PET and PET/CT Imaging of Neuroendocrine Tumors
Richard P. Baum
Vikas Prasad
Neuroendocrine tumors (NET) are a heterogeneous group of neoplasms that are characterized by their endocrine metabolism and histology pattern. Several names such as carcinoid tumor, gastroenteropancreatic (GEP) tumor, islet cell tumor, neuroendocrine tumor, neuroendocrine carcinoma, and others have been suggested to cover the wide variety of tumor types belonging to NETs (1). The term carcinoid was first coined by Oberndorfer (2) in 1907 for endocrine tumors of the small intestine. Previously NETs have also been called APUD-oma (for amine precursor uptake, and decarboxylation) and were suspected to have their origin from the neural crest, which was later found to be inappropriate because the peptide secreting cells of the tumors are not of the neuroectodermal unit (3).
The latest research has shown that pluripotent stem cells or differentiated neuroendocrine cells are responsible for the origin of different tumor subtypes (4). Apart from the diverse histological variants, the variable clinical manifestations, and the (frequently) slow growth, NETs present a unique challenge for the physicians to diagnose and treat these tumors at an early stage (5,6,7).
The various compounds produced by these tumors, along with their characteristic symptoms, although sometimes useful in the diagnosis of the disease, do not provide adequate information to allow a clinician to determine a treatment regime. The conventional imaging modalities like ultrasound, endoscopy, computed tomography (CT) scan, and magnetic resonance imaging (MRI), although very useful for detecting the site of the primary tumor, the number of lesions, and other anatomical parameters, do not give the functional status of the tumor, which is often essential for defining the prognosis (4).
The discovery of overexpression of receptors for peptide hormones in cancerous tissue in the mid-1980s led to the gradual upsurge in the role of nuclear medicine procedures in the diagnostic algorithms for NET (8). Since then, various radiopharmaceuticals targeting these tumor-related receptors, as well as various metabolic pathways peculiar to the neuroendocrine tumor cells, have been designed and used with great success (9).
Incidence and Pathophysiology
The reported incidence of NET is low; however, because of the slow progression of many of these tumors, some patients may not get diagnosed during their lifetime. Traditionally, NETs are classified according to the site of origin (foregut, midgut, and hindgut), as tumors originating from the same site typically share functional properties, histochemistry, and secretory granules (3).
Based on histopathology, the World Health Organization (WHO) recently established a new classification system (10) (Table 8.21.1). Although NETs can occur at many sites in the body, the most common sites of origin are the GEP tract and the bronchus/lungs; other less common sites are the skin, the adrenal glands, the thyroid, and the genital tract (3). Neuroendocrine GEP tumors have been found to be derived from endodermal protodifferentiated stem cells (11) (Table 8.21.2). Approximately 70% of carcinoid tumors originating from gastrointestinal (GI) tissue are found in either the bronchus, jejuno-ileum, or colon/rectum (3).
Histology
NETs consist of monotonous sheets of small, round cell nuclei and are characterized by their propensity for avidly staining with silver.
Using immunohistochemical methods, typical markers of neuroendocrine tissues, like chromogranin, neuron-specific enolase (NSE), and synaptophysin can be detected. Electron microscopy reveals that these tumors possess numerous membrane-bound neurosecretory granules, which contain various hormones and biogenic amines (1).
Using immunohistochemical methods, typical markers of neuroendocrine tissues, like chromogranin, neuron-specific enolase (NSE), and synaptophysin can be detected. Electron microscopy reveals that these tumors possess numerous membrane-bound neurosecretory granules, which contain various hormones and biogenic amines (1).
Table 8.21.1 World Health Organization Classification of Endocrine Tumors | ||
---|---|---|
|
Clinical Manifestation
Based on clinical presentation, NETs can be broadly classified into functional and nonfunctional tumors. The functional tumors are characterized by the release of biogenic amines into the blood stream, which are responsible for producing characteristic clinical syndromes (3). The most common presenting symptoms of functional NETs are diarrhea, flushing, pain, asthma/wheezing, pellagra, and carcinoid heart disease like endocardial fibrosis (Hedinger syndrome). Approximately 33% to 50% of the NETs are nonfunctional. The symptoms present in this latter subset of patients with NET are largely related to the mass effect of the tumor, which varies by location. In many cases, these nonfunctional tumors are detected incidentally, often at a late stage when they have already metastasized.
