Fig. 32.1
Anatomic variants of renal arteries. (a) In a majority of cases (55 %), single renal arteries are present bilaterally. Other variants include (b) early branching segmental arteries (14 %), (c) dual renal arteries supplying a single kidney (8 %), and (d) an accessory renal artery (7 %) (From Safian and Madder [24] with permission)
Potential sources of collateral supply in the event of renal arterial occlusion include intercostal, lumbar, internal iliac, and adrenal arteries [26]. These anastomotic pathways occur at the level of perforating capsular arterioles. However, the renal artery is functionally an “end artery,” and though these collaterals may preserve viability in the short term in the event of acute renal artery occlusion, they are insufficient to prevent progressive loss of renal mass in the setting of chronic occlusive disease [27]. In contrast, collateral venous pathways are likely to provide adequate outflow to maintain viability in the event of a chronic renal vein occlusion [28].
Pathophysiology of Renal Artery Stenosis
Renal artery stenosis can cause hypertension, renal dysfunction, and cardiopulmonary disturbance syndromes (Fig. 32.2).
The mechanism of hypertension in renal artery stenosis is thought to be related to activation of the renin-angiotensin axis. Decreased renal perfusion pressure distal to the culprit stenosis leads to elevation in serum renin levels. Elevated renin results in increased angiotensin II production. The outcome is vasoconstriction, decreased sodium excretion in the affected kidney, and activation of the sympathetic nervous system. If the stenosis is unilateral, vasoconstriction is thought to be the primary mechanism of hypertension. If the stenosis is bilateral, sodium and volume retention is the primary cause of hypertension [3, 30]. Similarly, volume retention can lead to pulmonary edema, and uncontrolled hypertension can exacerbate symptoms from obstructive coronary artery disease.
The mechanistic link between renal artery stenosis, ischemic injury to the kidney, and renal dysfunction is less clear, partly because the kidney requires only 10 % of the oxygen delivered under normal circumstances [30]. That is, renal excretory dysfunction in the setting of renal artery stenosis may not be due to ischemia, per se. The deleterious effect of concomitant hypertension may have a more prominent role than actual ischemia. Profibrotic mediators induced by angiotensin II – such as transforming growth factor beta, nuclear factor–kB, and platelet-derived growth factor – may also be responsible for renal injury [31–34]. Finally, local atheroembolic events from the culprit renal artery stenosis to the renal parenchyma may contribute [35].
Renal Translesional Hemodynamics
As for lesion assessment in the coronary circulation, the concept of translesional hemodynamic assessment is becoming an increasingly important aspect of renal artery intervention for many of the same reasons [36]. The correlation of angiographic stenosis with the actual physiologic severity of a renal artery stenosis is limited [36–38]. Therefore, translesional hemodynamic assessment is attractive. However, simply measuring a pressure gradient across a stenosis is not necessarily “lesion specific”; that is, such a gradient is dependent on the aortic pressure, the size of the catheter across the lesion, the downstream renal microvasculature, and the renal venous pressure in addition to the actual severity of the stenosis [36]. To circumvent these confounding issues, hyperemic translesional gradients and the fractional flow reserve can be employed, which requires understanding of the concept of renal flow reserve.
The renal flow reserve is the proportional increase in renal blood flow in a state of hyperemia compared to baseline in a normal kidney [39]. The renal flow reserve reflects the capacity of overall blood flow to a kidney to increase in response to vasodilatation of the renal microvasculature. In a normal kidney, the flow reserve approaches two with maximal hyperemia [40]. In a stenotic renal artery with normal or diseased parenchymal vessels, the flow reserve is less than in a normal kidney [39]. The lack of vasoreactivity of the renal microvasculature in the post-stenotic kidney may reflect maximal vasodilatation or irreversible microvascular disease. Following revascularization of the post-stenotic kidney with normal parenchymal vessels, the flow reserve increases to supranormal values, indicating lack of maximal vasodilatation of the renal microvasculature at rest and lack of irreversible renal microvascular disease [39]. In contrast, in the stenotic renal artery with abnormal parenchymal vessels, the flow reserve should remain abnormal following revascularization, indicating irreversible microvascular disease.
If the renal artery diameter remains constant, then the renal flow reserve is equal to the hyperemic average peak velocity divided by the baseline average peak velocity. Invasive measurement of flow velocities requires a Doppler wire, which is not widely available. Thus, fractional flow reserve is often used as a surrogate for direct measurement of flow velocities, when assessing the effects of a stenosis on flow reserve.
Renal fractional flow reserve is defined as the maximal renal blood flow through a stenotic renal artery divided by the maximal flow in the artery without a stenosis [36]. Maximal renal blood flow in a stenotic artery is equal to the difference of the hyperemic pressure distal to the stenosis and the mean central venous pressure divided by the renal arteriolar resistance. Similarly, the maximal renal blood flow in the absence of a stenosis is equal to the difference of the mean aortic pressure and the mean central venous pressure divided by the renal arteriolar resistance [36]. Under maximal hyperemia, the renal resistance is assumed to be constant and negligible. The central venous pressure is typically near 0 mmHg and negligible. In such conditions, the fractional flow reserve can be calculated with the ratio of the hyperemic pressure distal to the stenosis divided by the mean aortic pressure [41]. However, this assumption is not the case in patients with decompensated heart failure in which disregarding the elevated central venous pressure will result in a falsely elevated (less severe) estimated fractional flow reserve [41].
