Fig. 53.1
Thoracoscopic view of the pleural cavity, looking toward the apex of the lung, showing the lung covered by visceral pleura (at bottom of the image) and the parietal pleura covering the inner surface of the ribs and chest wall
The pleural cavity refers to the space enclosed by the pleural membranes which in healthy states is approximately 10–20 μm across and contains 8–10 mL of fluid. The area of the entire pleura is estimated to be 2,000 cm2 in an average adult male. In humans, the left and right pleural cavities are separated from each other and from the pericardial space. The visceral pleura covers the lung surface and extends deep within the interlobar fissures. The parietal pleura can be divided into the diaphragmatic, mediastinal, cervical, and costal pleura, Fig. 53.2. The parietal pleura may extend inferiorly beyond the costal surface, specifically at the right lower sternal region and at the posterior junction of ribs and vertebra bilaterally.
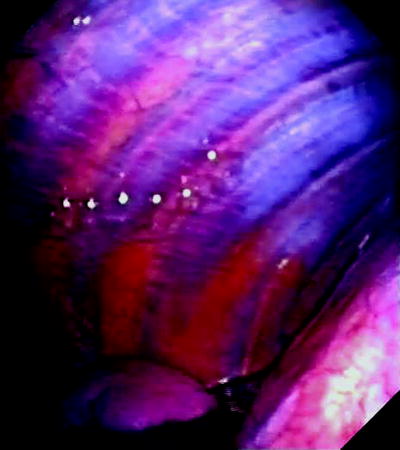
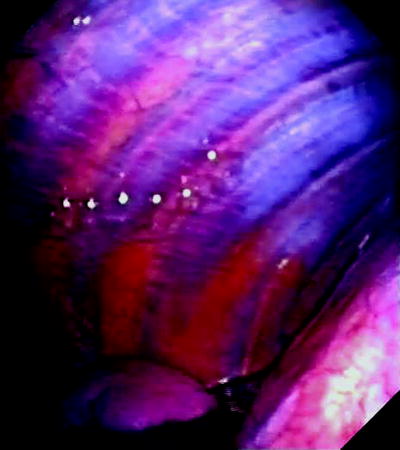
Fig. 53.2
Thoracoscopic view of normal costal parietal pleura. Normal pleura is extremely thin and offers a clear view of the underlying structures. Notice the ribs, and the intercostal vessels in between the ribs, running in parallel to each other. The diaphragm covered by the diaphragmatic pleura can be seen at the inferior right corner of the picture. A small amount of pleural fluid can be seen at the bottom of the pleural cavity
Blood Supply: Major, and at times fatal, bleeding is a known complication of pleural procedures – hence, understanding the blood supply of the pleura is important. The costal portion of the parietal pleura is supplied by the intercostal and internal mammary arteries. Contrary to conventional teaching that the intercostal arteries run inferiorly to the corresponding ribs, angiographic evidence shows that in the paravertebral regions these arteries often follow a variable course in the intercostal space not necessarily protected by the ribs. The intercostal arteries only consistently run parallel with the inferior margins of the ribs when the vessels reach the flanks. Elderly subjects often have more tortuous vessels and narrower rib spaces and are at risks of intercostal artery lacerations during pleural procedures. Percutaneous procedures, and thoracoscopic biopsies, should be performed as far away from paravertebral regions as possible.
The bronchial, upper diaphragmatic, internal mammary, and mediastinal arteries supply the mediastinal pleura; the subclavian artery supplies the cervical pleura, and the diaphragmatic pleura is supplied by the internal mammary artery and aorta, via posterior mediastinal and inferior phrenic arteries. Venous drainage follows arterial supply into the azygos vein and into the superior vena cava. The diaphragmatic pleura drains via the inferior phrenic veins into the inferior vena cava.
Arterial supply of the visceral pleura in humans is believed to arise from the bronchial arteries, although supply of the lung apex and its convex surface is debated. Venous drainage of the visceral pleura is mostly via the pulmonary veins.