The carcinoid tumors and tumors originating from the pancreas are mostly malignant in nature. Other than insulinomas, of which less than 10% are malignant, nearly all pancreatic NETs show malignant behavior. Among NETs of the GI tract, the highest incidence of metastases is found in jejuno-ileum tumors (58%), followed by lung/bronchus (6%) and rectum (4%). There are numerous factors determining the survival and the prognosis of patients; however, the presence of liver metastases is the single most important factor (6).
A correlation has been found between the size of the primary tumor and the chances of metastases in small intestinal carcinoids (3). Metastases to the liver occurred in 15% to 25% of tumors if the tumor diameter was less than 1 cm, in 58% to 80% if it was 1 to 2 cm, and greater than 75% if the tumor size was over 2 cm. These factors make it imperative to have a correct diagnostic algorithm before classifying a patient into a particular treatment regime (e.g., surgery, octreotide therapy, chemotherapy, or peptide receptor radionuclide therapy (PRRT).
Table 8.21.2 Origin of Gastroenteropancreatic Neuroendocrine Tumors | ||||||||||||||
---|---|---|---|---|---|---|---|---|---|---|---|---|---|---|
|
Diagnosis
A detailed history and thorough clinical examination are essential and represent the first steps in the diagnostic algorithm for NET. The characteristic history of flushing and intractable diarrhea necessitates the measurement of urinary or plasma serotonin or its metabolites in the urine. Other NET markers like chromogranin A and NSE are also useful. These markers are generally elevated in carcinoid tumors. If a patient is suspected of having a gastrinoma (history of abdominal pain, diarrhea, and gastroesophageal reflex disease [GERD]), the fasting gastrin level should be measured. If the diagnosis of insulinoma is suspected (recurrent hypoglycemia), C-peptide and serum glucose levels are determined and an elevated insulin level should be demonstrated under fasting conditions. For pheochromocytoma, metanephrines, catecholamines, and their metabolites should be measured in blood and in the urine (3). In patients with severe watery diarrhea and hypokalemia (Verner Morrison syndrome), vasointestinal polypeptide (VIP) should be measured in serum. Cutaneous alterations (erythema necrolyticum migrans) are typical for glucagonoma syndrome and should lead to the measurement of glucagon in serum.
Imaging
After the determination of biochemical markers, the next step in the diagnostic algorithm is to localize the site of the primary tumor. A CT scan of the thorax should be performed if a thoracic origin of the tumor is suspected (12). The role of ultrasound in the diagnosis of NET depends largely on the site of disease. Ultrasound has a high diagnostic sensitivity for the detection of liver metastases. If a gastric or a pancreatic primary tumor is suspected, primarily endoscopic ultrasound (EUS) should be performed. For the assessment of the vascularity of a tumor, color Doppler and power Doppler ultrasound are useful as well as a contrast-enhanced CT scan. Very commonly CT scans from the neck through the pelvic floor are performed.
For preoperative staging, MRI and CT (primarily contrast-enhanced CT) are used for the localization of the primary tumor and metastases. These modalities are also useful in the follow-up of patients after primary surgery. The accurate measurement of tumor size and extent of spread can be evaluated using three-dimensional reconstruction. MRI is the method of choice for the study of cervical masses and is specifically useful in intracranial and intraspinal lesions as well as to evaluate for bone marrow involvement (12).
In spite of giving valuable anatomical information about the tumors, these morphologically oriented imaging methodologies are of limited use in the diagnosis of an unknown neuroendocrine primary tumor and in defining the prognosis of NETs. This is where nuclear medicine, with its armamentarium of radiopharmaceuticals, excels over morphological imaging. One other significant advantage of nuclear medicine imaging techniques is the ability to perform a whole-body scan in a single study.
Radionuclide Imaging of Neuroendocrine Tumors
Molecular imaging using diverse positron emission tomography (PET) radiopharmaceuticals has gained a special role in the diagnostic work-up of patients with NET only in the past decade, although radionuclide imaging with single photon tracers has been in use for the diagnosis of NET (e.g., using indium-labeled somatostatin analogues) for nearly 20 years (13,14).
