Perfusion in Pharmacologic Imaging
Fernando O. Zelaya
María A. Fernández-Seara
Kevin Black
Steven C.R. Williams
Mitul A. Mehta
Pharmacokinetic imaging is a term used to broadly describe the use of imaging techniques to study the spatial and temporal dynamics of the effects of drugs on the human brain. In recent years, it has been simplified into the more general name of pharmacologic imaging, which refers simultaneously to the observation of dynamic and nondynamic (snapshot) information on drug action. This chapter will focus on the application of arterial spin labeling (ASL) to the study of drug action in the human brain.
Positron emission tomography (PET) has traditionally been used as the tool of choice for these types of investigations, either by measuring the effects of drug administration on cerebral blood flow (CBF) using radioactively labeled water (H215O)1,2 or by obtaining images of the specific sites of drug-induced activation by means of radioactively labeled probe molecules (ligands) that attach to the receptor sites targeted by the drug itself.3
In 2001, Jenkins4 recognized that the blood oxygen level–dependent (BOLD) contrast present in gradient echo–recalled magnetic resonance images (MRI) could be exploited to detect time-dependent effects of drug activation by collecting a temporal series of images during the administration of a psychoactive drug. Jenkins coined the term phMRI to refer to this methodology. The temporal change of the signal could be modeled using standard analysis techniques to identify brain areas whose signal varied with the pharmacokinetic profile of the drug,5,6 with a time-dependent behavioral measure affected by the drug,7,8 or with a predefined template or model.9 This technique has established itself as a powerful alternative to PET, particularly in preclinical imaging studies.
Although powerful in terms of noninvasiveness and methodologic flexibility, phMRI can be affected by the nonspecificity of the BOLD signal to a single physiologic parameter and with low-frequency sources of signal contamination, such as scanner drift, thermal noise, subject movement, and respiration. In clinical investigations, this method is also affected by the difficulty of identifying a reference signal that is not influenced by neuronal effects. More important, a model is commonly needed to analyze the temporal series data with respect to a known property of the drug.
The advent of ASL offered for the first time the opportunity to investigate drug action using a contrast-free MRI method that was directly sensitive to regional CBF in a manner analogous to 15O-labeled water PET scans. Unlike PET, ASL is entirely noninvasive, and because of this factor, it can be used in a serial fashion to obtain short- and long-term pharmacodynamic information on the effects of the drug upon brain activity. This chapter will describe the properties and advantages of ASL for pharmacologic imaging, present data from recent studies, and describe some of the limitations of the technique.
Cerebral Blood Flow and Neuronal Activity
Generating images of the regional distribution of CBF in humans became relevant to the study and the development of psychoactive drugs because of the well-established relationship that regional CBF has with the level of neuronal activity changes in human brain.10,11,12 This relationship is largely based on a phenomenon known as neurovascular coupling, which broadly encompasses the processes by which regional CBF is regulated at a local level in response to increases and decreases in neuronal activity.13 Although the precise molecular mechanisms of neurovascular coupling have not been fully elucidated, a vast amount of research carried out in the past few years has contributed to the establishment of the role that astrocytes play in the regulation of blood flow and the primary substances that control dilation and constriction of the smooth muscle wall of arterioles.14 In addition, recent investigations have uncovered the role played by pericytes and their ability to control the diameter of capillaries, demonstrating the specialized nature of the control of blood flow in the human brain and reiterating the intimate link between regional CBF and neuronal activation. The interrelationship of these two processes has been further reinforced by recent studies,15,16 which have demonstrated that increases in CBF in response to increases in neuronal activity (by direct sensory stimulation) occur with an onset delay of approximately 300 milliseconds or less, faster than previously foreseen and, again, reinforcing the relevance of CBF as a surrogate but directly connected signature of neuronal function.
These results have also contributed to the enhanced the interest of the pharmaceutical industry on techniques like ASL (in both clinical and preclinical investigations) because in many instances in the early stages of development of new drugs, it is important to establish evidence of drug penetration into the central nervous system.