Pleural Lymphatics: The lymphatics play a key role in fluid drainage of the pleural cavity. Fluid exits the pleural cavity by bulk flow (liquid and protein are evacuated at the same rate) via stomata (diameter 2.5–10 μm) on the parietal pleura, which empty into lymphatic plexuses in the intercostal spaces and over the diaphragm. The costal pleura drains into the internal mammary nodes anteriorly and the intercostal lymph nodes posteriorly. Pleura from the lung apex drains into the cervical chain, while pleura lining the diaphragm drains into the mediastinal nodes. Disease, especially malignant, involvement of thoracic lymph nodes often impairs the drainage routes and contributes to accumulation of pleural effusions.
A superficial network of lymphatic capillaries and collecting vessels exists on the visceral pleura, and flow from lymphatic capillaries is directed toward the hila of the lung via bronchovascular bundles. Disruption of the lung and pleural lymphatics during lung transplantation is believed to be a contributing cause of the early posttransplant pleural effusions which occur in practically all lung transplant patients.
Innervation: The parietal, but not the visceral, pleura is innervated by pain fibers. Hence, presence of pleuritic pain indicates pathologic, usually inflammatory or tumor, involvement of the parietal pleura which is supplied by the intercostal nerves. The central diaphragm is supplied by the phrenic nerve; irritation of the diaphragmatic pleura can induce referred pain to the ipsilateral shoulder. The visceral pleura is innervated by the vagus and sympathetic trunk.
Microscopic Anatomy
Both visceral and parietal pleurae in humans are approximately 40 μm thick. Between the pleural surface and underlying tissue, five layers are identified histologically, consisting of a single cellular layer and four subcellular layers (Fig. 53.3), as follows:
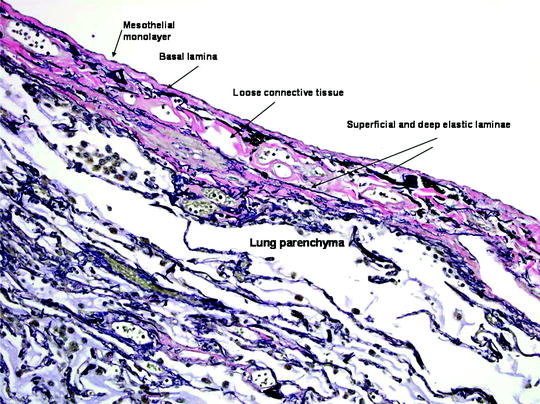
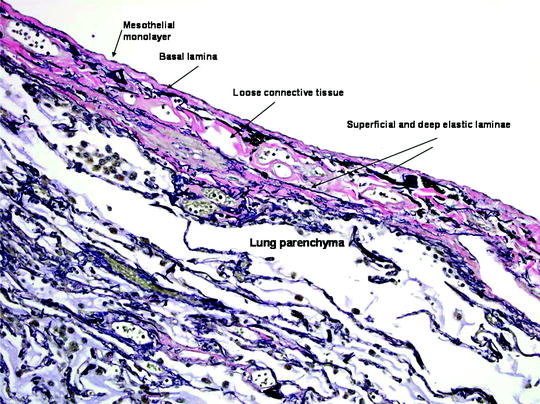
Fig. 53.3
The visceral pleura. The five layers of the visceral pleura, merging with underlying lung parenchyma. The elastic laminae of the pleura are highlighted in this Verhoeff-Van Gieson (VVG) stain for elastic fibers (VVG x 200) (Courtesy of Dr. A Segal, Perth, Australia)
1.
A monolayer of mesothelial cells
2.
The basal lamina and a thin connective tissue layer
3.
A thin superficial elastic layer, often merged with the second layer
4.
A loose connective tissue layer, containing nerves, blood vessels, and lymphatics
5.
A deep fibroelastic layer, often fused to the underlying tissue
Mesothelial Cells: Mesothelial cell is the predominant cell type in the pleural cavity. They vary from flat to cuboidal and can range from 10 to 50 μm in diameter and from 1 to >4 μm in thickness. Mesothelial cells are adherent to one another at the apical surface via tight junctions. At the basal surface, the cells are more loosely associated, although the basal portions are often seen to overlap. The cells slide over one another during the respiratory cycle, and therefore, at full inspiration the overlap disappears completely.