PET Radiopharmaceuticals
To utilize the full potential of molecular imaging for tumor diagnosis, a metabolic pathway or a genuine molecular event (e.g., receptor binding of a peptide) that is specific for a certain kind of tumor or tissue/cells may be targeted. The wide range of biogenic amines and peptides secreted, and their receptor expression by NETs, has been extremely useful for the detection of these tumors. One of the radiopharmaceuticals, metaiodobenzylguanidine (MIBG), labeled with iodine-131 ([131I]) (15) or with [123I], utilizes the structural resemblance of MIBG to norepinephrine (NE). Just like NE, MIBG is taken up by an active amine uptake mechanism by the cell membrane of sympathomedullary tissue and also by the intracellular granules, which results in prolonged retention of the radiopharmaceutical in NETs.
Iodine-123 is the preferred radionuclide for labeling MIBG because of its better physical imaging characteristics and the ability to perform single-photon emission computed tomography (SPECT), whereas [131I] MIBG can also be used for therapy. It is possible, however, that [124I] MIBG may offer advantages over [123I] MIBG in the coming years, but this requires further study.
Table 8.21.3 Drugs Interfering with Vesicular Monoamine Transporters, Potentially Leading to Wrong Interpretation of Iodine-123/131 Metaiodobenzylguanidine by Scintigraphy/SPECT | |||||||||||||||||||||||||||||||||||||||||||||||||||||||||||
---|---|---|---|---|---|---|---|---|---|---|---|---|---|---|---|---|---|---|---|---|---|---|---|---|---|---|---|---|---|---|---|---|---|---|---|---|---|---|---|---|---|---|---|---|---|---|---|---|---|---|---|---|---|---|---|---|---|---|---|
|
Special care has to be taken to withdraw certain drugs (Table 8.21.3) that may interfere with the MIBG uptake (by interacting with vesicular monoamine transport mechanism) in order to avoid false-negative results (16,17,18). Despite the high specificity of [131I] and [123I]-labeled MIBG, the inherent drawback of gamma camera imaging (relatively low resolution and difficult to quantify) necessitated the development of radiopharmaceuticals based on positron-emitting radiotracers.
The list of PET tracers currently being employed for the imaging of NETs is growing rapidly (Table 8.21.4). In order to understand and compare the results of this wide range of PET radiopharmaceuticals, it is essential to first describe their respective molecular targets.
The molecular events/targets that are currently being utilized for PET-based radiopharmaceuticals are: (a) somatostatin receptor expression, (b) serotonin production pathway, (c) biogenic amine storage, (d) catecholamine transport, (e) glucose metabolism, (f) and other miscellaneous peptide receptor expression.
Pharmaceuticals Based on Somatostatin Receptor Expression of Neuroendocrine Tumors
Intact monoclonal antibodies and other smaller fragments of antibodies have been used extensively in nuclear medicine for both diagnosis and therapy. Although the U.S. Food and Drug Administration approval of yttrium-90 ([90Y]) (e.g., Zevalin) and [131I] (e.g., Bexxar)
labeled anti-CD20 antibodies for the treatment of B cell non-Hodgkin’s lymphoma finally proved the success of radioimmunotherapy of hematological malignancies, the same is not true for imaging purposes because of the poor pharmacokinetics of whole monoclonal antibodies and the potential to generate an immune response. The discovery of overexpression of peptide receptors in various tumor cells in the 1980s opened a new chapter in the field of molecular imaging (8). Small peptides have better pharmacokinetic characteristics and no (or very low) antigenicity as compared to antibodies, making them nearly ideal ligands for receptor-based radionuclide imaging. Somatostatin, a cyclic peptide hormone, labeled with different radionuclides, is an example of such a peptide that has been used with high efficiency in the diagnosis of NETs.
labeled anti-CD20 antibodies for the treatment of B cell non-Hodgkin’s lymphoma finally proved the success of radioimmunotherapy of hematological malignancies, the same is not true for imaging purposes because of the poor pharmacokinetics of whole monoclonal antibodies and the potential to generate an immune response. The discovery of overexpression of peptide receptors in various tumor cells in the 1980s opened a new chapter in the field of molecular imaging (8). Small peptides have better pharmacokinetic characteristics and no (or very low) antigenicity as compared to antibodies, making them nearly ideal ligands for receptor-based radionuclide imaging. Somatostatin, a cyclic peptide hormone, labeled with different radionuclides, is an example of such a peptide that has been used with high efficiency in the diagnosis of NETs.