Because of the limited flow reserve of the renal circulation, particularly in the presence of renal artery stenosis, it may be more appropriate to simply measure hyperemic translesional pressure gradients [38]. In the post-stenotic kidney, the efferent arterioles constrict to maintain filtration pressure, which increases the pressure distal to the stenosis. Thus, a significant translesional pressure gradient may be masked at baseline. To remove this confounder, an arteriolar vasodilator specific to the kidney can be administered. Medications that are used to dilate the renal microvasculature and induce hyperemia include papaverine, dopamine, fenoldopam, and isosorbide dinitrate. Dopamine is thought to best achieve maximal hyperemia in the kidney [40]. In contrast, isosorbide dinitrate preferentially vasodilates the main renal arteries and thus does not establish a state of complete hyperemia.
In summary, the clinical implications of assessing hyperemic translesional gradients are multifold. First, removing the effects of efferent arteriolar vasoconstriction is necessary to more accurately assess the hemodynamic significance of a proximal renal artery stenosis [40]. Second, return of vasoreactivity of the renal microvasculature following revascularization may be a surrogate for viability of the kidney and/or reversibility of the clinical syndrome caused by the renal artery stenosis [40, 42].
Clinical Approach to Renal Vascular Diseases
By far, the most common renal vascular disease is renal artery stenosis. Atherosclerosis accounts for >90 % of renal artery stenosis, while fibromuscular dysplasia is the second most common cause (Table 32.1). Transplant renal artery stenosis is a rare, yet emerging, entity. Acute renal vascular disorders are a much less common category of renovascular disease.
Table 32.1
Comparison of renal artery stenosis due to atherosclerosis or fibromuscular dysplasia
Atherosclerosis | Fibromuscular dysplasia | |
---|---|---|
Typical clinical syndrome | Renal dysfunction | Hypertension |
Typical demographic | Older patients with multiple risk factorsor evidence of atherosclerosis | Younger females |
Imaging characteristics | Ostial or proximal stenoses,often calcified | Middle/distal stenoses |
“Beads-on-a-string” of medial fibroplasia | ||
Smooth or napkin-ring stenoses or redundancy if intimal fibroplasia | ||
Diagnostic invasive approach | Translesion hemodynamic assessmentencouraged | Translesion pressure gradient and IVUS extremely important for medial fibroplasia |
Therapeutic invasive approach | Stenting recommended | Angioplasty usually sufficient |
Outcomes | Cure/improvement 50–70 % | Cure/improvement 70–90 + % |
Atherosclerotic Renal Artery Stenosis
Presentation
Atherosclerotic renal artery stenosis can cause hypertension, renal excretory dysfunction, and cardiopulmonary disturbance syndromes. A patient with hypertension and renal artery stenosis may have essential hypertension, hypertension due to the renal artery stenosis (i.e., “renovascular hypertension”), or both. Likewise, a patient with renal ischemia may have excretory dysfunction of the post-stenotic kidney; however, disease of the parenchyma may be due to ischemia (i.e., “ischemic nephropathy”), hypertension (i.e., “hypertensive nephropathy”), diabetes (i.e., “diabetic nephropathy”), or other causes [24].
Hypertension is commonly found in patients with renal artery stenosis. Several clinical features classically distinguish hypertension due to renal artery stenosis from essential hypertension. The age of onset is typically either younger or older than that for essential hypertension. If a patient presents with hypertension under the age of 30 years, then renal artery stenosis – particularly, due to fibromuscular dysplasia – should be considered. A late presentation (>55 years) is also suggestive of a secondary cause such as renal artery stenosis. Older patients will also often have known atherosclerosis of other arterial beds. Hypertension due to renal artery stenosis may be refractory to multiple medications, be rapidly progressive, or be malignant with evidence of end organ damage [2].
Renal ischemia is another manifestation of renal artery stenosis that may occur with or without renovascular hypertension. Excretory dysfunction is difficult to detect by serum creatinine unless there is bilateral renal artery ischemia or ischemia of a solitary kidney. Several clinical features can help distinguish excretory dysfunction from renal ischemia from other causes of renal dysfunction, a difficult task for the clinician. A rapid increase in serum creatinine following the initiation of angiotensin-converting enzymes is suggestive of bilateral renal artery stenosis. Alternative causes of renal dysfunction – hypertension, diabetes, intrinsic renal disease, or post-obstructive causes – should be absent, though this is not always the case. Proteinuria is common to many forms of nephropathy, including ischemic nephropathy, but the urinary sediment should be acellular in the presence of renal ischemia [43]. If an elevated serum creatinine exists in the presence of unilateral renal artery stenosis, the diagnosis of clinically significant renal artery stenosis should be questioned as a normally functioning single kidney is adequate to maintain normal serum creatinine levels [3].
