Since the introduction of contrast-enhanced MR angiography (MRA), several different techniques for imaging the peripheral arteries have evolved. All of them provide good diagnostic image quality, whereas some older techniques suffer from drawbacks, such as long acquisition time, impaired image quality from venous enhancement, and limited spatial resolution. MRA provides the most comprehensive modality offering the ability to tailor the examination to the patient and the specific question to be answered. The drawbacks experienced at the introduction of MRA to clinical routine have largely been overcome or at least diminished, so that the benefits of MRA outbalance the limitations.
- •
Since the introduction of contrast-enhanced MR angiography (MRA), several different techniques for imaging of the peripheral arteries have evolved. All provide good diagnostic image quality, whereas some older techniques suffer from drawbacks, such as long acquisition time, impaired image quality from venous enhancement, or limited spatial resolution.
- •
Other techniques, such as CT angiography, digital subtraction angiography, and ultrasound, are superior to MRA in terms of spatial and/or temporal resolution. However, this superiority is only in certain anatomic areas or under certain circumstances.
- •
MRA provides the most comprehensive modality allowing the examination to be tailored to the patient and the specific question to be answered.
Introduction
Cardiovascular disease, with its major manifestations of coronary heart disease, cerebrovascular disease/stroke, and peripheral artery disease (PAD), still represents the leading cause of death in the United States. Approximately 83 million American adults have one or more types of cardiovascular disease; 50% of these are older than 60 years of age. The prevalence of asymptomatic PAD lies between 3% and 10% in the general population, increasing to 15% to 20% in persons older than 70 years. Additionally, the prevalence of symptomatic PAD is approximately 3% in patients aged 40 years and 6% at 60 years. However, these data might underestimate the total prevalence of cardiovascular disease, because screening studies showed that between 10% and 50% of all patients with intermittent claudication never consult a doctor about their symptoms. Besides family history of PAD, smoking is the most severe risk factor. Smokers are 3 times more often affected than nonsmokers. Furthermore, PAD is directly associated with arterial hypertension and diabetes mellitus, and is the most common cause of nontraumatic limb amputation in the industrialized world, and thus an important economic factor in health care in the western world.
The first clinical test performed to evaluate the presence or absence of PAD is calculation of the ankle brachial index (ABI), which is the ratio of the systolic pressure in the posterior tibial or dorsalis pedis artery divided by the systolic blood pressure in the brachial artery. A resting ABI of 0.90 or less correlates with a hemodynamically significant arterial stenosis, and therefore the ABI is often used to determine if suspected PAD is hemodynamically relevant. In symptomatic individuals, an ABI 0.90 or less is approximately 95% sensitive in detecting arteriogram-positive PAD and almost 100% specific in identifying healthy individuals. Thus, this test is well suited for identifying individuals with PAD but is nonspecific for the exact localization of pathologic vascular lesions. Hence an imaging modality that provides detailed information about the location and extent of vascular disease is of great interest.
Because digital subtraction angiography (DSA) has the highest spatial and temporal resolution compared with other imaging modalities and is the only technique that provides both diagnostic imaging and the opportunity of interventional procedures, it is still regarded as the standard of reference. However, the well-known disadvantages of DSA, including risks associated with the invasive procedure itself, ionizing radiation, and potentially nephrotoxic contrast agents, have led to a substantial change in the radiologic approach to the diagnosis of PAD in the past few years. CT angiography (CTA), MR angiography (MRA), and ultrasound, including Doppler techniques, can serve as noninvasive substitutes. The latter can provide high spatial resolution and dynamic information and vessel wall imaging in certain anatomic regions. However, other vascular territories are not accessible with ultrasound because of surrounding structures that impair imaging. Additionally, ultrasound in general suffers from its dependency on experienced examiners and patient preparation. CTA and MRA both feature user-independent noninvasive cross-sectional imaging of the entire arterial vasculature from head to toe. However, CTA suffers from the drawback of ionizing radiation and the need for potentially nephrotoxic contrast agents, and in some cases the influence of dense vessel wall calcifications on image analysis and rendering.