Table 8.21.4 Different Radiopharmaceuticals Currently Employed for the Diagnosis of Neuroendocrine Tumors | ||||||||||||||||||||||||||||||||||||||||||||||||||||||||||||||||||||||||||||||||||||||||||||||||||||||||||||||||||||||||||||||||||||||
---|---|---|---|---|---|---|---|---|---|---|---|---|---|---|---|---|---|---|---|---|---|---|---|---|---|---|---|---|---|---|---|---|---|---|---|---|---|---|---|---|---|---|---|---|---|---|---|---|---|---|---|---|---|---|---|---|---|---|---|---|---|---|---|---|---|---|---|---|---|---|---|---|---|---|---|---|---|---|---|---|---|---|---|---|---|---|---|---|---|---|---|---|---|---|---|---|---|---|---|---|---|---|---|---|---|---|---|---|---|---|---|---|---|---|---|---|---|---|---|---|---|---|---|---|---|---|---|---|---|---|---|---|---|---|
|
There are two naturally occurring bioactive forms of somatostatin: somatostatin-14 and somatostatin-28. Somatostatin receptors are normally expressed in different organs, such as the pituitary gland, thyroid, pancreas, liver, in the spleen (activated lymphocytes), and GI tract in different quantities. The primary action of this peptide hormone is the inhibition of hormone secretion and modulation of neurotransmission and cell proliferation through specific membrane bound G-protein-coupled receptors (19). These somatostatin receptors (SSTR) have generated wide clinical interest as they are expressed on various tumor types (20).
Table 8.21.5 Normal Organs/Tissues and Somatostatin Receptor Expression | ||||||||||||||||||||||||||||||||||||||||||||||||||||||||||||||
---|---|---|---|---|---|---|---|---|---|---|---|---|---|---|---|---|---|---|---|---|---|---|---|---|---|---|---|---|---|---|---|---|---|---|---|---|---|---|---|---|---|---|---|---|---|---|---|---|---|---|---|---|---|---|---|---|---|---|---|---|---|---|
|
Five different types of SSTR proteins have been cloned (SSTR 1 through 5). Recent studies revealed that SSTR 2 consists of two subtypes, SSTR 2a and SSTR 2b. Some of these receptors, especially SSTR 2, are overexpressed in NET, which forms the basis of peptide receptor imaging using radiolabeled somatostatin analogues (19). Of importance is also to understand the complex interaction between SSTR 2 and SSTR 5. Sharif et al. (21) have shown that the presence of SSTR 5 in the same cells modulates trafficking and cell surface regulation of SSTR 2a and cellular desensitization to the effects of the somatostatin (sometimes also known as somatotropin release-inhibiting factor). Prolonged treatment with Sandostatin LAR/s.c. (Novartis Oncology, East Hanover, New Jersey) may have some bearing on the imaging with radiolabeled somatostatin receptor ligands.
For defining the dosimetry of a radiopharmaceutical, and also for determining the potential cause of false-positive results, it is essential to know the expression of SSTR in normal and in nonmalignant tissues. Table 8.21.5 shows in detail the expression of SSTR
in different normal tissues (22,23,24,25,26,27,28). Of particular importance is the expression of SSTR on activated lymphocytes, macrophages, and fibroblasts. Most of the tumors that have been studied with radiolabeled somatostatin analogues mainly express SSTR 2. However, recent results have shown that SSTR 1 and SSTR 3, 4, and 5 are also expressed on many tumors (Table 8.21.6) with varying percentages of expression (23).
in different normal tissues (22,23,24,25,26,27,28). Of particular importance is the expression of SSTR on activated lymphocytes, macrophages, and fibroblasts. Most of the tumors that have been studied with radiolabeled somatostatin analogues mainly express SSTR 2. However, recent results have shown that SSTR 1 and SSTR 3, 4, and 5 are also expressed on many tumors (Table 8.21.6) with varying percentages of expression (23).
