27 The introduction of metabolic imaging into the radiation therapy planning (RTP) process is a major advance in the management of cancer patients. The impact can be seen in Conventional imaging techniques may up- or downstage patients with a variety of cancers. When radiation therapy is employed for optimal management of the diseased sites, understaging may result in partial treatment, and overstaging may lead to unnecessary radiation toxicity to the site(s) without disease. Positron emission tomography (PET) allows accurate staging not only at the primary site, but also at the locoregional level and distant regions. Staging with fluorodeoxyglucose (FDG) PET may change the intent of treatment from curative to palliative in 10 to 26% of cases when a new distant metastasis is detected. Several studies have documented that the improved staging with FDG PET can be used to improve patient management and significantly impact RTP (Table 27.1) and therefore improve outcome and minimize toxicity.1–4
PET and PET/CT in Radiation Therapy Planning
Sandip Basu, Guobin Song, Abass Alavi, and Eugene C. Lin
More Accurate Staging
Tumor Volume Delineation
Changes in Staging | Effect on Radiotherapy | |
T-stage | Larger extension of primary tumor (upstaging) | Enlargement of radiotherapy fields to avoid geographic miss |
Change of radiotherapy indication from curative to palliative | ||
Less extension of primary tumor (downstaging) | Decrease in radiotherapy fields and hence decrease in radiation exposure of normal tissues, thus allowing dose escalation | |
Change of radiotherapy indication from palliative to curative | ||
N-stage | Detection of new site of lymph node involvement (upstaging) | Enlargement of radiotherapy fields to avoid geographic miss Change of radiotherapy indication from curative to palliative |
Omission of enlarged lymph nodes, diagnosed as malignant on CT or MRI (downstaging) | Change of radiotherapy indication from palliative to curative Decrease in radiotherapy fields and hence decrease in radiation exposure of normal tissues, thus allowing dose escalation | |
M-stage | Detection of distant metastases | Change of radiotherapy indication from curative to palliative |
Source: From van Baardwijk A, et al. The current status of FDG-PET in tumor volume definition in radiotherapy treatment planning. Cancer Treat Rev 2006;32(4):245–260. Reprinted with permission.
Abbreviations: CT, computed tomography; MRI, magnetic resonance imaging; PET, positron emission tomography.
Target Volumes in Radiation Therapy Planning
- Gross tumor volume (GTV). Derived from the gross demonstrable extent and location of tumor identified with the CT or simulator images
- Clinical target volume (CTV). Expanded GTV to include subclinical disease based on additional clinical information
- Planning target volume (PTV). Expanded CTV by the addition of a variable margin to take into account internal organ movement as well as patient motion and setup uncertainties10,11
- Biological target volumes (BTV). Derived from the biological information about tumors and surrounding normal tissue obtained from the PET component of PET/CT.12 BTV can have different implications based upon the PET radiotracer used for scanning; for example, BTV determined from FDG might be considered to represent the viable tumor cells and can be used to determine a minimum dose for the entire tumor. However, a BTV resulting from fluoromisonidazol (FMISO; a PET tracer for hypoxia) would represent a smaller tumor subvolume, where the maximum tumor dose requires delivery to the hypoxic cells associated with radioresistance.
Clinical Applications
Integration of PET images in radiotherapy planning has been primarily studied in non–small cell lung cancer (NSCLC),13–15 with some studies published on esophageal carcinoma,16 head and neck cancer (HNC),17 and rectal carcinoma.18
Study Results for Lung Cancer
- A survey of the published findings shows that treatment volumes are significantly altered in 30 to 60% patients with NSCLC with the addition of biologic targeting with FDG PET.19
- In a series of 44 patients of NSCLC, FDG PET altered the stage of the disease in 25% of the cases by downstaging the disease in a majority of them.20 GTV based on FDG PET was on average smaller than the GTV defined by CT. Also, in a different study it was found that for the same radiotoxicity to the lungs, spinal cord, and esophagus, the dose to the tumor can be enhanced by 25%; this resulted in a potentially higher tumor control probability (24% for PET/CT planning compared with 6.3% for CT-only planning).21
- FDG PET also alters the delineation of the GTV by discriminating tumor tissue from atelectasis or necrosis.19,22,23 Modifications in target volume delineation allows delivering a tumoricidal dose to the target while minimizing the radiation dose to the uninvolved tissue.