MRA, compared with other techniques, features the lowest spatial resolution but can provide dynamic information and vessel wall imaging with high soft tissue contrast without the definite need for contrast agents. However, the MR contrast agents are in general regarded as safe for the kidneys in patients with sufficient renal function (glomerular filtration rate >30 mL/min per 1.73 m 2 ) and are thus used in most MRA applications. Recently, some MRA contrast agents have been implicated in the development of nephrogenic systemic fibrosis in patients with significant renal insufficiency. Therefore, new noncontrast MRA techniques have been developed to provide MRA images without the need for contrast agents. Recent technical developments, including the introduction of new image reconstruction algorithms and dedicated contrast agents, have pushed the limits of MRA toward higher spatial resolution and image quality and have enabled time-resolved imaging. The combination of multichannel MR systems, high field strength, multielement receiver coils for angiography and dedicated contrast agents has helped to substantially minimize most of the traditional drawbacks of MRA.
Contrast agents for MRA
Today, all contrast agents approved for MRA, or at least used for MRA, are gadolinium-based. However, these agents can be differentiated in several ways; besides relaxivity, the 2 most important ways depend on their molecular structure and their interaction with blood components. Diagnostic MRA examinations can be created with every gadolinium-based contrast agent (GBCA); however, the molecular structure, and therefore the characteristics of a GBCA, are of clinical importance in terms of resulting image quality. In addition, the molecular structure may be of significance in terms of molecular stability and the risk of releasing free gadolinium, which is highly toxic and seems to be the trigger for development of nephrogenic systemic fibrosis. The 2 structures present today are linear and cyclic molecules, although cyclic agents seem to be more stable than linear agents. The latter again can be divided into ionic and nonionic agents, although the ionic agents are regarded as more stable than the nonionic agents. In terms of interaction with blood components, most agents do not show any binding to blood components, specifically proteins (human serum albumin [HSA]). However, some agents feature at least a temporary linkage to blood proteins, which leads to a prolonged presence of contrast agent within the blood vessels because of a restricted transfer of contrast agent from the vessels to the surrounding tissue ( Table 1 ).
Linear | Cyclic | |||
---|---|---|---|---|
Ionic | Nonionic | Ionic | Nonionic | |
No protein binding | Gadopentetate dimeglumine (Magnevist) | Gadodiamide (Omniscan) Gadoversetamide (OptiMark) | Gadoterate meglumine (Dotarem) | Gadobutrol (Gadavist/Gadovist) Gadoteridol (ProHance) |
Weak protein binding | Gadobenate dimeglumine (MultiHance) Gadoxetate disodium (Eovist/Primovist) | |||
Strong protein binding | Gadofosveset trisodium (Ablavar) |
The current standard contrast agent for imaging of the entire body and vasculature is a 0.5-mol/L extracellular gadolinium chelate without any protein binding. This type of contrast agent provides reasonable image quality for nearly all clinical indications of MRA. The advent of innovative new contrast agents with properties distinct from those of the traditional extracellular gadolinium agents promises to further expand the clinical application of contrast-enhanced MRA (CE-MRA). For some anatomic regions or indications, high-relaxivity contrast agents with higher concentrations of gadolinium chelates and/or an at least transient binding to proteins seem to be beneficial. These indications include imaging of the carotid or renal arteries or time-resolved applications.
Gadolinium chelates with protein binding feature a delayed passage or do not pass the vessel walls at all because of their size when bound to human serum proteins such as HSA. This effect helps make MRA more independent of the short-duration arterial first-pass imaging and allows for high spatial resolution at “steady state” (equilibrium state) with potentially prolonged acquisition times of up to several minutes.