Table 8.21.6 Tumors with Proven Somatostatin Receptor Expression | ||||||||||||||||||||||||||||||||||||||||||||||||||
---|---|---|---|---|---|---|---|---|---|---|---|---|---|---|---|---|---|---|---|---|---|---|---|---|---|---|---|---|---|---|---|---|---|---|---|---|---|---|---|---|---|---|---|---|---|---|---|---|---|---|
|
Table 8.21.7 Affinity Profiles (IC50) of Somatostatin Receptor Subtypes for Different Somatostatin Analogues (the Smaller the Value, the Higher the Affinity) | ||||||||||||||||||||||||||||||||||||||||||||||||||||||||||||||||||||||||||||||
---|---|---|---|---|---|---|---|---|---|---|---|---|---|---|---|---|---|---|---|---|---|---|---|---|---|---|---|---|---|---|---|---|---|---|---|---|---|---|---|---|---|---|---|---|---|---|---|---|---|---|---|---|---|---|---|---|---|---|---|---|---|---|---|---|---|---|---|---|---|---|---|---|---|---|---|---|---|---|
|
SSTR 2 is expressed maximally (95%) in neuroendocrine tumors of midgut origin, followed by SSTR 1 (80%) and SSTR 5 (75%) (3). This observation has lead to the gradual modification of somatostatin analogue synthesis. Most of the currently used somatostatin radiopharmaceuticals (Table 8.21.7) are derivatives of octreotide, lanreotide, or vapreotide and show variable binding to SSTR (17,19,29,30).
The first radiolabeled somatostatin analogue to be approved for scintigraphy of NET was [111In]-DTPA-D-Phe1-octreotide ([111In]-pentetreotide; OctreoScan, Mallinckrodt, Inc, St. Louis, Missouri). Large clinical studies have shown that this radiopharmaceutical is well suited for the scintigraphic localization of primary and metastatic NETs (31,32). Molybdenum-99-technetium (99mTc)-labeled somatostatin analogues, such as 99mTc-depreotide (6-hydrazinonicotinic), 99mTc-vapreotide, 99mTc-P829, and 99mTc-EDDA-HYNIC-TOC, have also been used (32,33,34,35,36,37). Among these, only 99mTc-EDDA-HYNIC-TOC (or -TATE) has been shown in a larger patient population to be superior to [111In]-pentetreotide for the detection of SSTR-positive tumors and metastases (33).
In an effort to find a somatostatin analogue with higher affinity for SSTR receptors, the next generation of somatostatin analogues (e.g., DOTA-TOC [DOTA-D-Phe1-Tyr3-octreotide]) (16) were developed and labeled with different radionuclides for imaging as well as for therapy (38,39,40,41,42,43,44,45). Replacement of the alcohol group at the C-terminus of the peptide by a carboxylic acid group resulted in the formation of DOTA-D-Phe1-Tyr3-Thr8-octreotide (DOTA-TATE) and has been shown to have very high affinity for the SSTR 2 receptor, which even surpasses the binding affinity of natural somatostatin (29,46).
The binding affinity to other SSTR receptors is low (SSTR 5) or negligible (SSTR 3), and there is no significant affinity to SSTR 1 and SSTR 4 (19). Indium-111-DOTA-lanreotide (LAN; D-2-Nal-Cys-tyr-D-Trp-Lys-Val-Thr-NH2) has been postulated to be superior to [111In]-DTPA-octreotide in the detection of neuroendocrine pancreatic carcinoma (47). However, the claim that [111In]-DOTA-lanreotide targets SSTR 2 through 5 with higher affinity and SSTR 1 with lower affinity was not confirmed by Reubi et al. (29).
Next in the development of high-affinity somatostatin analogues was DOTA-NOC (DOTA-1-Nal3-octreotide), which was the result of amino acid exchange at position 3 of octreotide (Fig. 8.21.1). This compound has improved affinity for SSTR 2 and higher affinity to SSTR 3 and SSTR 5 (19), resulting in coverage of a wider spectrum of SSTR (pansomatostatin analogue). It is expected to have a significant effect on staging, diagnosis, and therapy of NETs and various other somatostatin receptor–expressing tumors. All these somatostatin analogues labeled with various radionuclides have been used for scintigraphy or PET of SSTR positive tumors with variable success. Other somatostatin analogues, which are in the preclinical stage of development, are DOTA-NOC-ATE [(DOTA-1Nal3,Thr8)-octreotide] and DOTA-BOC-ATE [(DOTA, BzThi3, Thr8)-octreotide]. Also radiolabeled with [111In], these “fourth-generation analogues” have been shown to have very high affinity for SSTR 2, SSTR 3, and SSTR 5 and intermediate high affinity to SSTR 4 (48).