Study Results for Head and Neck Carcinoma
Studies have shown excellent treatment outcomes in HNC patients treated with intensity-modulated radiation therapy (IMRT),24,25 in which many small pencil beams are used to conform the volume irradiated to any irregular shape. IMRT has the ability to deliver high doses of radiation to the tumor target with very high precision; thus, accurate tumor targeting and delineation are required more than ever.
Studies in patients with locally advanced head neck squamous cell carcinoma have demonstrated that PET/CT-based radiation treatment would significantly change the dose distribution.26,27
PET/CT has also been found helpful in the management of occult primary head and neck tumors by determining a site of origin of the primary tumor in 60%.28 This translates into reduced dose distribution to uninvolved mucosal sites compared with the results of CT scan only–based plans.
Dose Painting and Theragnostic Imaging
These novel concepts are primarily based upon the results of scanning with novel imaging markers of tumor hypoxia or proliferation and variations in tumor volume as well as viability during radiotherapy that could modify the delineation of target volumes.
Dose Painting
Information obtained from other novel tracers like those of hypoxia, proliferation, apoptosis, and receptor expression can be integrated with that of FDG PET imaging, which provides greater insight into the biologic pathways involved in radiation responses.29–34 Taken together, these can be utilized by the new sophisticated software planning algorithms to deliver IMRT treatments,35–39 where the intensity through the treatment beam is varied. Combining several IMRT treatment portals can result in complex cross-sectional dose distributions being achieved and even the delivery of high-dose areas within the target, a technique that has been referred to as “dose painting.”12 This concept depends on the ability to visualize tumor subvolumes, which are potentially radioresistant, and then paint some additional dose restricted to those subvolumes.
PET/CT for Adaptive Radiotherapy during Course of Treatment: Theragnostic Imaging
This is based upon calculation of the mean dose delivery during the early part of the treatment and the tumor response during this initial period of therapy and revision of the subsequent treatment plan.40–43 This replanning of the radiotherapy during the course of treatment can help in substantial sparing of the surrounding nontarget tissues and is the major advantage of “theragnostic” imaging, a term coined by Bentzen.43
Respiratory Gating of PET
- The aim is to accurately target the dose of radiation to the “diseased” tissue volume with relative sparing of the surrounding normal tissue.
- Gating systems that can be coupled with the development of treatment plans and target volumes in equivalent physiologic state are under active research. These will allow the radiation to be delivered in synchrony with physiologic motion such as respiration.44–47
- These are especially relevant to the treatment of the thoracic neoplasms.
Future Developments for Incorporating PET into Routine Radiotherapy Planning
- Interpretation of PET images for contouring target volumes
- Proper coregistration of PET and CT images
- Computer software for easy image transfer to and acceptance by treatment planning systems from the PET systems
- Mechanisms to account for tumor motion
Promising Approaches
- Use of four-dimensional PET/CT can correct for respiratory motion artifacts seen in conventional PET/CT imaging. It has the potential to reduce smearing and improve the accuracy in PET/CT coregistration.
- Employing PET/CT for radiosurgery treatment planning for the purpose of improved local control rates following radiosurgery and to reduce subsequent tumor recurrence rates.
Summary
The literature with regard to the clinical impact and patient outcome of using PET/CT to modify dose in metabolically active tumor cells is evolving at this time, and further clinical studies are needed. The detailed structural and functional imaging information potentially improves RTP by minimizing unnecessary irradiation of normal tissues and by reducing the risk of geographic miss. The potential of PET to quantify metabolism and identify new imaging targets within tumor tissue such as cellular proliferation, hypoxia, tumor receptors, and gene expression, thereby helping in the biological optimization of dose delivery, is an exciting area of research.45–49 However, the impact of PET/CT-based IMRT dose painting RTP on tumor control and clinical outcome can only be determined with prospective research studies of patients.