Contrast agents for MRA
Today, all contrast agents approved for MRA, or at least used for MRA, are gadolinium-based. However, these agents can be differentiated in several ways; besides relaxivity, the 2 most important ways depend on their molecular structure and their interaction with blood components. Diagnostic MRA examinations can be created with every gadolinium-based contrast agent (GBCA); however, the molecular structure, and therefore the characteristics of a GBCA, are of clinical importance in terms of resulting image quality. In addition, the molecular structure may be of significance in terms of molecular stability and the risk of releasing free gadolinium, which is highly toxic and seems to be the trigger for development of nephrogenic systemic fibrosis. The 2 structures present today are linear and cyclic molecules, although cyclic agents seem to be more stable than linear agents. The latter again can be divided into ionic and nonionic agents, although the ionic agents are regarded as more stable than the nonionic agents. In terms of interaction with blood components, most agents do not show any binding to blood components, specifically proteins (human serum albumin [HSA]). However, some agents feature at least a temporary linkage to blood proteins, which leads to a prolonged presence of contrast agent within the blood vessels because of a restricted transfer of contrast agent from the vessels to the surrounding tissue ( Table 1 ).
Linear | Cyclic | |||
---|---|---|---|---|
Ionic | Nonionic | Ionic | Nonionic | |
No protein binding | Gadopentetate dimeglumine (Magnevist) | Gadodiamide (Omniscan) Gadoversetamide (OptiMark) | Gadoterate meglumine (Dotarem) | Gadobutrol (Gadavist/Gadovist) Gadoteridol (ProHance) |
Weak protein binding | Gadobenate dimeglumine (MultiHance) Gadoxetate disodium (Eovist/Primovist) | |||
Strong protein binding | Gadofosveset trisodium (Ablavar) |
The current standard contrast agent for imaging of the entire body and vasculature is a 0.5-mol/L extracellular gadolinium chelate without any protein binding. This type of contrast agent provides reasonable image quality for nearly all clinical indications of MRA. The advent of innovative new contrast agents with properties distinct from those of the traditional extracellular gadolinium agents promises to further expand the clinical application of contrast-enhanced MRA (CE-MRA). For some anatomic regions or indications, high-relaxivity contrast agents with higher concentrations of gadolinium chelates and/or an at least transient binding to proteins seem to be beneficial. These indications include imaging of the carotid or renal arteries or time-resolved applications.
Gadolinium chelates with protein binding feature a delayed passage or do not pass the vessel walls at all because of their size when bound to human serum proteins such as HSA. This effect helps make MRA more independent of the short-duration arterial first-pass imaging and allows for high spatial resolution at “steady state” (equilibrium state) with potentially prolonged acquisition times of up to several minutes.
Technical concepts for peripheral MRA
The main prerequisite for any diagnostic imaging modality of the arterial vasculature is to get high enough spatial resolution for reliable stenosis grading in combination with sufficient vessel contrast. In addition, dynamic information can be of great value for comprehensive imaging of diseased vasculature. Detailed vessel wall evaluation also potentially helps to further increase the image quality and specificity of an examination. An MRA dataset of high image quality features excellent signal-to-noise ratio and is free of venous enhancement. To reach this goal with MRA, several technical concepts evolved over time. Of all available methods, CE-MRA has evolved as the preferred technique for MR imaging of the arterial vasculature. CE-MRA can be performed in several different ways, and the contrast agent application scheme must be tailored to every CE-MRA technique ( Table 2 ).