Cardiopulmonary syndromes include decompensated heart failure, classically manifested by “flash pulmonary edema,” and unstable angina. Cardiopulmonary syndromes are often seen in association with uncontrolled hypertension and/or renal dysfunction. Clinically significant renal artery stenosis should be expected in the setting of decompensated heart failure in the absence of left ventricular or valvular dysfunction [4].
Diagnostic Testing
Diagnostic evaluation must go beyond simple demonstration of an anatomically severe renal artery stenosis. Rather, to optimally select which patients might benefit from revascularization, the clinician should seek information regarding the physiologic severity of a lesion, parenchymal disease of the post-stenotic kidney, and the cause-effect relationship of a stenosis to a particular clinical syndrome. Granted, it is challenging to demonstrate these features in every case, but this approach is useful in guiding the evaluation of patients with renal artery stenosis. The lack of such an approach has likely contributed to the inability of prior randomized trials to show a benefit of renal artery revascularization over medical therapy alone.
Serologic Studies
Serum creatinine is perhaps the most commonly used test to assess for nephropathy of any cause. However, serum creatinine levels do not become elevated until more than 50 % of renal mass is lost [24].
Baseline serum creatinine and estimated glomerular filtration rate have been an inconsistent predictor of clinical improvement following renal artery stenting, often due to lack of strong competing predictor variables adjusted for in various prediction models [44–49]. However, the rate of decline of renal dysfunction (expressed by slope of 1/serum creatinine over time) prior to revascularization may be a predictor of improvement following revascularization, with a steeper rate of decline indicating a higher chance of improvement in renal function as compared to stable, longstanding excretory dysfunction [45, 49]. It may be that actively declining renal function is a surrogate for residual viability.
Other noninvasively collected serologic studies that have been studied in the diagnostic evaluation of renal artery stenosis include plasma renin activity and B-type natriuretic peptide. Plasma renin activity (measured before and/or after administration of captopril) has poor diagnostic characteristics for the detection of physiologically significant renal artery stenosis and is not recommended (class III) by the American Heart Association in the evaluation of renal artery stenosis [2]. On the other hand, B-type natriuretic peptide is more promising. This hormone may predict improvement in hypertension following renal artery revascularization. In a small cohort of patients (n = 27) with refractory hypertension and atherosclerotic renal artery stenosis, 17 of 22 patients with a BNP level greater than 80 pg/mL prior to renal artery stenting were shown to have improvement in hypertension (<140/90 mmHg or decrease in DBP by 15 mmHg on same or fewer antihypertensives) at a mean follow-up of 3.5 ± 1.3 months [50]. In contrast, 0 of five patients with a BNP < =80 pg/mL had improvement in hypertension post-stenting. Of note, these results do not generalize to patients with renal excretory dysfunction due to renal artery stenosis or cardiopulmonary disturbance syndromes as exclusion criteria for this study included serum creatinine > =2 mg/dL and decompensated heart failure. Also, the optimal BNP cutoff for this indication should be validated in other study populations. The interpretation of early results from the Hercules (Herculink Elite Renal Stent to Treat Renal Artery Stenosis) study has been pessimistic regarding the predictive ability of BNP for improvement in hypertension following revascularization.
Urine Studies
Proteinuria is a nonspecific marker of nephropathy of multiple causes, including ischemic nephropathy [43]. The degree of proteinuria may have predictive ability regarding improvement in renal function following revascularization for renal artery stenosis. In a retrospective study of 83 patients with renal dysfunction who had undergone percutaneous renal artery revascularization, the presence of >0.6 g/day of urinary protein had an odds ratio of 6.4 (95 % CI 1.4–27.6) for lack of improvement in renal function following revascularization at a mean follow-up of 22 months [51]. Thus, higher degrees of proteinuria may predict less residual renal function to be salvaged by revascularization.
The urinary sediment should be bland in patients with ischemic nephropathy. The presence of cellular casts, for example, is suggestive of an alternative, or additional, cause of nephropathy.
Noninvasive Imaging
Duplex ultrasonography is an excellent initial imaging study in the evaluation of renal artery stenosis. It can provide anatomic information on lesion severity (2-D imaging), physiologic information on lesion severity (color and spectral Doppler and velocity measurements), and insight into parenchymal disease (renal resistive index and renal size). Though not universally adopted by vascular laboratories, a peak systolic velocity >200 cm/s and a renal artery/aortic peak systolic velocity ratio of 3.5 is generally consistent with a physiologically significant stenosis. Limitations of duplex ultrasonography include a high degree of expertise needed by the ultrasonographer and inability to attain high quality images due to patient habitus or bowel gas.
Exclusion criteria for many studies of renal artery revascularization have included a renal size <7 cm, assuming this finding is a surrogate for non-viability of the post-stenotic kidney. A normal-sized kidney is >/=10 cm and is reassuring that residual renal function is present. In a prospective cohort study of 215 patients who had undergone renal artery stenting for uncontrolled hypertension, a renal parenchymal/pelvic ratio was an independent predictor of improvement in mean blood pressure 1 year after the intervention [48].