References
- Mah K, Caldwell CB, Ung YC, et al. The impact of (18)FDG–PET on target and critical organs in CT-based treatment planning of patients with poorly defined non-small-cell lung carcinoma: a prospective study. Int J Radiat Oncol Biol Phys 2002;52:339–350
- Ciernik IF, Dizendorf E, Baumert BG, et al. Radiation treatment planning with an integrated positron emission and computer tomography (PET/CT): a feasibility study. Int J Radiat Oncol Biol Phys 2003;57:853–863
- Dizendorf EV, Baumert BG, von Schulthess GK, et al. Impact of whole-body 18F-FDG PET on staging and managing patients for radiation therapy. J Nucl Med 2003;44:24–29
- Kalff V, Hicks RJ, MacManus MP, et al. Clinical impact of (18)F fluorodeoxyglucose positron emission tomography in patients with non-small-cell lung cancer: a prospective study. J Clin Oncol 2001;19:111–118
- van Baardwijk A, Baumert BG, Bosmans G, et al. The current status of FDG-PET in tumour volume definition in radiotherapy treatment planning. Cancer Treat Rev 2006;32(4):245–260
- Black QC, Grills IS, Kestin LL, et al. Defining a radiotherapy target with positron emission tomography. Int J Radiat Oncol Biol Phys 2004;60:1272–1282
- Daisne J-F, Sibomana M, Bol A, Doumont T, Lonneux M, Gregoire V. Tri-dimensional automatic segmentation of PET volumes based on measured source-to-background ratios: influence of reconstruction algorithms. Radiother Oncol 2003;69:247–250
- Erdi YE, Mawlawi O, Larson SM, et al. Segmentation of lung lesion volume by adaptive positron emission tomography image thresholding. Cancer 1997; 80(12, Suppl)2505–2509
- Yaremko B, Riauka T, Robinson D, Murray B, Mc Ewan A, Roa W. Threshold modification for tumour imaging in non-small cell lung cancer using positron emission tomography. Nucl Med Commun 2005;26:433–440
- International Commission on Radiation Units and Measurements. Prescribing, recording and reporting photon beam therapy, ICRU Report 50. Bethesda, MD: International Commission on Radiation Units and Measurements; 1993
- International Commission on Radiation Units and Measurements. Prescribing, recording and reporting photon beam therapy (Supplement to ICRU Report 50), ICRU Report 62. Bethesda, MD: International Commission on Radiation Units and Measurements; 1999
- Ling CC, Humm J, Larson S, et al. Towards multidimensional radiotherapy (MD-CRT): biological imaging and biological conformality. Int J Radiat Oncol Biol Phys 2000;47:551–560
- Caldwell CB, Mah K, Ung YC, et al. Observer variation in contouring gross tumour volume in patients with poorly defined non-small-cell lung tumours on CT: the impact of 18FDG-hybrid PET fusion. Int J Radiat Oncol Biol Phys 2001;51:923–931