The pleural cavity is frequently invaded by undesirable agents, but the pleural cavity is not under close surveillance by polymorphonuclear cells. Mesothelial cells thus provide the frontline defense against invading cells (e.g., cancer), pathogens (e.g., bacteria), and particular matters (e.g., asbestos) by provoking a significant inflammatory response, phagocytosis, and release of potent cytokines which effectively recruit inflammatory cells (e.g., neutrophils) to initiate appropriate immune responses to eradicate the invading molecules. Mesothelial cells are multipotent and have definite roles in extracellular matrix synthesis and hence pleural fibrosis and repair. The diverse range of biological functions mesothelial cells play in both health and disease states is reviewed elsewhere.
Pathologic Changes in the Pleura
A diverse range of insults can affect the pleura, and the resultant responses are equally complex. Most pleural disorders, however, involve inflammatory changes, fibrosis, and often vascular hyperpermeability, leading to fluid accumulation. Detailed discussion on pleural pathologies can be found in specialist texts.
Pleural Inflammation and Fibrosis: Acute pleuritis develops with many pleural diseases (e.g., infection) as well as iatrogenic procedures (e.g., pleurodesis), and if persists, the chronic inflammation often progresses to pleural fibrosis and thickening (e.g., asbestos-related fibrothorax) (Fig. 53.4). In addition to fibroblasts, mesothelial cells also contribute to collagen and matrix synthesis in pleural fibrosis. Mesothelial cells can undergo epithelial-mesenchymal transformation and convert into fibroblast-like cells, a process implicated in peritoneal fibrosis. For detailed review of the causes and pathology of pleural fibrosis, please refer to reviews elsewhere.
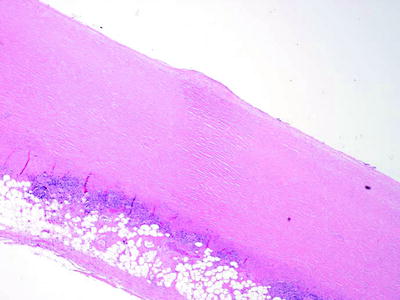
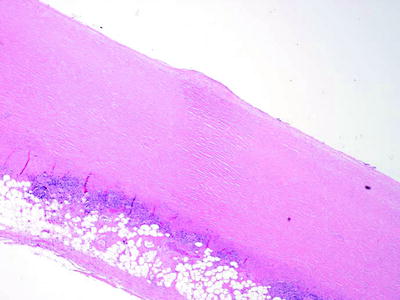
Fig. 53.4
Fibrous pleural plaque of the parietal pleura, demonstrating “basket-weave” collagen (H & E x40) (Courtesy of Dr. A Segal, Perth, Australia)
Pleural Effusion: The development of pleural effusions is further discussed below.
Pleural Malignancy: An estimated 300,000 patients develop a malignant pleural effusion per annum in the USA, which can arise from metastatic or primary pleural cancers. Metastatic pleural disease accounts for the majority of cases (Fig. 53.5a) with lung and breast being the most common primary cancer sites. In Europe, one million patients with lung cancer develop a pleural effusion each year. Cancer cells embolize to peripheral lung tissues and/or directly invade the visceral pleura before spreading onto the parietal surface. Occasionally, hematogenous or direct spread to the parietal pleura can occur. In contrast, primary pleural mesothelioma (Fig. 53.5b) is believed to originate from the parietal pleura before spreading to the visceral pleura. Mesothelioma patients with disease limited to the parietal, but not the visceral, pleura have been shown to have better prognosis (33 vs. 7 months). Figure 53.6 shows an example of the histological appearance of a malignant pleural mesothelioma.
