Percutaneous Intervention for Deep Venous Thrombosis
Anticoagulation for deep vein thrombosis (DVT) has been the standard of care for the past 40 years. The rationale is to prevent thrombus propagation, decrease the rate of recurrent DVT, and reduce the risk of pulmonary emboli. Despite the efficacy in preventing new thrombus formation, anticoagulation does nto enzymatically remove existing thrombus. The ability for the vein to restore blood flow depends on the endogenous fibrinolytic capacity of the involved vessel. In thrombosis of the larger veins, the fibrinolytic process is limited, and it is rare that the vein will spontaneously recanalize with normalization of flow. In most cases, the sequelae is thrombus organization and eventual fibrotic occlusion of the vein or associated valvular damage.
Thrombolytic agents were developed to break down thrombus and complement the anticoagulation provided by heparin. In 1977, streptokinase received U.S. Food and Drug Administration (FDA)-approval for intravenous systemic therapy for DVT. Although early clinical studies demonstrated the superiority of lytic therapy compared with anticoagulation alone, systemic streptokinase therapy never received widespread acceptance for DVT because of adverse bleeding complications and immunologic reactivity.1,2 In extensive thrombosis of larger veins, systemic thrombolytic therapy was marginally effective.3
Catheter-directed thrombolysis was proposed initially by Dotter in 1974 as a method to improve thrombolysis.4 The rationale was simple: Instead of systemic infusion, in which drug interaction with the thrombus was inefficient and nontargeted, a catheter was inserted and used to deliver the lytic agent directly into the thrombus, thus greatly improving the efficiency of clot lysis. Catheter-directed thrombolysis has emerged to become the standard-of-care in the endovascular management of thrombosed surgical bypass grafts, peripheral arterial emboli and thromboses, and upper-extremity venous thrombosis. Since the early 1990s, there has been growing international interest in the development of catheter-directed thrombolysis techniques for lower extremity DVT.5,6 The focus of this chapter is to discuss the pharmacologic agents used in venous interventions, indications for treatment, and long-term outcomes of catheter-directed thrombolysis for lower-extremity DVT.
Antithrombotic and Thrombolytic Agents
Of the rapidly growing technologies in endovascular medicine, few interventional radiologists are familiar with the exploding science of antithrombotic and thrombolytic agents. Most are familiar with two first-generation drugs, urokinase and heparin, both developed nearly 20 to 50 years ago. The use of these newer compounds will become increasingly important in the management of DVT and eventually may prove to enhance synergistically the rate of venous thrombolysis.
Low-molecular-weight Heparins
The standard management of DVT involves anticoagulation using intravenous unfractionated heparin followed by oral warfarin for 6 months with a desired international normalized ratio (INR) between 2.0 and 3.0. A recent advance in anticoagulation therapy is the introduction of low-molecular-weight heparins (LMWH) for the management of DVT (Table 33–1). Low-molecular-weight compounds are depolymerized heparinoids derived from enzymatic digestion of unfractionated bovine mucosal heparin. Their main advantage is a long half-life and the ability to provide relatively rapid systemic anticoagulation using a subcutaneous injection once or twice a day for the prophylaxis or treatment of DVT. LMWHs are direct inhibitors of factor X, and monitoring of routine partial prothromboplastin times (PTT) is unnecessary during anticoagulation therapy. Outpatient subcutaneous injections combined with decreased necessity for coagulation studies lead to an overall pharmacoeconomic advantage over standard inpatient intravenous heparin therapy.7 In our practice, to decrease hospital stay, we routinely use enoxaparin (Lovenox, Rhone-Poulenc, Collegeville, PA) instead of intravenous heparin at the conclusion of our endovascular procedure while converting the patient to long-term warfarin. The dosing of enoxaparin is based on weight and indication. Patients with DVT treated with endovascular methods undergo 1 mg/kg injections twice a day (or 1.5 mg/kg once a day) for 7 days while concomittantly taking oral warfarin. The INR is monitored on an outpatient basis during warfarin therapy until the patient achieves steady-state values between 2.0 and 3.0. Despite the advantages of LMWH, neither unfractionated or low-molecular-weight heparinoids actively promote thrombolysis.
