Perfusion in Chronic Carotid Artery Disease
Nolan S. Hartkamp
Jeroen Hendrikse
Reinoud P.H. Bokkers
Chronic carotid artery disease refers to the narrowing of the carotid arteries due to the buildup of an atherosclerotic plaque within the arteries wall.1,2 Atherosclerosis within the carotid arteries is usually the most severe within 2 cm of the bifurcation of the common carotid artery to the internal and external carotid artery. The plaque gradually encroaches the lumen of the artery and leads to a stenosis or complete occlusion of the artery. Steno-occlusive lesions are associated with high morbidity and mortality due to the occurrence of stroke. This is the rapid loss of brain function due to brain tissue ischemia after a disturbance in the blood supply to the brain.
Ischemic stroke is caused by a blockage of freshly oxygenated blood to the brain. Basic physiologic functions such as synaptic transmission, the membrane ion pump, and energy metabolism are disrupted. Within minutes, decreased perfusion can lead to irreversible neuronal damage. Although the pathophysiology of cerebral ischemia occurrence is not completely understood, there are two major processes that underlie the occurrence of stroke. First, the formation of thrombus at the atherosclerotic plaque resulting in thrombo-emboli; and second, hypoperfusion of the brain tissue due to lumen reduction and decreased perfusion in the more distal circulation.3
Previously known as cerebrovascular accident, stroke is the second leading cause of long-term disability and death in high-income countries.4 Symptoms may be transient, lasting seconds to minutes, or persist for a longer period of time. In approximately 25% of the patients presenting with stroke, a stenosis or occlusion caused by atherosclerosis is found in one of the brain’s feeding arteries.5,6
Standard of Care: Current Guidelines Regarding Treatment
Treatment of carotid artery disease depends on the degree of stenosis and whether a patient is symptomatic. For patients with a stenosis, it has become apparent from the results of three high-quality prospective randomized trials that a constriction larger than 60% to 70% of the internal carotid artery (ICA) leads to a significant incidence of stroke.7,8,9 Treatment consists of risk factor management, antiplatelet drug therapy, and medical revascularization therapy. Revascularization can either be done by means of surgical carotid endarterectomy treatment or carotid artery angioplasty with subsequent stent placement. When treated with antiplatelet drug therapy alone, the risk of developing fatal or nonfatal recurrent stroke is 26%. This is lowered to 9% when combined with revascularization treatment.8 The effectiveness for patients who are asymptomatic or have a stenosis 60% or less is unclear, and surgical treatment is therefore currently not recommended.
Patients with a complete occlusion of one of the ICAs are at greater risk for future stroke. The annual risk of brain tissue ischemia is 5% to 6% and in those with compromised cerebral perfusion and poor collateral blood flow it is raised to 9% to 18% per year.10,11,12,13 Treatment consists primarily of risk factor management and antiplatelet drug therapy. Surgical revascularization treatment has been largely abandoned as an international randomized trial showed in 1985 that extracranial-to-intracranial (EC-IC) bypass surgery does not prevent stroke in patients with symptomatic ICA occlusion.14 There are however studies that suggest however that EC-IC bypass surgery may be effective in a subgroup of patients with severely impaired cerebral perfusion.14,15 Cerebral perfusion imaging may therefore play a role in selecting those patients with a carotid occlusion at high risk for future stroke who may benefit from bypass surgery.16
Hemodynamic Imaging
Imaging of cerebral hemodynamics in chronic carotid artery disease is focused on assessing the effect of the steno-occlusive lesion upon cerebral circulation and metabolism. The changes that occur at the brain tissue level can be discriminated in four separate stages of hemodynamic compromise.17,18 An obstructive lesion in one of the brain-feeding arteries will lead to a reduction of the arterial perfusion pressure distally to the steno-occlusive lesion.17 The reduced cerebral perfusion pressure induces initially collateral blood flow recruitment from alternative flow pathways to the supply territory of the affected brain-feeding artery. During this initial phase, cerebral blood flow (CBF) and cerebral blood volume (CBV) are kept relatively stable. The first stage of hemodynamic impairment occurs when collateral blood flow recruitment is insufficient to sustain cerebral perfusion. Autoregulative vasodilatation of the terminal arterioles occurs in order to sustain normal perfusion by reducing the vascular resistance to arterial inflow in the affected brain tissue regions. This results in an increase in CBV, while CBF may decrease by up to 18%. Stage two of hemodynamic compromise occurs
when the vasodilatory capacity is exhausted. At this stage, CBF will decrease passively with any further decline in perfusion pressure. In order to sustain oxygen metabolism, the oxygen extraction fraction will increase to its maximum. A further decline leads inevitably to a drop of the cerebral metabolic rate of oxygen, referred to as stage three, potentially resulting in cellular ischemia and permanent brain tissue damage.
when the vasodilatory capacity is exhausted. At this stage, CBF will decrease passively with any further decline in perfusion pressure. In order to sustain oxygen metabolism, the oxygen extraction fraction will increase to its maximum. A further decline leads inevitably to a drop of the cerebral metabolic rate of oxygen, referred to as stage three, potentially resulting in cellular ischemia and permanent brain tissue damage.