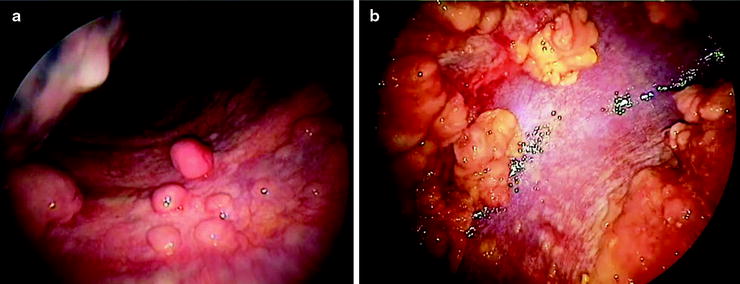
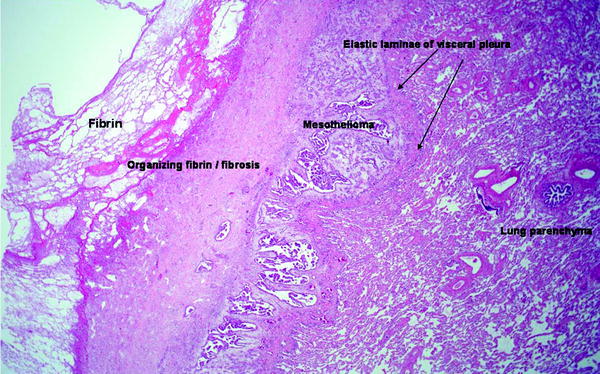
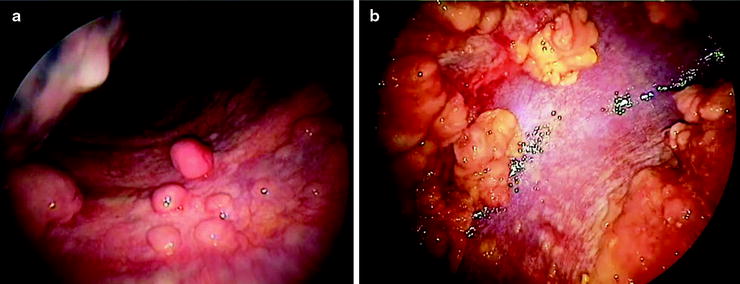
Fig. 53.5
Thoracoscopic views of (a) multiple metastatic carcinoma deposits on the parietal pleura and (b) malignant mesothelioma at the parietal pleura (left) and diaphragm (right) sparing the costophrenic angle (Courtesy Dr. N Rahman, Oxford, UK)
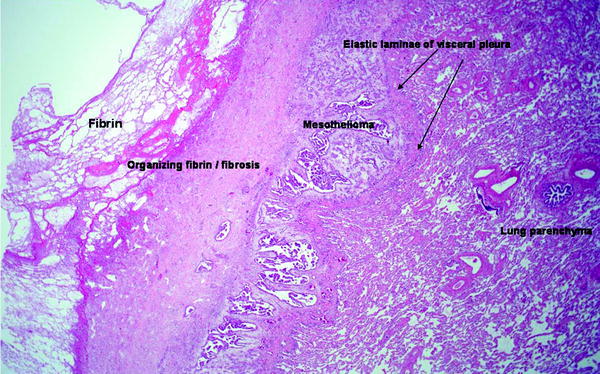
Fig. 53.6
Malignant mesothelioma, epithelioid type. A reactive zone of fibrin and fibrous tissue (left) overlies the tumor, which is separated from the underlying lung parenchyma by the elastic lamina of the visceral pleura (H & E x20) (Courtesy of Dr. A Segal, Perth, Australia)
The importance of pleural involvement in non-small cell lung cancer has been recognized in the latest (7th edition) revised “TNM classification of malignant tumors”; the presence of a malignant pleural effusion staged the cancer at M1a (stage IV), reflecting the advanced (and inoperable) nature of the disease when the pleura is involved. A series of studies have shown that positive pleural lavage cytology for malignant cells is a poor prognostic indicator in patients undergoing resection of lung cancer, with a median survival of 13 months compared to 49 months if negative. The importance of pleural lavage cytology has recently been emphasized in a meta-analysis. Currently, results of pleural lavage are not part of the TNM staging system. However, a new classification of visceral pleural invasion (VPI), PL0-3, has been proposed and is now in use in many centers:
PL0: tumor within the subpleural parenchyma or invading superficially into the pleural connective tissue below the elastic layer; PL1: tumor invades beyond the elastic layer;
PL2: tumor invades the visceral pleural surface; PL3: tumor invades the parietal pleura.
Breaching the elastic layer of the visceral pleural layer, PL1 and 2, confers VPI and a T status of pT2. Five of the six studies using this staging system showed adverse prognosis for patients with VPI. Parietal involvement is termed pT3.
Pleural malignancies often express high levels of vascular endothelial growth factor (VEGF), which in animal studies is a key driving force for pleural/peritoneal fluid formation. The role of antagonizing VEGF to control malignant effusions has not been established.
