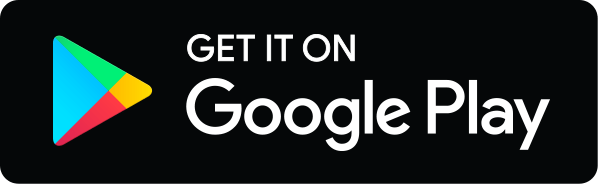