The renal resistive index is defined by the formula 1 – (EDV/PSV). Values >0.80 are considered abnormal and representative of small vessel renovascular disease. This parameter can be a useful prognosticator if interpreted correctly in the context of a particular patient’s clinical circumstances. In a prospective cohort study of 138 patients who had undergone renal artery revascularization, a renal resistive index >=0.80 was a strong independent predictor of lack of improvement in renal function following revascularization. Conversely, an index <0.80 was a strong independent predictor of improvement in both renal function and hypertension [52]. Of note, if a segment distal to a critical stenosis is insonated, the RRI will be normal (due to a parvus et tardus waveform) but is not necessarily indicative of lack of parenchymal vascular disease. Thus, the renal resistive index is more useful as a prognostic test than as a diagnostic study for renal artery stenosis.
Computed tomographic angiography and magnetic resonance angiography are considered to be accurate diagnostic tests for characterizing the anatomic severity of the typical ostial of proximal renal artery stenosis found in atherosclerotic disease [53]). In a meta-analysis of 25 studies encompassing 998 patients, gadolinium-enhanced magnetic resonance angiography had a sensitivity of 97 % (95 % CI: 93 ± 98 %) and specificity of 93 % (95 % CI: 91 ± 95 %) for severe renal artery stenosis in general with catheter-based angiography as the gold standard [54]. Additional advantages and limitations of CT and MR angiography are listed in Table 32.2.
Table 32.2
Comparison of imaging modalities for renal artery stenosis
Modality | Pros | Cons |
---|---|---|
Doppler ultrasonography | Inexpensive | Highly operator dependent |
Noninvasive | Limited visibility in obese patients | |
No radiation exposure | Challenging in transplant renal artery stenosis | |
No contrast use | ||
Able to obtain functional information | ||
CT angiography | Good characterization of aortaand proximal large vessel stenoses | Radiation exposure |
Use of contrast | ||
Limited visibility in presence of severe calcification | ||
Can be difficult to see mid/distal renal arteries (FMD, dissection) | ||
MRA | Good characterization of aortaand proximal large vessel stenoses | Expensive |
No radiation exposure | Can be difficult to see mid/distal renal arteries (FMD, dissection) | |
High false-positive rate | ||
Scintigraphy | Physiologic information on stenosis severity | Poor diagnostic characteristics in presence of bilateral renal artery stenosis, renal artery stenosis to a solitary kidney, and global nephropathy of any cause |
Information on excretory function | ||
Invasive angiography | Superior anatomic definition of stenoses | Risk of vascular complications |
Hemodynamic assessment | Radiation exposure | |
Use of contrast (less than CT angiography) |
Renal scintigraphy is another noninvasive imaging modality for the evaluation of renal artery stenosis. This test involves intravenous administration of a technetium 99 m-labeled compound to a patient followed by imaging to assess the time to maximum activity of a tracer in the kidney. Additionally, the symmetry of tracer uptake between kidneys, the retention of tracer in the renal cortex, and the effect of captopril on these same parameters on repeat imaging following the administration of oral captopril is determined. This study provides information about size, perfusion, and excretory function. Delayed uptake and decreased uptake compared to contralateral kidney are suggestive of renal ischemia. Cortical retention of tracer is indicative of nephropathy in general. The diagnostic characteristics of this modality for physiologically significant renal artery stenosis is poor in the setting of bilateral renal artery stenosis, renal artery stenosis to a solitary kidney, and global nephropathy of any cause [2]. However, abnormal unilateral function, defined by a ratio of <=0.45 of estimated blood flow to the affected kidney/total bilateral renal blood flow, has been shown to be an independent predictor of improvement in hypertension or renal function in a prospective cohort study of 40 patients who underwent renal artery stenting for uncontrolled hypertension or renal dysfunction [55]. Likely, lateralization of perfusion on a renal scintigram is a surrogate for a hemodynamically significant stenosis.
Catheter-Based Evaluation
Catheter-based angiography is considered the gold standard technique for characterizing the anatomic severity of a renal artery stenosis (Fig. 32.3a). This modality is typically not the 1st-line imaging test for atherosclerotic renal artery stenosis due to the small but real risk of vascular complications. However, it should be mentioned that less contrast is required for diagnostic catheter-based angiography (<20–25 cc for abdominal aortography; <10 cc for each renal artery) compared to CT angiography (>85 cc iodinated contrast) [53]. The technique of catheter-based angiography is discussed later in the chapter.