Pneumothorax: Stretched out visceral pleura in the statically expanded lung apices is prone to bleb and bullae formation, even in nonsmoking individuals. These are thought to be an important factor in predisposition to primary spontaneous pneumothorax. A recent study of primary pneumothorax using fluorescein-enhanced autofluorescence thoracoscopy raises the possibility of diffuse leak from the visceral surface (“pleural porosity”) rather than one ruptured bleb as the source of air leak from the lung.
Due to the anatomical boundaries of the parietal pleura, iatrogenic pneumothorax may result from insertion of subclavian central venous catheters, damaging the pleura above the first rib. Due to pleural extension below the costal margin inferiorly, pneumothorax may occur during attempted posterior access to upper abdominal organs.
Role of the Pleural Cavity
The pleura permits friction-free movement of the lungs within the relatively rigid thorax and facilitates the development of positive and negative intrapleural pressure during the respiratory cycle. A patent pleural cavity is, however, not essential to life. Longitudinal studies of patients who underwent talc pleurodesis to obliterate the pleural space as treatment for pneumothoraces showed minimal restrictive changes in lung functions after 22–35 years. This was collaborated by animal studies which showed no major impairment in lung volumes and gaseous exchange following pleurodesis. Studies of elephants showed that many were autopleurodesed at birth, and their pleural cavity is replaced by fibrous tissues. It is intriguing why humans, throughout evolution, maintain a patent pleural cavity that is nonessential and susceptible to numerous disease pathologies.
Pleural Fluid Formation and Absorption
In the healthy state, the pleural cavity contains a small amount of normal physiologic fluid to facilitate the gliding of the visceral pleura over the parietal pleural membrane. This pleural fluid is formed by filtration, according to the net hydrostatic-oncotic pressure gradient, from the systemic, especially the intercostal arterial, circulation of parietal pleura. Water and small molecules (≤4 nm) can pass freely between the mesothelial cells, whereas transcytosis can allow active transport of larger particles through the mesothelial cells.
There is approximately 0.13 ± 0.06 mL/kg body mass of normal physiologic fluid in each hemithorax as estimated by the urea dilution method in a pleural lavage study of normal subjects undergoing thoracoscopy. The fluid is a transudate with low protein and lactate dehydrogenase (LDH), and its biochemical composition (e.g., glucose and urea concentrations) resembles that of other interstitial fluids. Total leukocyte counts average 1,716 cells/μL, which are predominantly (75%) macrophages and lymphocytes (23%) in nonsmokers, but the numbers of neutrophils are significantly raised in smokers.
Extrapolating from animal data, a 70-kg man produces 17 mL/day of physiologic pleural fluid. The rate of formation approximates 0.01 (in sheep) to 0.02 (in rabbits) mL/kg/h, and the half-life of fluid turnover is 6–8 h. The drainage capacity in normal pleura is large (estimated around 0.2–0.3 mL/kg/h) and well over the usual production rates.
Pleural Fluid Formation in Pathologic States
A pleural effusion, an abnormal accumulation of pleural fluid, develops when the rate of pleural fluid formation exceeds the rate of its removal. Most effusions develop from both increases in pleural fluid entry and decreases in fluid exit rates. In the presence of the normal fluid absorption capacity, fluid formation has to increase by over 30-fold, and stay at that rate, to create an effusion. On the other hand, decreased removal of the fluid alone is unlikely to result in significant accumulation of pleural fluid, given the normal rate of pleural fluid formation is low.
Transudates account for ∼60% of pleural effusions seen in clinical series. They are formed when the Starling’s equation is disturbed by increased intravascular pressures (most commonly in congestive cardiac failure) and/or decreased pleural fluid oncotic pressures (e.g., in cirrhosis and nephrotic syndrome): all of which can contribute to fluid movement across the pleural capillaries to the pleural cavity.