Measuring the Autoregulative Status
Several strategies have been developed to assess the extent of hemodynamic impairment in chronic carotid artery disease. During the first stage of hemodynamic impairment, baseline perfusion measurements such as CBF and CBV are relatively normal. Autoregulative vasodilatation of the small arterioles sustains cerebral perfusion in this phase by reducing the vascular resistance to arterial inflow. Here, the degree of the autoregulatory response can be assessed indirectly by measuring the dilatory response to a vascular challenge that dilates the cerebral vessels. The status of the cerebral autoregulation is typically expresses as cerebrovascular reactivity (CVR), which may be calculated as a percentage of increase of CBF at baseline to CBF during or after the vasodilatory challenge. The effect can be measured either at the brain tissue level, with techniques like positron emission tomography, single-photon emission tomography, and arterial spin labeling perfusion magnetic resonance imaging (MRI), or by measuring the increase in flow velocity in the middle cerebral artery with transcranial Doppler (TCD).10,11,19
There are a few different methods available to administer a vasodilatory challenge. Vasodilatation of the cerebral arterioles is generally accomplished by having an increase of local carbon dioxide (CO2) levels. The challenge can therefore be as simple as having the patient hold his or her breath.20 A more complex system can also be employed to administer CO2 through inhalation in either a uncontrolled or controlled fashion, respectively, via free-breathing circuit or a rebreathing circuit.20,21 Another suitable candidate as a vasodilatory challenge is administration of acetazolamide (Diamox).22,23,24 Acetazolamide penetrates the blood–brain barrier and inhibits carbonic anhydrase, which has been detected widely in cerebral tissue, thus inducing acidosis with resulting blockade of the vasoreaction to CO2. Nevertheless, administration of acetazolamide induces a considerable increase in CBF similar to that evoked by CO2 inhalation, which can be attributed to vasodilatation of the cerebral arteries, small vessels, and arterioles due to a decrease in tissue pH.22 A dose of 16 mg/kg was found to give an optimal vasodilatory response without causing unnecessary discomfort for the patient, although care must be taken to ensure maximum action of the drug in patients.25,26 Serious adverse events need not be expected; in a series of more than 1,000 examinations with acetazolamide, no acute ischemic complications were reported.27
All these vascular challenge methods have advantages and disadvantages, which may depend, among other factors, on the experience of a particular imaging site with a specific method to measure cerebrovascular reactivity. Still, limited studies are currently available that perform head-to-head comparisons between the different vasodilatory challenge methods.20,23 The advantage of acetazolamide is the long duration of the effect and the relatively straightforwardness of administering acetazolamide during the MRI protocol without the requirement of satisfying patient compliance (breath-holding), the need for using a mask (CO2 challenge), or an even more complex setup (CO2 rebreathing circuit). An example protocol for a vasodilatory challenge study, using arterial spin labeling perfusion MRI, is shown in the Geek Box.
Imaging Modalities
From the first method of measuring global brain perfusion in 1948 with nitrous oxide to the development of the first cross-sectional imaging method in the 1980s, positron emission tomography in the human brain, there have been vast improvements in imaging brain perfusion.28,29 The most commonly used techniques in current clinical practice for assessing cerebral perfusion in patients suffering from carotid artery disease are bolus passage CT (Chapter 9), gadolinium-based dynamic susceptibility contrast (Chapter 12) and dynamic contrast enhanced (Chapter 23) MRI, and TCD.30 As discussed in the specified chapters of this book, the different modalities each have their own individual strengths and weaknesses for imaging brain tissue perfusion and measuring the autoregulative status.