Advantages of Measuring Regional Cerebral Blood Flow Using Arterial Spin Labeling
As stated previously, reasons for the need to assess this parameter stem from well-documented neuronal as well as vasoactive effects of several psychoactive compounds and the need to perform repeated measures studies in which an assessment of the variance in the baseline physiologic state across subjects is desirable. The main advantages of the ability to perform measurements of CBF for the study of drug action can be summarized as follows:
The ability to noninvasively determine global effects of the drug as well as baseline brain function (i.e., prior to drug administration in the absence of a cognitive or sensory paradigm). If performed in combination with task-evoked or resting-state BOLD examinations, the interpretation of changes in the BOLD signal can also be enhanced by the availability of data on the intrinsic physiologic effects of the compound.
Determination of the baseline neurophysiologic state, prior to drug administration, in repeated measures designs of blood flow or BOLD changes.
Determination of absolute quantitative changes in a single physiologic parameter, elicited by the drug (unlike BOLD), which is sensitive to both regional CBF and the regional rate of oxygen metabolism.
Assessment of time-dependent effects of the drug. This is not possible with radioactive tracers owing to the invasive nature of those techniques and the limited half-life of many of the tracers. It is also extremely difficult to perform similar investigations by means of electroencephalography in prolonged examinations because of the artifacts caused by continued removal of the electroencephalograph cap and is potentially complicated using BOLD data owing to its sensitivity to low frequency fluctuations.
Determination of drug effects independent of context-driven interpretations such as when using BOLD functional MRI (fMRI). This offers particular advantages for the assessment of novel analgesics, antidepressants, and others. In such cases, the interaction of the affective state of the subjects and the drug are likely to be long lasting (e.g., chronic pain and mood states) and can also be affected by presentation of affective stimuli, such as pictures of subjects in pain, emotional faces, and so forth. The potential disadvantage of employing only task-driven BOLD fMRI in those instances is that changes in activation between two treatment conditions (e.g., drug vs. placebo) could have a physiology-related component whose magnitude would be difficult to ascertain.
In combination with BOLD fMRI and a suitable calibration paradigm, ASL can be used to determine changes in cerebral metabolic rate of oxygen.
Noncontrast, noninvasive MRI examinations such as ASL are inexpensive compared with PET.
ASL offers special advantages in study designs in which conditions change at a very low frequency (e.g., learning paradigms where learning occurs over minutes or more) and in those in which drug administration occurs over a long period of time. This reduced sensitivity to extraneous sources of low frequency noise stems from the continued subtraction of control and labeled images. This feature is of particular advantage when investigating the effects of orally administered drugs.
Arterial Spin Labeling Methodology
As stated in another chapter, in ASL, regional information on the rate of blood transport through the brain tissue (CBF or cerebral perfusion) is encoded by labeling (or tagging) the MR signal of arterial blood over a region proximal to the image volume by means of a selective inversion of its signal amplitude. The effect of the labeled blood is measured by collecting the images and comparing the voxel-wise signal intensities with those obtained in the absence of labeling (i.e., control image or nontagged image). Subtraction of consecutively acquired tagged and nontagged image pairs yields a voxel-wise signal difference proportional to the rate of perfusion of the labeled blood at each voxel that can be converted to physiologic units using information from one additional scan.17,18
The choice of ASL methodology for pharmacologic imaging studies is linked to the speed of data collection and the pharmacokinetics of the compound under study. ASL techniques are divided into two principal categories, based on a distinction between two methods for labeling arterial blood.