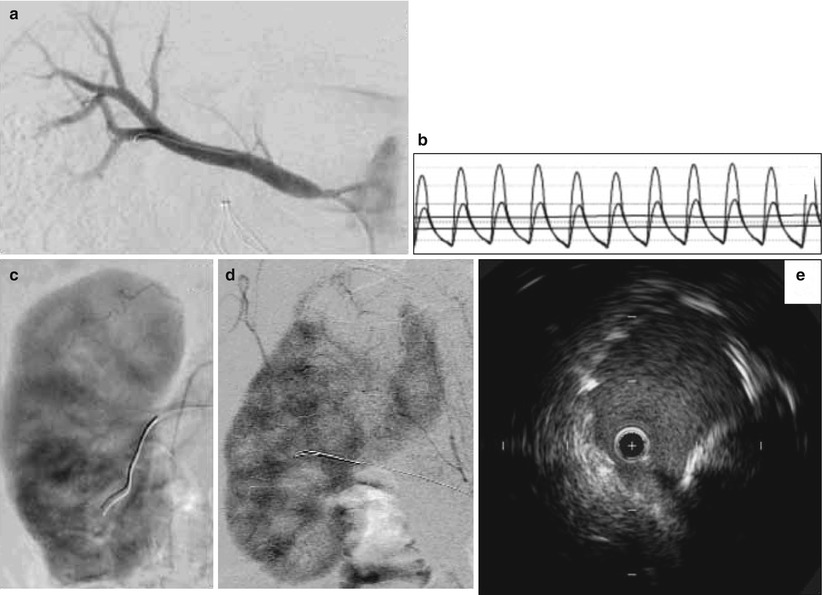
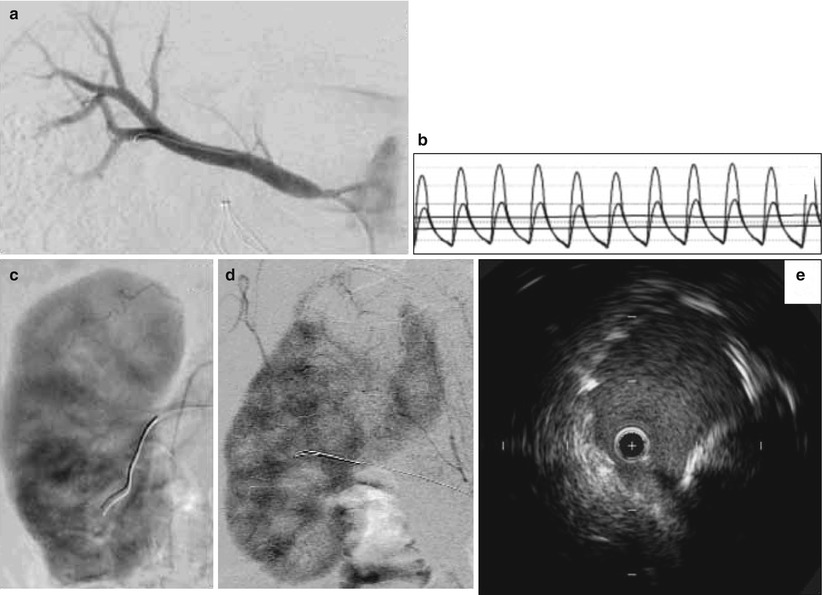
Fig. 32.3
Catheter-based assessment of renal artery stenosis. (a) Selective right renal angiogram demonstrating the typical ostial location of an atherosclerotic renal artery stenosis. (b) Translesional hemodynamic measurements obtained from a 0.014-in. flow wire in a different patient. After intrarenal administration of 40 mg of papaverine, the FFR was 0.6 and the hyperemic systolic gradient was 40 mmHg. (c) Normal nephrogram. (d) Nephrogram indicating abnormal perfusion of the upper pole of the kidney. (e) IVUS showing underdeployed renal artery stent that was subsequently post-dilated
Measurement of translesional pressure gradients provides important diagnostic information as angiographic evaluation alone is thought to be an inaccurate measure of lesion severity [36, 41, 56, 57] (Fig. 32.3b). With a flow wire or small caliber catheter, systolic and mean translesional pressures can be measured at rest. With a flow wire, a hyperemic translesional systolic and mean gradient as well as a fractional flow reserve can be measured after the administration of a renal vasodilator. In a prospective study of 17 patients with medically refractory hypertension, 86 % of those with a FFR < 0.8 after intra-arterial administration of papaverine had improvement in hypertension at 90 days following renal artery stenting. In contrast, only 30 % of those with a hyperemic FFR > 0.8 experienced improved blood pressure control [36]. In another prospective study of 62 patients with unilateral renal artery stenosis 50–90 % by qualitative angiography, among multiple hemodynamic, angiographic, and IVUS variables, a hyperemic systolic gradient measured by a flow wire was the only independent predictor of clinical improvement in hypertension at 12 months following renal artery stenting. In this study, 84 % of those with a hyperemic systolic gradient >=21 mmHg showed improvement in blood pressure control compared to 36 % for those with a hyperemic systolic gradient <21 mmHg [38]. Similar findings were demonstrated in a prospective study of 53 patients with unilateral renal artery stenosis and refractory hypertension in which a dopamine-induced hyperemic mean gradient was the strongest predictor, among other translesional hemodynamic variables, of improvement in hypertension after renal artery stenting [37]). In this study, a dopamine-induced hyperemic mean gradient had an area under the receiver operator characteristic curve of 0.77 (95 % CI 0.64–0.90) for improvement in hypertension 3 months following stenting. The optimal cutoff value was 20 mmHg.
The predictive ability of hyperemic gradients likely reflects more accurate assessment of lesion severity by removing the effects of downstream resistance that confounds lesion assessment at rest. Furthermore, response in the parenchymal vessels to a vasodilator may represent reversibility of disease and viability of the kidney. The technique of obtaining translesional pressure gradients is discussed later in the chapter [40, 42].