SSTR antagonists, (NH(2)-CO-c(DCys-Phe-Tyr-DAgl(8)(Me,2- naphthoyl)-Lys-Thr-Phe-Cys)-OH (SST(3)-ODN-8) and (SST(2)-ANT) have also been labeled with [111In], and their superiority over SSTR agonists (in murine models) for in vivo targeting of SSTR 2 and SSTR 3 rich tumors, as shown by the group of Ginj et al. (49) and Reubi et al. (50), has resulted in the shift in paradigm, and they are now being contemplated for use in tumor diagnosis. The intrinsic superior efficiency and resolution of PET imaging favors the use of PET-labeled SSTR binding agents if the kinetics of the PET tracer are similar to those of the single photon emitter. Thus, both PET and SPECT SSTR binding agents will likely be used in practice.
Pharmaceuticals Based on the Serotonin Production Pathway
Most of the clinical symptoms of a neuroendocrine tumor are due to the excessive production of serotonin. The name serotonin was coined by Maurice M. Robert in 1948 and literally means “a serum
agent responsible for producing vasoconstriction.” It was later identified as 5-hydroxytryptamine (HTP). The precursor for the production of 5-hydroxytryptamine is tryptophan (Fig. 8.21.2). One of the intermediates in the production pathway, HTP has been labeled successfully with carbon-11 ([11C]) in a phase I clinical trial. After administration, the functionally active, serotonin-producing neuroendocrine tumor cells accumulate the radiopharmaceutical, leading to visualization on the PET images.
agent responsible for producing vasoconstriction.” It was later identified as 5-hydroxytryptamine (HTP). The precursor for the production of 5-hydroxytryptamine is tryptophan (Fig. 8.21.2). One of the intermediates in the production pathway, HTP has been labeled successfully with carbon-11 ([11C]) in a phase I clinical trial. After administration, the functionally active, serotonin-producing neuroendocrine tumor cells accumulate the radiopharmaceutical, leading to visualization on the PET images.
Pharmaceuticals Based on Biogenic Amine Production and Storage Mechanism
Neuroendocrine tumors are characterized by the production and storage of several biogenic amines. One radiopharmaceutical that has been designed based on this observation is [11C]- or fluorine-18 ([18F])-labeled L-dihydroxyphenylalanine (DOPA). The increase in the activity of L-DOPA decarboxylase is one of the hallmarks of NETs (51,52). The metabolic pathway leading to the production of dopamine by the decarboxylation reaction is shown in Fig. 8.21.3.
Pharmaceuticals Based on the Catecholamine Transport Pathway
The success achieved by MIBG encouraged scientists to develop PET radiopharmaceuticals based on the catecholamine transport mechanism. Pheochromocytoma, neuroblastoma and other chromaffin tumor tissues, due to their ability to produce epinephrine and norepinephrine, concentrate many synthetic amine precursors using catecholamine transporters. Carbon-11-epinephrine ([11C]-E) and [11C]-hydroxyepiphedrine (11C-HED) are catecholamine analogues and [18F]-fluorodopamine (18F-FDA) is a catecholamine precursor, developed by the National Institutes of Health. Like [123I]/[131I]-MIBG, these radiopharmaceuticals may also be prone to interference by drugs (Table 8.21.3), acting on vesicular monoamine transporters and the norepinephrine transporter (16).