Antiplatelet Agents
ADP-receptor Inhibitors
For the past several decades, the mainstay of antiplatelet therapy has been aspirin, which remains the bench mark for comparison with newer agents. Aspirin is an inhibitor of the platelet enzyme cyclooxygenase and binds to prevent the formation of thromboxane A2, the most potent stimulator of platelet aggregation known. Despite the permanent inhibition of cyclooxygenase by aspirin, other pathways exist that can trigger platelet aggregation including activation of adenosine 5′-diphosphate (ADP) receptors on the platelet surface. Recently, the FDA approved two agents that specifically block the platelet adenosine diphosphate (ADP)-receptor (see Table 33–1): ticlopidine (Ticlid, Roche Laboratories, Nudey, NJ) and clopidogrel (Plavix, Bristol-Myers Squibb, Princeton, NJ). Ticlopidine is approved for reducing the risk of a thrombotic stroke in patients with transient ischemic attacks or with completed strokes. Clopidogrel is indicated for a reduction of atherosclerotic events, such as myocardial infarction (MI), stroke, and vascular death, in patients with prior stroke, MI, or peripheral vascular disease. Both drugs have shown superiority compared with aspirin and placebo.8,9 Aspirin remains a commonly used antiplatelet agent in our practice because of its availability and low cost and is given during follow-up after venous interventions. The ticlopidine or clopidogrel may have a role when combined with lytic therapy to accelerate and enhance the rate and efficiency of clot lysis.10,11
Low-molecular weight heparin (tradename, supplier) 1. Enoxaparin (Lovenox, Rhone-Poulenc, Collegeville, PA) 2. Dalteparin (Fragmin, Pharmacia/Upjohn, Stockholm, Sweden) 3. Ardeparin (Normiflo, Wyeth Ayerst, Philadelphia, PA) 4. Danaparoid (Orgaran, Organon, West Orange, NJ) 5. Nadroparin (Fraxiparin, Sanofi, Paris, France) 6. Tinzaparin (Logiparin, Novo and Leo, Copenhagen, Denmark) 7. Certoparin (Sandoparin, Novartis, Nuremburg, Germany) 8. Reviparin (Clivarin, Knoll AG, Ludwigshafer, Germany) Platelet ADP-receptor inhibitors (tradename, supplier) 1. Ticlopidine (Ticlid, Roche, Nutley, NJ) 2. Clopidogrel (Plavix, Bristol-Meyers Squibb, Princeton, NJ) Platelet IIbIIIa-receptor inhibitors (tradename, supplier) 1. Abciximab (RheoPro, Centocor, Malvern, PA) 2. Eptifibatide (Integrilin, Cor-Key/Schering-Plough, Kenilworth, NJ) 3. Tirofiban (Aggrastat, Merck, West Point, PA) |
ADP, adenosine diphosphate.
Glycoprotein IIbIIIa Inhibitors
A key step in thrombus formation is the binding of circulating fibrinogen to the surface of the activated platelet, leading to platelet–platelet aggregation. The platelet surface receptor that binds to fibrinogen is glycoprotein (GP) IIbIIIa, and it is estimated that the average platelet has 80,000 GP IIbIIa receptors. Even when the thromboxane A2 pathway is blocked, as with aspirin therapy, the platelet still can aggregate through other pathways. Whether the platelet is stimulated by von Willebrand factor, ADP, epinephrine, or thrombin exposure, the final common pathway is the activation of the platelet receptor GP IIbIIIa. Therefore, the GP IIbIIIa receptor is potentially a more rational target for the prevention of thrombotic events than other components in thrombus formation.