- Erdi YE, Rosenzweig K, Erdi AK, et al. Radiotherapy treatment planning for patients with non-small cell lung cancer using positron emission tomography (PET). Radiother Oncol 2002;62:51–60
- Fox JL, Ramesh R, O’Meara W, et al. Does registration of PET and planning CT images decrease interobserver and intraobserver variation in delineating tumour volumes for non-small cell lung cancer? Int J Radiat Oncol Biol Phys 2005;62:70–75
- Moureau-Zabotto L, Touboul E, Lerouge D, et al. Impact of CT and 18F-deoxyglucose positron emission tomography image fusion for conformal radiotherapy in esophageal carcinoma. Int J Radiat Oncol Biol Phys 2005;63:340–345
- Scarfone C, Lavely WC, Cmelak AJ, et al. Prospective feasibility trial of radiotherapy target definition for head and neck cancer using 3 dimensional PET and CT imaging. J Nucl Med 2004;45:543–552
- Roels S, Duthoy W, Haustermans K, et al. Definition and delineation of the clinical target volume for rectal cancer. Int J Radiat Oncol Biol Phys 2006;65:1129–1142
- Bradley JD, Perez CA, Dehdashti F, et al. Implementing biologic target volumes in radiation treatment planning for non-small cell lung cancer. J NucI Med 2004;45(suppl ll):S96–S101
- De Ruysscher D, Wanders S, van Haren E, et al. Selective mediastinal node irradiation based on FDG-PET scan data in patients with non-small-cell lung cancer: a prospective clinical study. Int J Radiat Oncol Biol Phys 2005;62:988–994
- De Ruysscher D, Wanders S, Minken A, et al. Effects of radiotherapy planning with a dedicated combined PET-CT-simulator of patients with non-small cell lung cancer on dose limiting normal tissues and radiation dose-escalation: a planning study. Radiother Oncol 2005;77:5–10
- Nestle U, Walter K, Schmidt S, et al. 18F-deoxyglucose positron emission tomography (FDG-PET) for the planning of radiotherapy in lung cancer: high impact in patients with atelectasis. Int J Radiat Oncol Biol Phys 1999;44:593–597
- Bradley J, Thorstad WL, Mutic S, et al. Impact of FDG-PET on radiation therapy volume delineation in non-small-cell lung cancer. Int J Radiat Oncol Biol Phys 2004;59:78–86
- Chao KS, Deasy JO, Markman J, et al. A prospective study of salivary function sparing in patients with head-and-neck cancers receiving intensity-modulated or three-dimensional radiation therapy: Initial results. Int J Radiat Oncol Biol Phys 2001;49:907–916
- Lee N, Xia P, Quivey JM, et al. Intensity-modulated radiotherapy in the treatment of nasopharyngeal carcinoma: an update of the UCSF experience. Int J Radiat Oncol Biol Phys 2002;53:12–22