Pharmaceuticals Based on Increased Glucose Metabolism
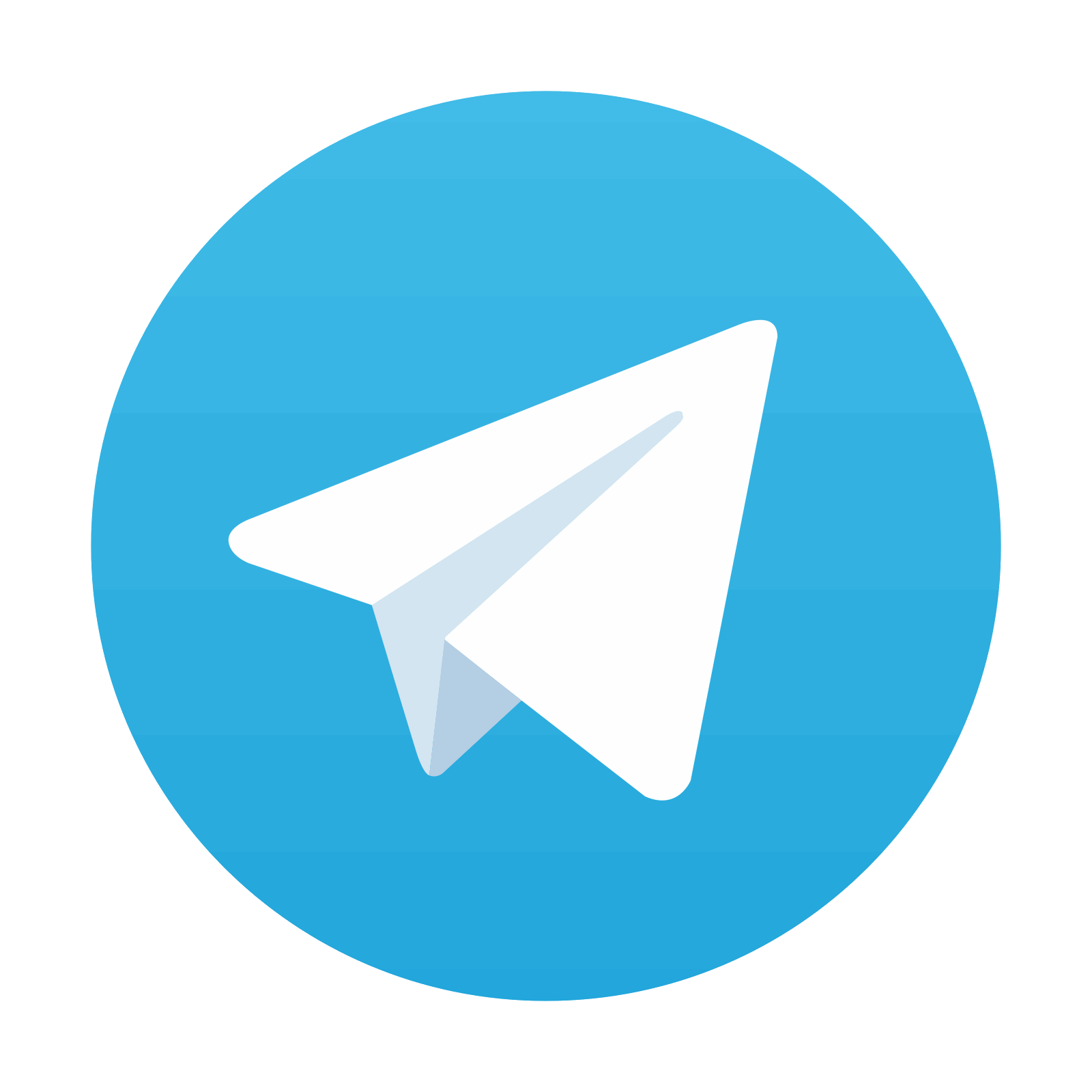
Stay updated, free articles. Join our Telegram channel

Full access? Get Clinical Tree
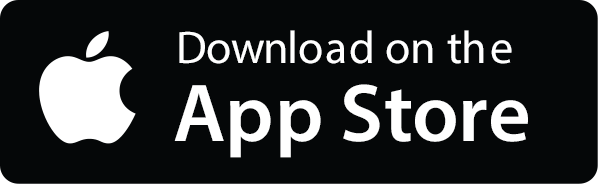
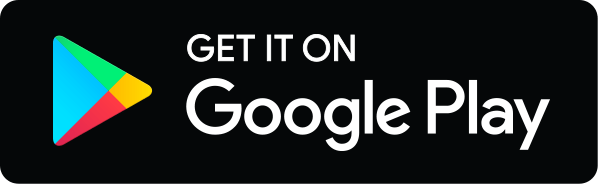