MRA Technique | Author/Journal | Year | B 0 | Gadolinium-Based Contrast Agents | Total Dose | Injection Scheme |
---|---|---|---|---|---|---|
Bolus-chase | Czum et al, Journal of Magnetic Resonance Imaging | 2000 | 1.5T | Gadoteridol | 40 mL | 20 mL @ 0.5 mL/s 20 mL @ 1.5 mL/s |
Bolus-chase | Hany et al, Radiology | 2001 | 1.5T | Gadodiamide | 40 mL | 20 mL @ 1 mL/s 20 mL @ 0.5 mL/s |
Bolus-chase | Kramer et al, Investigative Radiology | 2007 | 3.0T | Gadopentetate dimeglumine | 20 mL | 10 mL @ 1.5 mL/s 10 mL @ 0.8 mL/s |
Bolus-chase | Maki et al, Journal of Magnetic Resonance Imaging | 2009 | 1.5T | Gadopentetate dimeglumine | 40 mL | 20 mL @ 1.8 mL/s 20 mL @ 1.4 mL/s |
Bolus-chase | Maki et al, Journal of Magnetic Resonance Imaging | 2009 | 1.5T | Gadofosveset | 0.05 mmol/kg | Calc. mL @ 0.7 mL/s |
Bolus-chase | Anzidei et al, Investigative Radiology | 2011 | 1.5T | Gadobenate dimeglumine | 15 mL | 10 mL @ 2 mL/s first pass 5 mL @ 0.5 mL/s steady state |
Continuous table movement | Kramer et al, Investigative Radiology | 2008 | 3.0T | Gadopentetate dimeglumine | 15 mL | 15 mL @ 1 mL/s |
Continuous table movement | Voth et al, Investigative Radiology | 2009 | 3.0T | Gadobutrol | 0.1 mmol/kg diluted 1:1 with saline | Calc. mL @ 1.5 mL/s |
Hybrid MRA | Meissner et al, Radiology | 2005 | 1.5T | Gadopentetate dimeglumine | 40 mL | 15 mL @ 1.5 mL/s calf 25 mL @ 2 mL/s abdomen + thigh |
Hybrid MRA | Berg et al, Investigative Radiology | 2008 | 3.0T | Gadodiamide | 35 mL | 10 mL @ 1 mL/s calf 15 mL @ 1 mL/s + 10 mL @ 0.6 mL/s abdomen + thigh |
Multiple injection | Hany et al, Radiology | 2001 | 1.5T | Gadodiamide | 38 mL | 10 mL @ 2 mL/s stat. abdomen 13 mL @ 0.5 mL/s dyn. thigh 15 mL @ 0.5 mL/s dyn. calf |
Multiple injection | Janka et al, Cardiovascular Interventional Radiology | 2006 | 1.5T | Gadopentetate dimeglumine | 35 mL | 10 mL @ 1.0 mL/s abdomen 15 mL @ 1.0 mL/s thigh 10 mL @ 1.0 mL/s calf |
CE-MRA
The basic principle of CE-MRA is to image gadolinium-enhanced blood. Thus, gadolinium arterial first pass must be synchronized with the acquisition of at least central k-space information to acquire an angiogram of the arterial lumen during the arterial phase. Peripheral parts of k-space are sampled not necessarily during peak arterial enhancement but rather should be while the injected contrast agent is present in the imaged field of view (FOV) to avoid artifacts (eg, edge blurring). However, injection of GBCA only leads to transient T1 shortening of the blood pool. Generally, enough contrast must be injected to decrease the T1 of blood to values smaller than those of stationary background tissues. After having briefly enhanced the arterial vasculature, most GBCAs rapidly diffuse into the extravascular extracellular space. Relative to peak arterial concentration, the intravascular half-life of commercially approved standard GBCA is approximately 90 seconds. Some dedicated GBCAs feature a longer intravascular half-life because of interaction with proteins in the blood pool. The major challenge in CE-MRA is balancing arterial first pass, spatial resolution, anatomic coverage, and data acquisition time.
Depending on the clinical question, imaging at one or multiple phases is recommended. Before GBCA is injected, a nonenhanced acquisition with exactly the same sequence settings as the contrast-enhanced scans should be acquired for later subtraction. Many of the primary clinical questions can be answered with only one postcontrast acquisition during the arterial first pass, especially for the larger proximal vessels. However, sometimes additional phases are needed, such as in the case of imbalanced filling of the arterial vasculature of both legs or to detect low flow collaterals. To get best results for peripheral MRA in terms of image quality, several different acquisition schemes were developed and are still used.