Renal vein renin sampling via a catheter selectively placed in the renal veins has been described in the assessment of renal artery stenosis. In a prospective study of 50 patients with refractory hypertension and unilateral renal artery stenosis, a ratio of <1.5 of the plasma renin activity from the renal vein of the post-stenotic kidney to the plasma renin activity from the renal vein of the contralateral kidney was an independent predictor of failure to improve hypertension following revascularization [58]. Such lateralization of renal vein renin levels may be a surrogate for an ipsilateral, physiologically significant renal artery stenosis. Thus, it is unclear if this modality would have practical, incremental information to translesional hemodynamic data. Clinical utility is also limited if results of the renin assay cannot be obtained in “real time.” The role of renal vein renin sampling may be more useful in guiding the decision to perform nephrectomy of a post-stenotic atrophic kidney in a patient with refractory hypertension [2].
The nephrogram (cineangiographic visualization of contrast uptake into the renal parenchyma) can provide information on the extent of parenchymal disease and may be predictive of outcomes following revascularization (Fig. 32.3c, d). In a retrospective study of 24 hypertensive patients with unilateral renal artery stenosis and 17 controls, Mahmud et al. analyzed the ability of two aspects of the nephrogram to predict outcomes following renal artery stenting. The authors showed that improvement in renal frame count (number of frames required for contrast to reach the distal parenchyma) and renal blush grade (objective score of intensity of parenchymal opacification by contrast) after renal artery stenting predicted better blood pressure control post-intervention [59]. Of note, to determine renal frame count as defined in this study would require image acquisition at 30 frames/s, which is faster than that typically used for digital subtraction angiography. It will be interesting if related future research determines whether any pre-interventional features of the nephrogram are also predictive.
Intravascular ultrasound (IVUS) has been described for renal artery stenting [38, 60] (Fig. 32.3e). The predictive characteristics of IVUS for improvement in blood pressure were studied in 62 patients undergoing renal artery revascularization [38]. The minimum luminal area had an area under the receiver operator characteristic curve of 0.86 (95 % CI 0.76–0.95), indicating excellent predictive value for improvement at 12 months following revascularization. The optimal cut point for the minimum luminal area was 7.8 mm2, which had 79 % predictive accuracy. IVUS may improve technical results, as well. In a retrospective study of 131 patients undergoing IVUS-guided renal artery stenting, IVUS identified 22 (14 %) cases of stent malapposition or underexpansion, 8 (5 %) dissections and 6 (4 %) in geographic miss of the ostium [38, 60]. These findings were not apparent on angiography.
Management
All patients with atherosclerotic renal artery stenosis should receive appropriate medical therapy for atherosclerosis including antiplatelet therapy, HMG CoA reductase inhibitors (statins), and blood pressure control in addition to counseling for smoking cessation and lifestyle modifications. The challenging clinical decision making for renal artery stenosis often involves whether to offer revascularization in addition to optimal medical therapy.
Indications for renal artery revascularization proposed by the American College of Cardiology and American Heart Association in 2005 are summarized in Table 32.3. There are both surgical and endovascular revascularization options. Surgical methods include aorto-renal bypass, extra-anatomic bypass (e.g., splenorenal, hepatorenal), and endarterectomy [2]. The only randomized trial of surgical compared to percutaneous revascularization was conducted in the 1980s and was underpowered (n = 58) and not applicable to contemporary practice (renal artery angioplasty performed without stenting) [61]. In a large surgical series of 323 patients undergoing surgical revascularization for renal artery stenosis, the perioperative mortality rate was 5.6 % [62]. In another similar report from a different institution, the 30-day mortality for surgical renal artery revascularization was 7.4 % [63]. Due to the higher morbidity and mortality associated with surgical revascularization for renal artery stenosis, endovascular treatment is usually preferred. Accordingly, several randomized trials have compared percutaneous renal artery revascularization with medical therapy [64–68].
Table 32.3
2005 ACC/AHA guidelines for renal artery revascularization
Class of indication | Level of evidence | Indication |
---|---|---|
I | B | Hemodynamically significant RAS with recurrent, unexplained HF, or sudden unexplained pulmonary edema |
IIa | B | RAS with either (a) accelerated, resistant, or malignant hypertension, (b) hypertension with unilateral small kidney, and (c) hypertension with medical intolerance |
IIa | B | CKD with bilateral RAS or RAS to solitary kidney |
IIa | B | RAS and unstable angina |
IIb | C | Asymptomatic bilateral RAS or solitary viable kidney with hemodynamically significant RAS |
IIb | C | Asymptomatic unilateral hemodynamically significant RAS in a viable kidney |
IIb | C | RAS and CKD with unilateral RAS with two kidneys present |
Randomized Trials Comparing Percutaneous Renal Artery Revascularization to Medical Therapy
Five randomized trials have been published in which percutaneous renal artery revascularization was compared to medical therapy, and results from a 6th trial are awaited [64–68] (Table 32.4). The initial three trials were small, published in 1998–2000 prior to the routine use of stenting in the renal artery, and included study populations with hypertension. The other two trials were published in 2009 and included larger study populations that consisted primarily of patients with renal insufficiency. The methodology and results of these trials are summarized below.