Transcranial Doppler
TCD is widely used for measuring the flow velocity through the brain’s blood vessels. Despite the fact that measurements may not be representative for the whole hemisphere, the main advantage of TCD is that it allows repeated and noninvasive investigation of flow. In patients with chronic carotid artery disease, measurements of the middle cerebral artery are most frequently performed because this is primarily fed through the ICA.23,24,25,27 The flow velocity (FV) as measured with TCD cannot be easily translated into volume of blood flow, however, additional hemodynamic information may be derived from the TCD waveform. The Gosling pulsatility index (PI) is the most commonly used hemodynamic index.31,32 It is the ratio between the mean velocity and the difference between the systolic and diastolic FV. A greater PI is attributed to a higher distal CVR, and
nowadays the PI is used as an indicator for CVR.33,34,35 The PI is, however, not only dependent on CVR, but also on other factors such as the heart rate, amplitude between diastolic and systolic pressure, cerebral perfusion pressure, and vascular resistance. The PI can be assessed quickly, but it is not an accurate estimate of the CVR, because it is codependent on multiple other hemodynamic variables and is therefore a more accurate description of cerebral perfusion pressure rather than CVR.36
nowadays the PI is used as an indicator for CVR.33,34,35 The PI is, however, not only dependent on CVR, but also on other factors such as the heart rate, amplitude between diastolic and systolic pressure, cerebral perfusion pressure, and vascular resistance. The PI can be assessed quickly, but it is not an accurate estimate of the CVR, because it is codependent on multiple other hemodynamic variables and is therefore a more accurate description of cerebral perfusion pressure rather than CVR.36
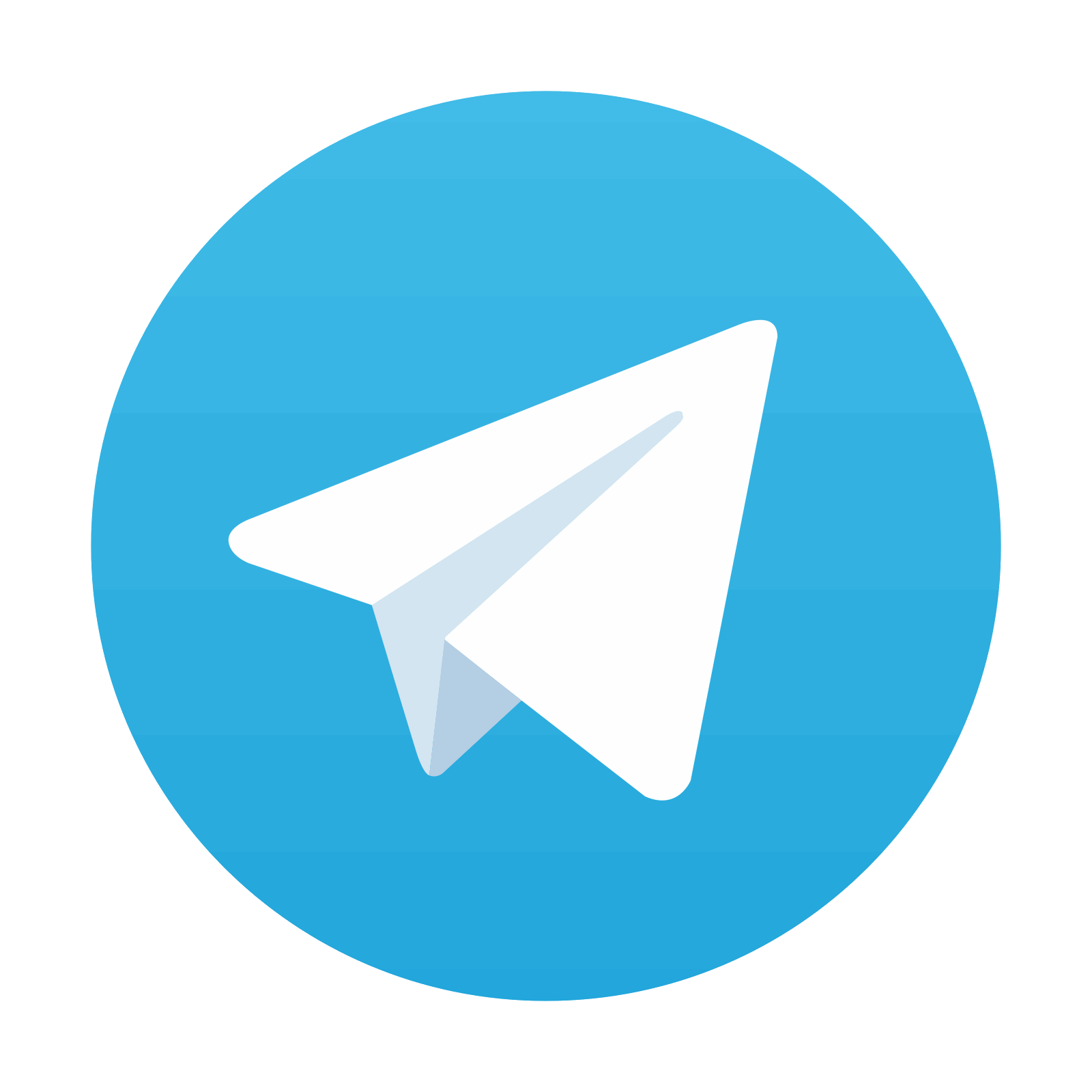
Stay updated, free articles. Join our Telegram channel

Full access? Get Clinical Tree
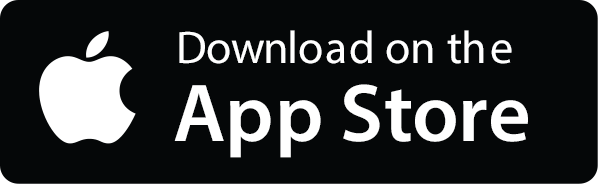
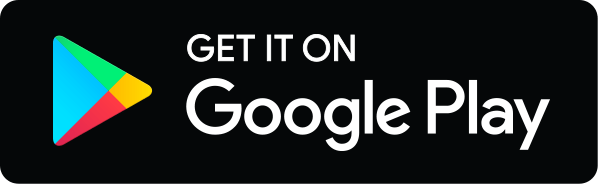