Three agents are currently approved by the FDA (see Table 33–1): abciximab (RheoPro, Centocor/Lilly, Malvern, PA), eptifibatide (Integrilin, Cor-Key/Schering-Plough, South San Francisco, CA), and tirofiban (Aggrastat, Merck, West Point, PA). All the current agents are for intravenous use only, although studies are under current clinical trial using oral GP IIbIIIa receptor blockers. Abciximab is a chimeric mouse–human monoclonal antibody specific to the GP IIbIIIa receptor and is approved as an adjunct to percutaneous coronary interventions in the prevention of cardiac ischemic complications such as acute clot formation in a freshly angioplastied artery.12 Because it is a human-murine compound, it is antigenic (48,000 d). Eptifibatide is a synthetic cyclic peptide analog of barbourin, a snake venom found in the pygmy rattlesnake Sistrurus m. barbouri and does not have any documented antigenicity because of its relatively small size (831 d). Eptifibatide is indicated for the treatment of acute coronary syndrome (unstable angina and non-Q-wave myocardial infarction) and as an adjunct during percutaneous coronary interventions.13 Tirofiban is a nonpeptide tyrosine analog (495 d) and is approved for use with intravenous heparin for treatment of acute coronary syndrome or during percutaneous coronary interventions.14 The role of these agents in combination with thrombolytic therapy is currently undergoing clinical investigation, but early data suggest a synergistic effect in increasing the rate of thrombolysis.15
Thrombolytic Agents
The common feature of the thrombolytic agents is the ability to activate plasminogen. Plasminogen is cleaved to plasmin, which then enzymatically breaks apart the fibrin links that bind aggregated platelets together. Currently, five thrombolytic agents are approved by the FDA (Table 33–2): streptokinase, urokinase, anistreplase, alteplase and reteplase.