Table 32.4
Trials of percutaneous renal artery revascularization compared to medical therapy
Study | N | Study population | Intervention | Lesion assessment | Primary outcome | Follow-up |
---|---|---|---|---|---|---|
EMMA [64] | 49 | HTN (DBP >95 or on AHM) | Angioplasty | Angiography | Ambulatoryblood pressure | 6 months |
Unilateral RAS ≥75% or ≥60% + abnormalfunctional study | Scintigraphy | |||||
Functionalcontralateral kidney | IVP | |||||
CrCl ≥50 cc/min | Renal vein renin | |||||
Recent pulmonaryedema excluded | ||||||
SNRASCB [66] | 55 | HTN (DBP >95 + 2 AHM) | Angioplasty | Angiography | Change in meanBP and SCr | 6 months |
Unilateral and bilateralRAS >50% | ||||||
SCr >5.7 mg/dL excluded | ||||||
DRASTIC [67] | 106 | HTN (DBP >95 × 2 + 2 AHM)or increase SCr >0.2 mg/dLon ACEi | Angioplasty | Angiography | SBP and DBP at 3 and 12 months | 12 months |
RAS ≥50% | ||||||
Solitary kidney excluded | ||||||
SCr > 1.7 mg/dL excluded | ||||||
Heart failure excluded | ||||||
STAR [65] | 140 | Renal dysfunction(CrCl <80 mL/min) | Stenting | CT, MR, or angiographyfor inclusion | ≥20% decrease in CrCl | 2 years |
RAS ≥50% | ||||||
CrCl <15 mL/min excluded | Angiography for treatment | |||||
Malignant HTN excluded | ||||||
ASTRAL [69] | 806 | Unexplained renaldysfunction or uncontrollableHTN thought due to RAS | Stenting | CT, MR, US, orangiography for inclusion | Slope of 1/SCr | 34 months |
“Substantial” anatomic RAS | ||||||
Angiography for treatment | ||||||
CORAL [70] | 1,080(goal) | SBP≥155 + 2 AHM | Stenting | Angiography | Composite of CV/renal death, MI, HFhospitalization, stroke,doubling SCr,and dialysis | Ongoing |
Pressure gradient(obtained by catheter,not flow wire) | ||||||
≥80% RAS by angiography | ||||||
60–80 % stenosis byangiography and 20 mmHgsystolic gradient | ||||||
Recent HF excluded |
The 1st randomized trial of percutaneous renal artery revascularization (Essai Multicentrique Medicaments vs Angioplastie, or EMMA) compared to medical therapy included 49 patients with unilateral renal artery stenosis and uncontrolled hypertension [64]. No statistically significant change in the primary outcome of 24-h ambulatory blood pressure was found between the two groups. The study was limited by lack of adequate power, lack of adjunctive stenting in the intervention group, and a high crossover rate (7 of 26 in medical therapy group). Furthermore, the trial was not applicable to those with severe disease as only 8 of 23 in angioplasty group had >75 % stenosis, and those with malignant hypertension, pulmonary edema, and creatinine clearance <50 mL/min were excluded.
The second early randomized trial (Scottish and Newcastle Renal Artery Stenosis Collaborative Group) of percutaneous renal artery revascularization compared to medical therapy included two parallel arms – patients with bilateral renal artery stenosis and unilateral renal artery stenosis – each compared to medical therapy [66]. This trial was also limited by a small study population and lack of stenting in the interventional group. Despite these limitations which bias against a beneficial effect of angioplasty, those in the angioplasty group with bilateral renal artery stenosis exhibited an impressive benefit in the primary outcome of blood pressure improvement at 6 months compared to baseline (mean 166/90 vs. 185/94, SD not provided, p value not provided). In comparison, the medical therapy arm of the bilateral renal artery stenosis group had minimal change in blood pressure over the prespecified follow-up period of 6 months (177/93 vs. 179/91). The comparison of the change in blood pressure between the two groups (17 mmHg systolic/3 mmHg diastolic) in favor of angioplasty did not reach statistical significance. In those with unilateral renal artery stenosis, the blood pressure improved to a similar degree over 6 months in the angioplasty (−9/−5 mmHg) and medical (−8/−6 mmHg) groups.
Renal angioplasty was also compared with medical therapy in the DRASTIC (Dutch Renal Artery Stenosis Intervention Cooperative study) trial [67]. Eligible patients had refractory hypertension or an increase in serum creatinine by 0.2 mg/dL as well as a>=50 % renal artery stenosis due to atherosclerosis by qualitative angiographic assessment by the treating interventionalist. Exclusion criteria included a history of unstable angina or decompensated heart failure, serum creatinine >2.3 mg/dL or >1.7 in presence of solitary kidney, and post-stenotic kidney <8 cm. Though a power analysis was not provided, 106 patients were randomized and followed for 1 year. The primary outcome included the systolic and diastolic blood pressure at 3 and 12 months. Twenty-two of the 50 (44 %) patients in the medical group crossed over to the angioplasty group for either worsening hypertension or renal function. Only two patients who underwent angioplasty received adjunctive stenting. There was no statistical difference in the primary outcome at 3 or 12 months. Regarding secondary outcomes, patients in the angioplasty group were taking fewer antihypertensives than the medical therapy group (1.9 vs. 2.4, p = 0.002) at 12 months. In eight patients in the medical therapy group who did not cross over, the renal artery stenosis progressed to complete occlusion and was associated with an increase of serum creatinine by>=50 %. Restenosis (>=50 %) within the treated renal artery segment occurred in 23 of the 48 patients who underwent repeat angiography. Blood pressure improved in 38 of 56 patients in the angioplasty group but only 18 of 48 in the medical therapy group.