First, in pulsed ASL, labeling of arterial blood is achieved by inverting the signal over a relatively wide region (∼100 mm) in the vicinity of the carotid arteries. This is achieved with spatially selective radiofrequency pulses whose B1-field profile is homogeneous over a wide region, for example, hyperbolic secant and other specially tailored pulses.19,20,21 The contrast-to-noise ratio of this approach is partly driven by the large number of arterial spins inverted by the pulse. The image data are commonly collected later using a single-shot acquisition technique such as echo-planar imaging. Because data collection is achieved with a relatively short repetition time (∼2 seconds), the temporal series of the perfusion-weighted acquisition can be used for analysis in a manner similar to phMRI22; alternatively the series of control-label pairs can be broken into an arbitrarily selectable number of averages to produce a succession of resting state CBF maps that
contain information on the evolution of drug-induced CBF changes. This approach has been employed to evaluate CBF changes in response to rapid injection of opioid substances.23,24
contain information on the evolution of drug-induced CBF changes. This approach has been employed to evaluate CBF changes in response to rapid injection of opioid substances.23,24
Second, in continuously labeled ASL or pseudo-continuously or pulsed-continuously labeled ASL, arterial blood is labeled by flow-driven inversion of arterial blood in the presence of a magnetic field gradient, which is applied in the direction of the moving blood (inferior to superior). As the blood flows along the direction of the gradient, a relatively low amplitude radiofrequency pulse of 1 to 2 seconds’ duration is applied over an infinitesimally thin plane located (as much as possible) in the optimum region of the carotid supply. The flow of arterial spins along the gradient changes their Larmor frequency in a continuous manner and induces a rotation of the longitudinal magnetization vector about the effective field, which in turn changes the orientation of the spins by 180 degrees25,26 as the arterial spins cross the labeling plane. This method is extremely effective at labeling a substantial amount of arterial blood, but because it requires a longer repetition time between acquisition of the images (4 to 6 seconds), it is well suited for the study of drugs that act more slowly in the brain. The superior efficiency of labeling also allows for the collection of image data using multishot (segmented) acquisition techniques, such as three-dimensional spiral fast spin echo or three-dimensional gradient and spin echo.27,28
Summary of Some Recent Applications
ASL has been used in at least two studies to investigate the effects of alcohol administration on CBF. Tolentino et al.29 investigated the differences in CBF changes induced by an alcohol dose on low and high responders to alcohol. Increases in CBF were found in up to five frontal regions, but these increases were lower in those with a higher tolerance to alcohol (low responders). These differences persisted even after carefully controlling for gray matter masking to minimize white matter contributions and confirming that the differences between alcohol and placebo were not the result of a low signal-to-noise ratio (SNR) on placebo. In a separate investigation, Rickenbacher et al.30 evaluated gender differences in the effects of alcohol on CBF. Bilateral frontal increases were found in men but not in women.
Carhart-Harris et al.31 investigated the effects of the hallucinogen psilocybin and observed significant decreases in CBF in the thalamus and posterior and anterior cingulate cortex (PCC and ACC). The decreased CBF in the ACC predicted the intensity of subjective effects. These effects were not influenced by changes in end tidal CO2, suggesting that CBF is at least in this case a marker of neuronal activity changes induced by the drug and independent of factors that ubiquitously affect vasodilation or constriction. These results opened the possibility of employing ASL to characterize antipsychotic drugs.
Fernandez-Seara et al.32 investigated the effects of metoclopramide, a dopamine receptor antagonist used in the treatment of nausea and vomiting. In addition, they determined the effects of the drug on arterial blood velocity in the internal carotid and vertebral arteries using phase-contrast velocity mapping MRI, as a way to control for the effects of the drug on global CBF and to evaluate potential changes in labeling efficiency between physiologic states. The drug caused increased CBF in bilateral putamen (Fig. 72.1), consistent with the elevated density of dopamine receptors in that structure and consistent with its motor side effects.