- Schwartz DL, Ford E, Rajendran J, et al. FDG-PET/CT imaging for preradiotherapy staging of head-and-neck squamous cell carcinoma. Int J Radiat Oncol Biol Phys 2005;61:129–136 [Medline]
- Schwartz DL, Ford EC, Rajendran J, et al. FDG-PET/CT-guided intensity modulated head and neck radiotherapy: a pilot investigation. Head Neck 2005;27:478–487
- Wong WL, Saunders M. The impact of FDG PET on the management of occult primary head and neck tumors. Clin Oncol (R Coll Radiol) 2003;15:461–466
- Fujibayashi Y, Taniuchi H, Yonekura Y, Ohtani H, Konishi J, Yokoyama A. Copper-62-ATSM: a new hypoxia imaging agent with high membrane permeability and low redox potential. J Nucl Med 1997;38:1155–1160
- Shields AF, Grierson JR, Dohmen BM, et al. Imaging proliferation in vivo with [F-18]FLT and positron emission tomography. Nat Med 1998;4:1334–1336
- Borbath I, Gregoire V, Bergstrom M, Laryea D, Langstrom B, Pauwels S. Use of 5-[(76)Br]bromo-2′-fluoro-2′-deoxyuridine as a ligand for tumour proliferation: validation in an animal tumour model. Eur J Nucl Med Mol Imaging 2002;29:19–27
- Gronroos T, Bentzen L, Marjamaki P, et al. Comparison of the biodistribution of two hypoxia markers [18F]FETNIM and [18F]FMISO in an experimental mammary carcinoma. Eur J Nucl Med Mol Imaging 2004;31:513–520
- Mahy P, De Bast M, Leveque PH, et al. Preclinical validation of the hypoxia tracer 2-(2-nitroimidazol-1-yl)-N-(3,3,3-[(18)F]trifluoropropyl)acetamide, [(18)F]EF3. Eur J Nucl Med Mol Imaging 2004;31:1263–1272
- Mishani E, Abourbeh G, Jacobson O, et al. High-affinity epidermal growth factor receptor (EGFR) irreversible inhibitors with diminished chemical reactivities as positron emission tomography (PET)-imaging agent candidates of EGFR overexpressing tumors. J Med Chem 2005;48:5337–5348
- Chao KS, Bosch WR, Mutic S, et al. A novel approach to overcome hypoxic tumor resistance: Cu-ATSM-guided intensity-modulated radiation therapy. Int J Radiat Oncol Biol Phys 2001;49:1171–1182
- Douglas JG, Stelzer KJ, Mankoff DA, et al. [F-18]-fluorodeoxyglucose positron emission tomography for targeting radiation dose escalation for patients with glioblastoma multiforme: clinical outcomes and patterns of failure. Int J Radiat Oncol Biol Phys 2006;64:886–891
- Mutic S, Malyapa RS, Grigsby PW, et al. PET-guided IMRT for cervical carcinoma with positive para-aortic lymph nodes: a dose-escalation treatment planning study. Int J Radiat Oncol Biol Phys 2003;55:28–35