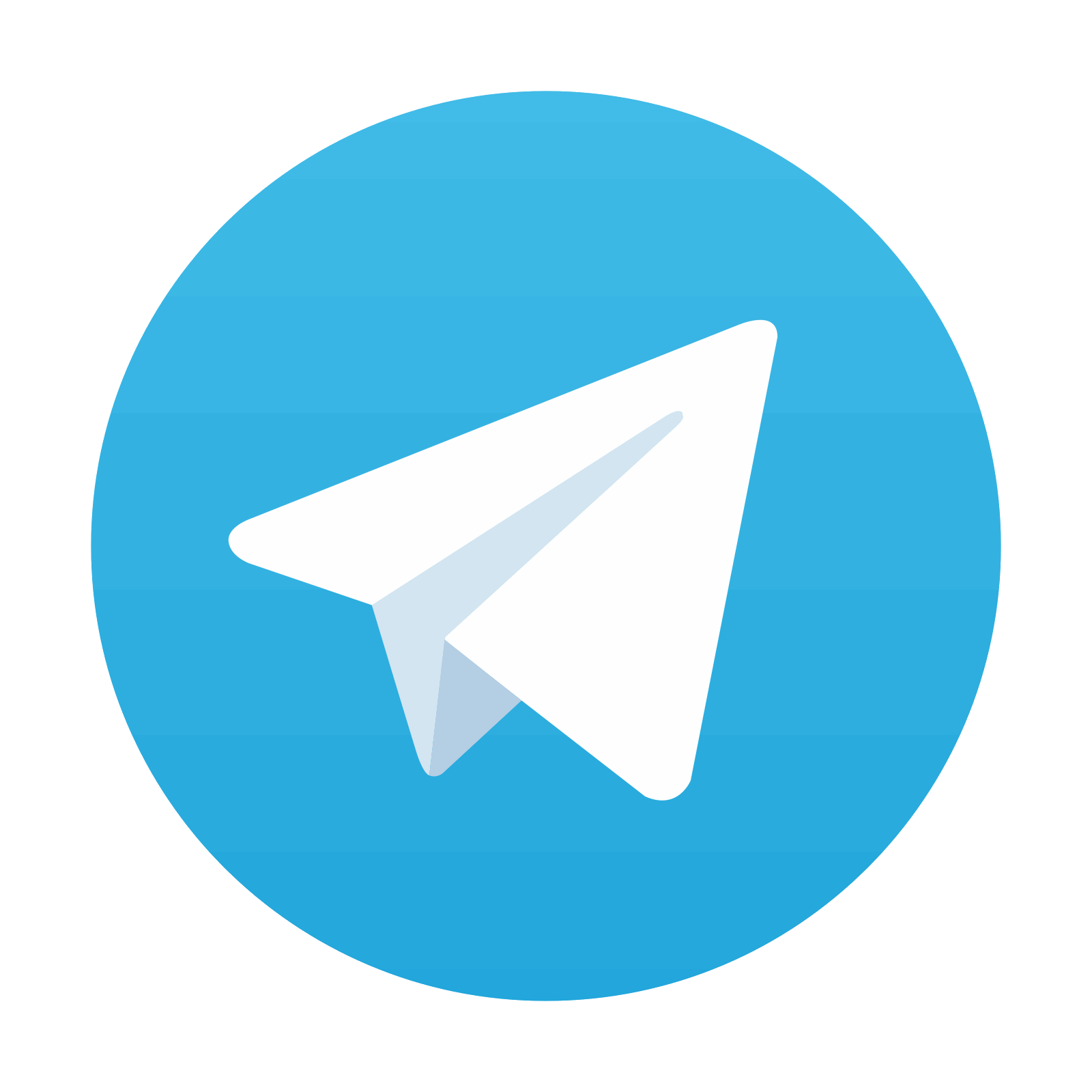
Stay updated, free articles. Join our Telegram channel

Full access? Get Clinical Tree
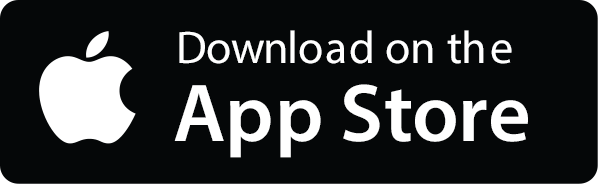
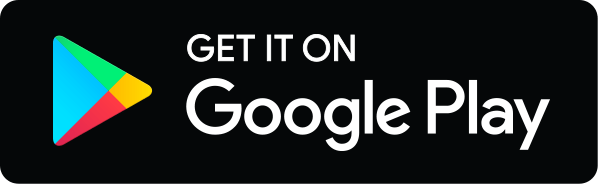