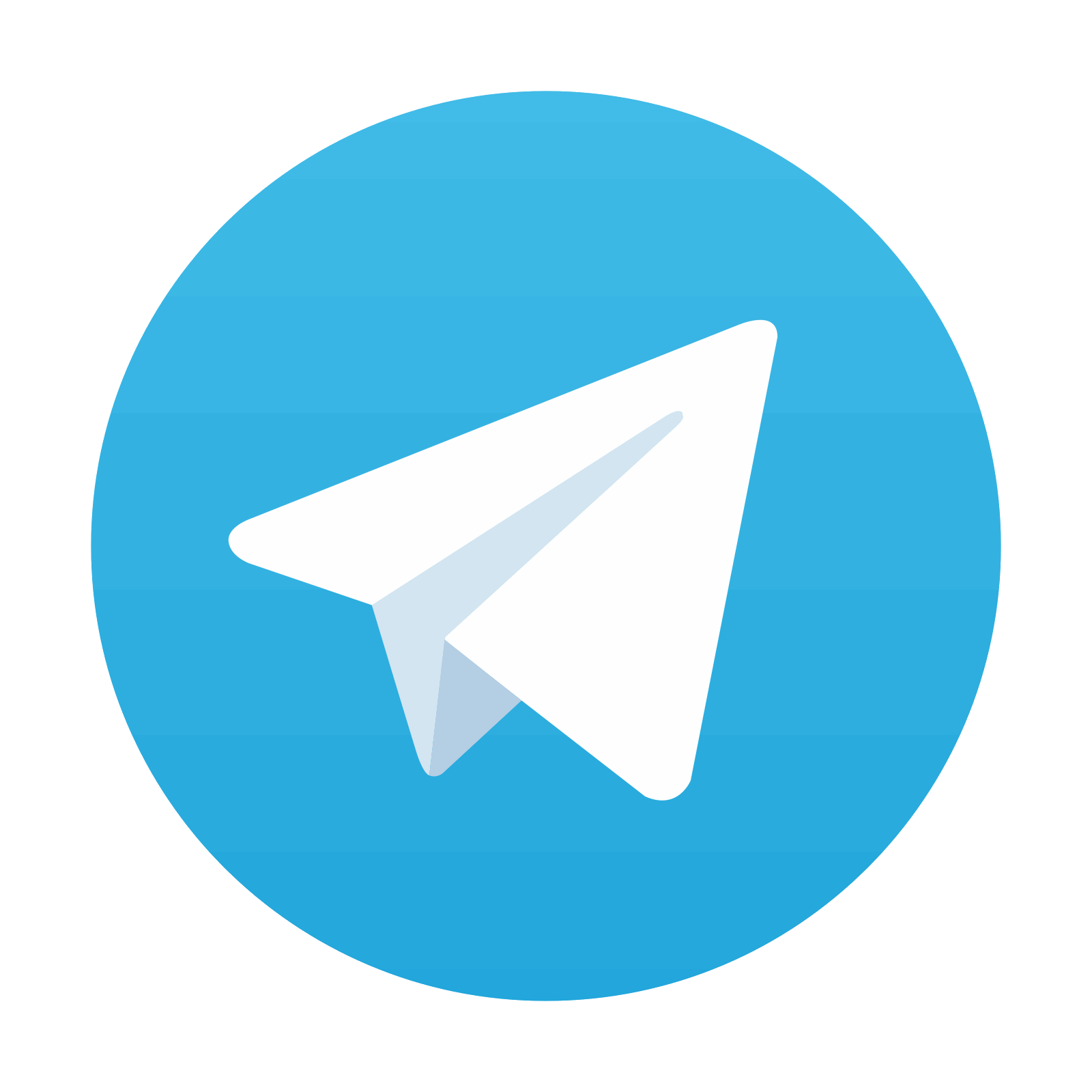
Stay updated, free articles. Join our Telegram channel

Full access? Get Clinical Tree
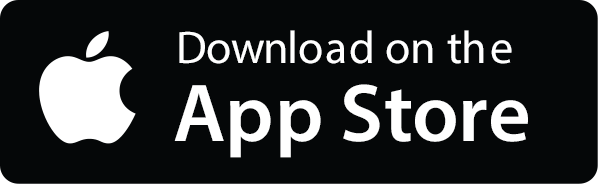
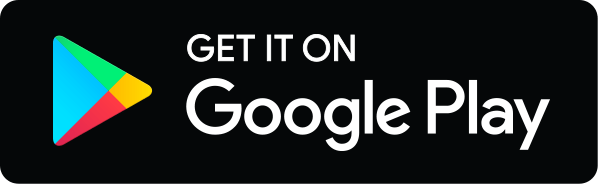