- Vanuytsel LJ, Vansteenkiste JF, Stroobants SG, et al. The impact of (18)F-fluoro-2-deoxy-D-glucose positron emission tomography (FDG-PET) lymph node staging on the radiation treatment volumes in patients with non-small cell lung cancer. Radiother Oncol 2000;55:317–324
- Lee NY, Mechalakos JG, Nehmeh S, et al. Fluorine-18-labeled fluoromisonidazole positron emission and computed tomography-guided intensity-modulated radiotherapy for head and neck cancer: a feasibility study. Int J Radiat Oncol Biol Phys 2008;70:2–13
- Geets X, Lee L, Lonneux M, Coche E, Cosnard G, Grégoire V. Re-assessment of HNSCC tumor volume during radiotherapy with anatomic and functional imaging. [abstract] Radiother Oncol 2006;78(Suppl 1):S59
- Humm JL, Lee J, O’Donoghue JA, et al. Changes in FDG tumor uptake during and after fractionated radiation therapy in a rodent tumor xenograft. Clin Positron Imaging 1999;2:289–296
- Brahme A. Biologically optimized 3-dimensional in vivo predictive assay-based radiation tberapy using positron emission tomography-computerized tomography imaging. Acta Oncol 2003;42:123–136
- Bentzen SM. Theragnostic imaging for radiation oncology: dose-painting by numbers. Lancet Oncol 2005;6:112–117
- Livieratos L, Stegger L, Bloomfield PM, et al. Rigid body transformation of list mode projection data for respiratory motion correction in cardiac PET. IEEE Medical Imaging Conference records. Piscataway, NJ: IEEE; 2003
- Wang Y, Baghaei H, Li H, et al. A simple respiration gating technique and its application in high resolution PET. IEEE Medical Imaging Conference records. Piscataway, NJ: IEEE; 2003
- Guivarc’h O, Turzo A, Visvikis D, et al. Synchronisation of pulmonary scintigraphy by respiratory flow and by impedance plethysmography. Proc SPIE Medical Imaging #5370. Bellingham, WA: SPIE;2004:1166–1175
- Erdi YE, Nehmeh SA, Pan T, et al. The CT motion quantitation of lung lesions and its impact on PET measured SUV’s. J Nucl Med 2004;45:1287–1292
- Grégoire V, Haustermans K, Geets X, Roels S, Lonneux M. PET-based treatment planning in radiotherapy: a new standard? J Nucl Med 2007;48(Suppl 1): 68S–77S
- Senan S, De Ruysscher D. Critical review of PET-CT for radiotherapy planning in lung cancer. Crit Rev Oncol Hematol 2005;56(3):345–351
- Ciernik IF, Dizendorf E, Baumert BG, et al. Radiation treatment planning with an integrated positron emission and computer tomography (PET/CT): a feasibility study. Int J Radiat Oncol Biol Phys 2003;57:853–863
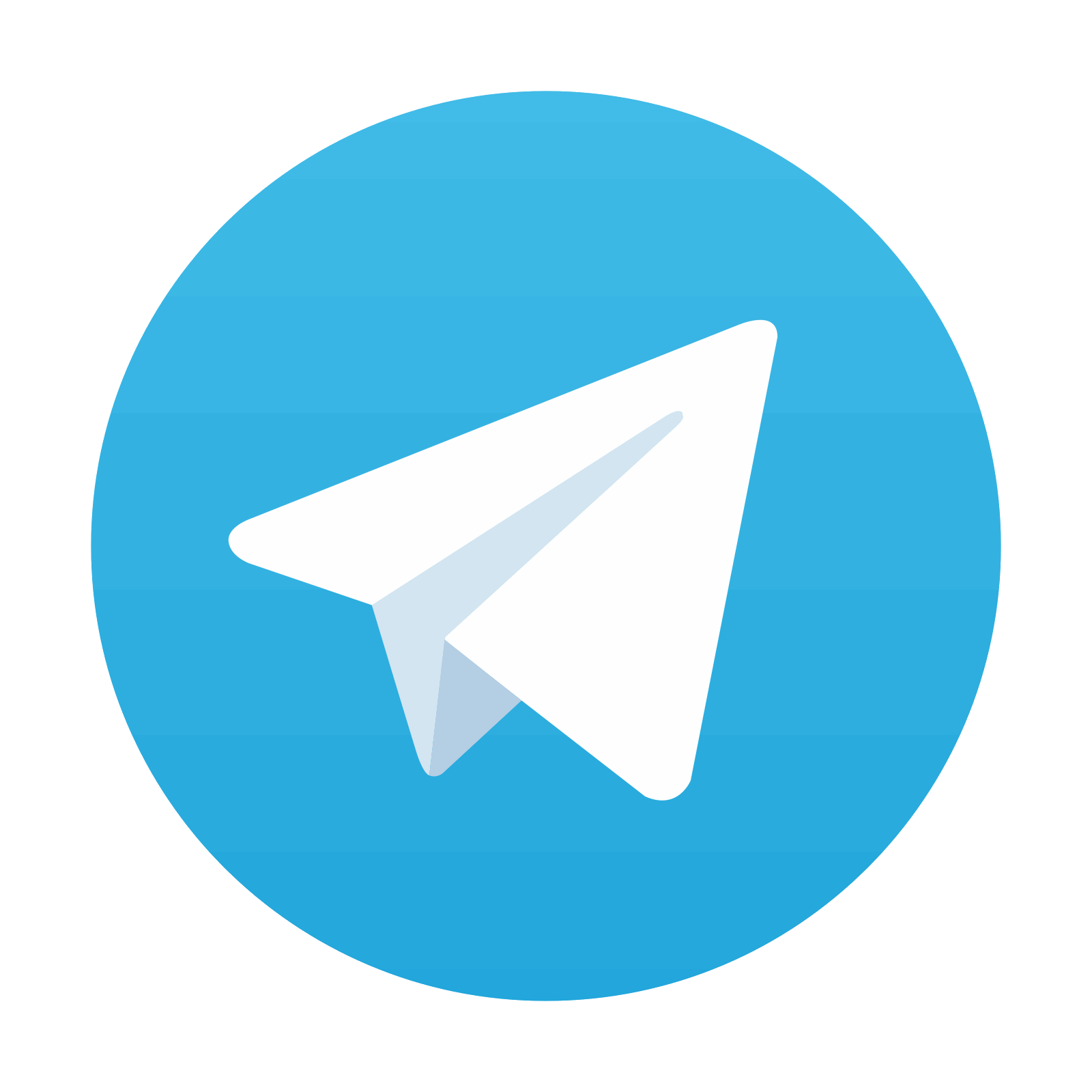
Stay updated, free articles. Join our Telegram channel

Full access? Get Clinical Tree
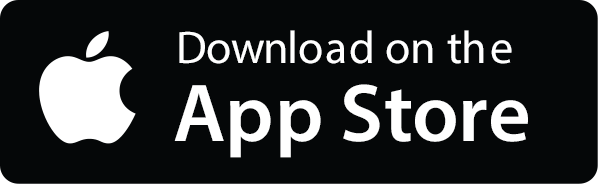
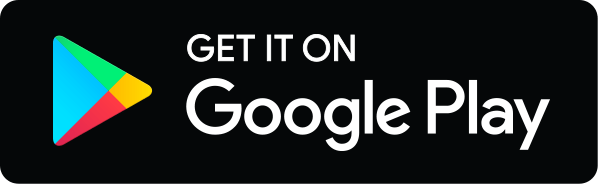