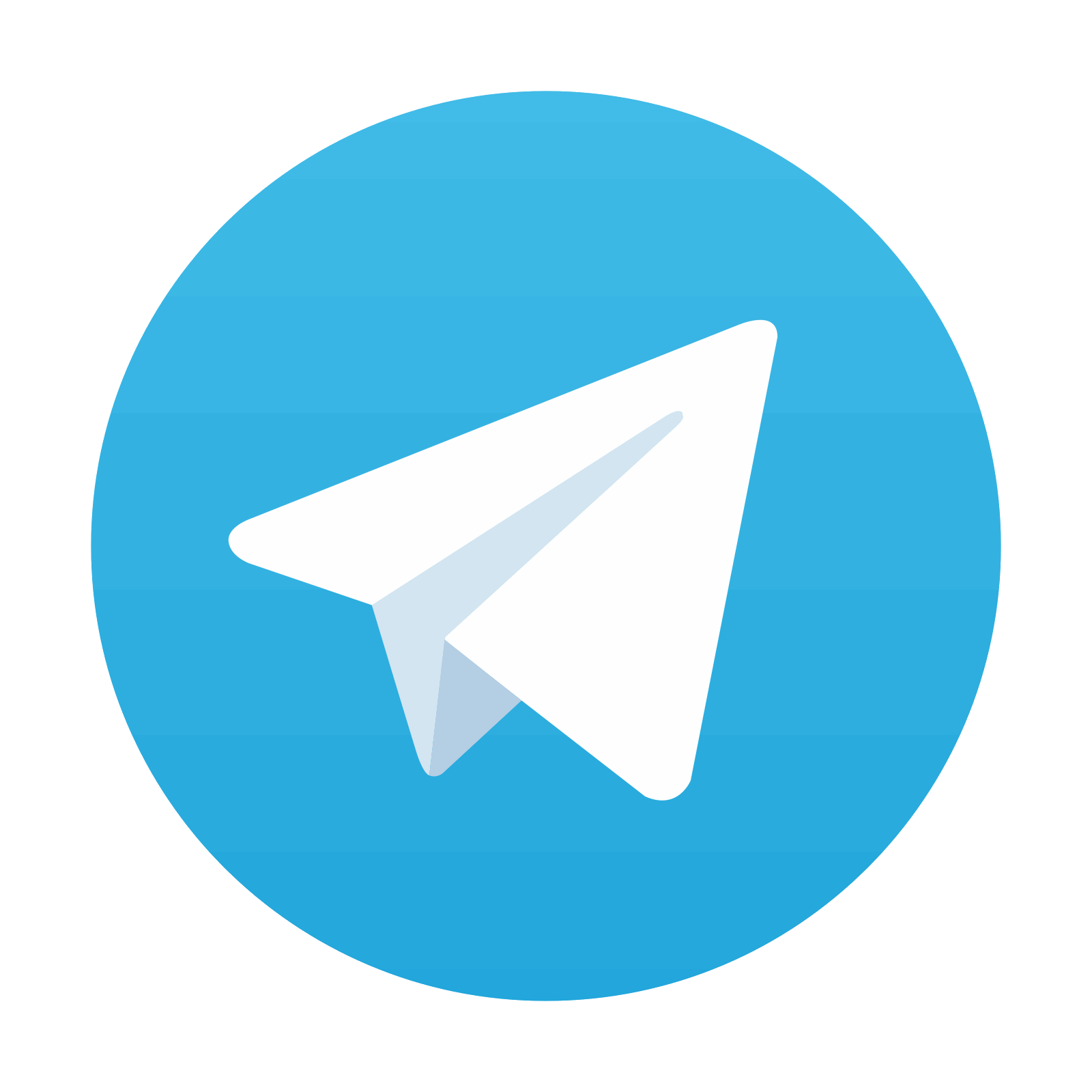
Stay updated, free articles. Join our Telegram channel

Full access? Get Clinical Tree
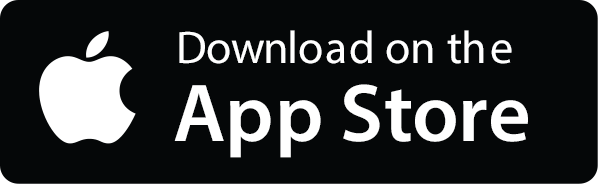
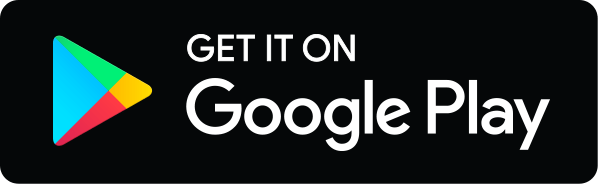