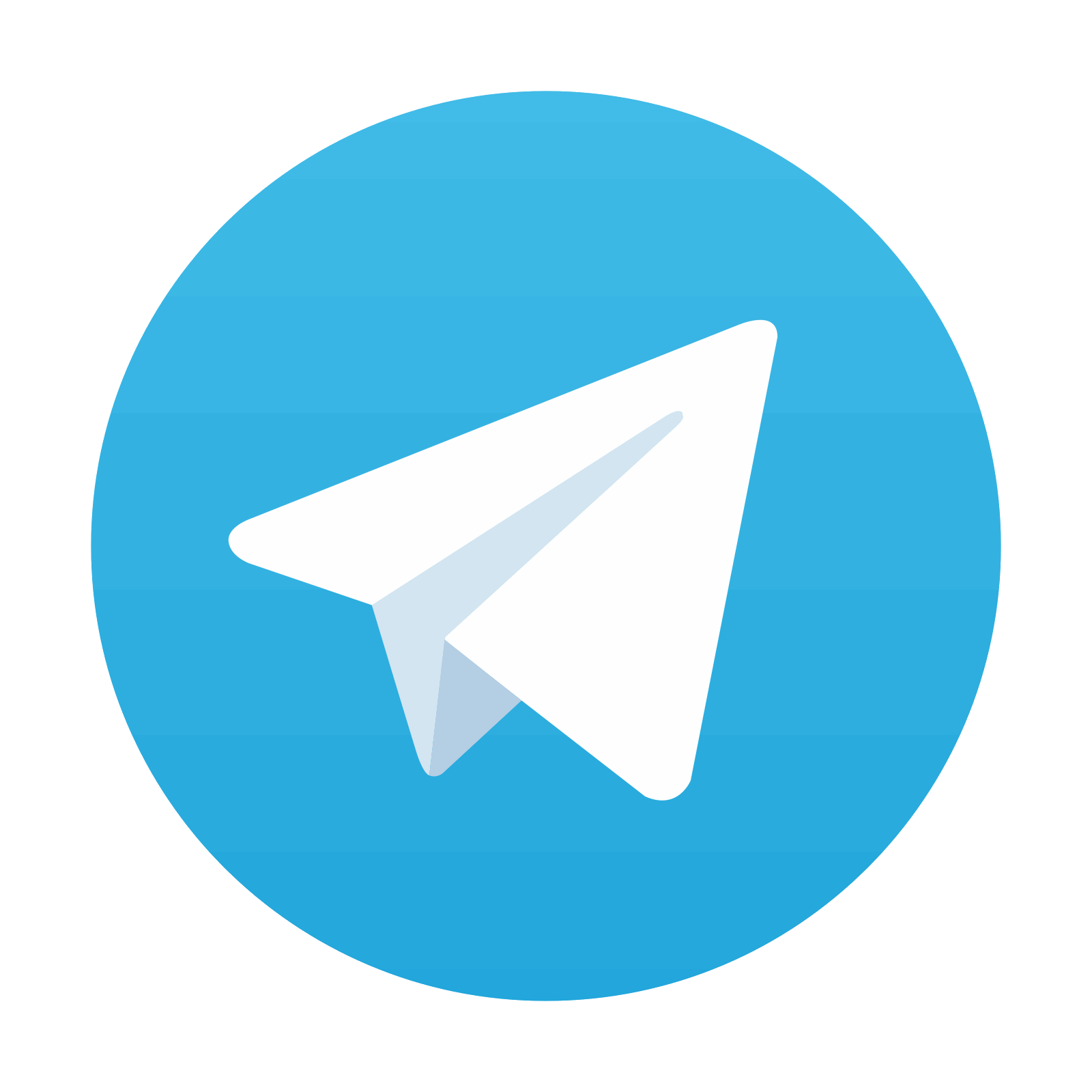
Stay updated, free articles. Join our Telegram channel

Full access? Get Clinical Tree
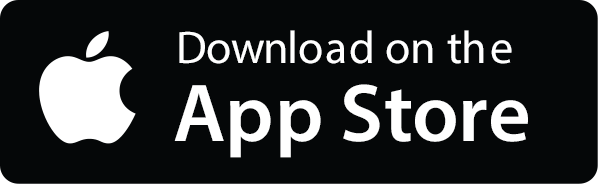
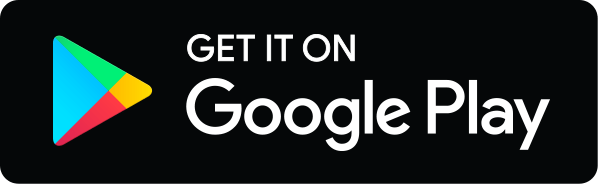