Streptokinase is a derivative of the bacterial protein produced by group C β-hemolytic streptoccoci and is approved for intravenous therapy of DVT, arterial thrombosis and emboli, acute MI (AMI), and occluded arteriovenous cannulas. Streptokinase is an indirect activator of plasminogen because it first must form an intermediate complex before enzymatic conversion of plasminogen to plasmin. Because of its antigenicity and increased bleeding risks, streptokinase is no longer widely used in catheter-directed therapy.
Urokinase is a direct plasminogen activator found naturally in urine and is derived from human renal cell tissue culture. Urokinase received FDA approval in 1978 for the intravenous treatment of pulmonary embolism, coronary artery thrombosis, and clearance of thrombosed catheters, and it is probably the most commonly used lytic agent in catheter-directed therapy worldwide.16
First Generation (tradename, supplier) 1. streptokinase (Streptase, Astra Pharmaceuticals, Wayne, PA) 2. urokinase (Abbokinase, Abbott Laboratories, North Chicago, IL) Second Generation (tradename, supplier) 1. alteplase (Activase, Genetech, South San Francisco, CA) 2. anistreplase (Eminase, Roberts Pharmaceutical, Eatontown, NJ) Third Generation 1. reteplase (Retevase, Centocor, Malvern, PA) Future Agents 1. r-TNK-t-PA 2. r-prourokinase 3. r-staphylokinase |
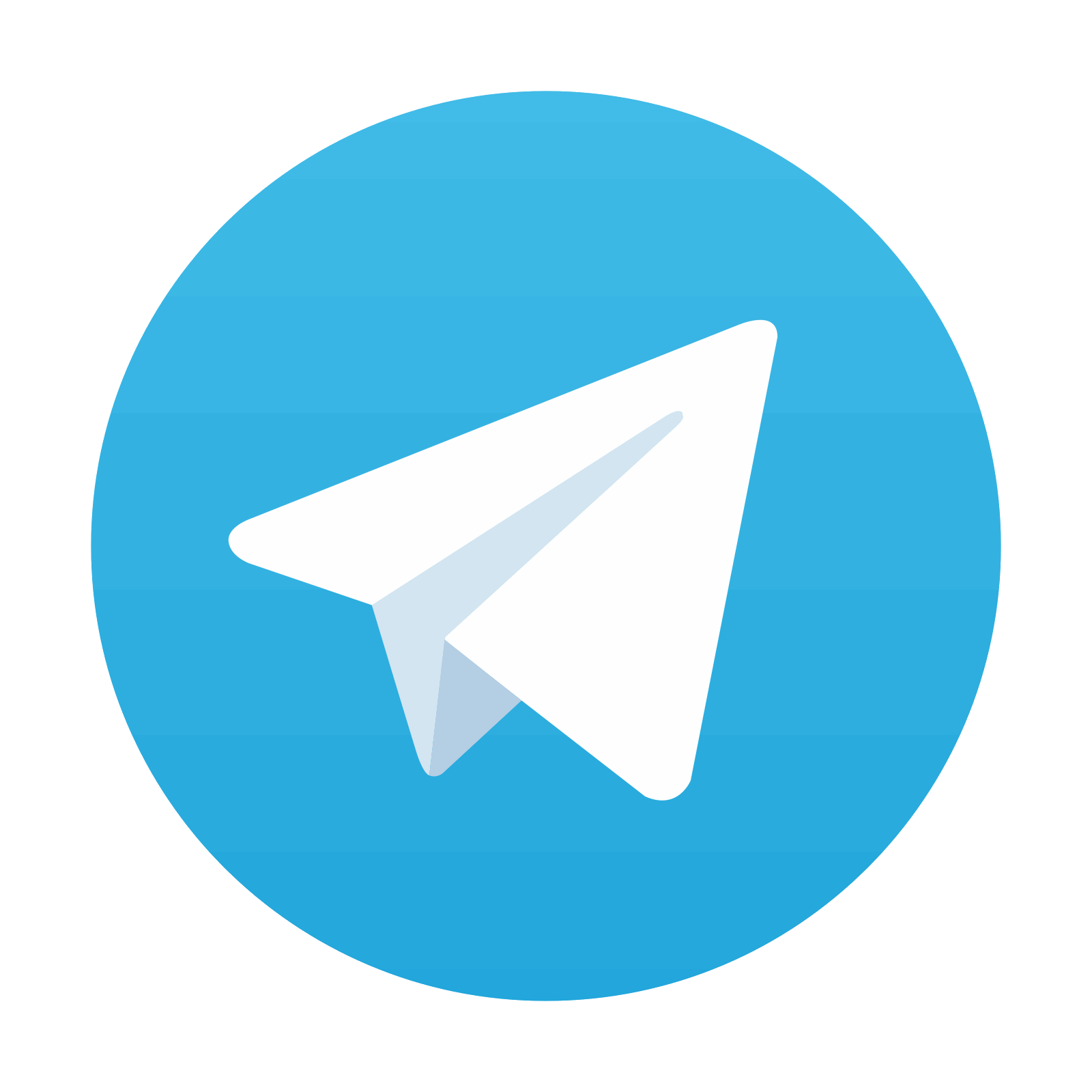
Stay updated, free articles. Join our Telegram channel

Full access? Get Clinical Tree
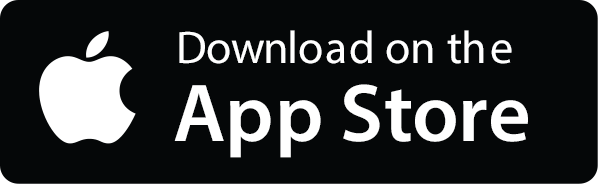
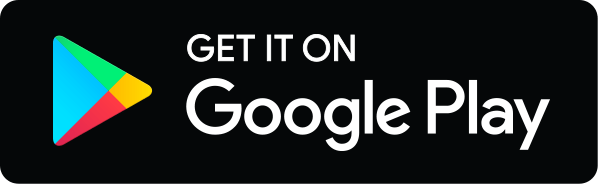