David Li • Richard H. Marshall • David C. Madoff
The hepatitis C epidemic in the United States has contributed to a dramatic rise of hepatocellular carcinoma (HCC), with HCC increasing in incidence at a rate of 4.5% per year from 1980 to 2005.1,2 HCC has a poor prognosis and is the third leading cause of cancer-related mortality despite being the sixth most common cancer worldwide.3 Given both its increased incidence and poor prognosis, the mortality rate from primary liver cancer has increased more than any other major cancer in the United States over the past several decades.1 In addition, the liver remains a common site of metastases from colorectal carcinoma and other primary tumors.4 Surgical resection of primary tumors and metastases confined to the liver remains the mainstay of curative therapy.5,6
In recent years, advances in hepatobiliary surgical techniques have led to improved morbidity and mortality in patients after hepatic resection.7,8 Despite these advances, major hepatic resection (i.e., greater than three Couinaud segments) places patients at risk for developing complications related to liver insufficiency in the perioperative period. The anticipated volume of liver which remains after surgery, termed the future liver remnant (FLR), has been shown to be a strong, independent predictor of postoperative complications.9,10
Portal vein embolization (PVE) is a well-established procedure to redirect portal blood flow to the intended FLR for patients who are candidates for extensive liver resection to promote hypertrophy of nonembolized segments that will remain after resection.11–13 When needed, this increased FLR volume is associated with improved biliary excretion, albumin uptake, and postoperative liver function in patients undergoing major hepatectomy.14–16 PVE has also been shown to improve the functional reserve of the FLR before surgery; reduce perioperative morbidity; and allow for safe, potentially curative hepatectomy in patients previously considered ineligible for resection based on anticipated small remnant liver volumes.17–20 This chapter summarizes the mechanism of action, current indications, outcomes, techniques, and complications related to PVE.
MECHANISM OF ACTION
The liver is unique for its enormous capacity for regeneration: even a loss of as much as two-thirds of the liver parenchyma can be compensated with complete recovery of liver function within 2 weeks.21 Regeneration of the liver depends on portal blood flow delivering multiple mitogenic factors, the most potent hepatotrophic agents being hepatocyte growth factor (HGF) and tumor growth factor alpha (TGF-α).22,23 Comitogenic factors, such as insulin and glucagon, are transported via the portal system and act in concert with hepatotrophic agents to stimulate cytokine release and ultimately hepatocyte proliferation. The synergistic action of insulin with HGF accounts for the observation that hepatic regeneration rates are slower in diabetic as compared to nondiabetic patients.24,25
Regeneration of the liver depends on both the stimulus of injury and the condition of the liver parenchyma. Hepatocyte proliferation is directly proportional to the degree of severity of the insult to the liver: minor injuries (i.e., <10% parenchymal involvement) induce only localized mitotic reactions, whereas major injuries (i.e., >50% parenchymal involvement) induce multiple mitotic waves throughout the entire liver.21 Liver regeneration rates depend on the time from injury, with the greatest rate of regeneration after PVE occurring within the first 2 weeks (Fig. 31.1).10 Hepatocyte removal or necrosis is a stronger stimulus for liver regeneration as compared to cell-mediated apoptosis.26,27 Apoptosis is the predominant mechanism of cell death in PVE, thus regeneration after PVE occurs at a slower rate compared with hepatectomy.10 In addition, PVE is known to cause minimal pain and fever as compared to the postembolization syndrome associated with transarterial embolization where necrosis is the primary mechanism of cell death.28 Cirrhotic livers are known to have both a reduced rate and capacity for liver regeneration.29 Both a suboptimal hepatocyte microenvironment with fibrosis reducing delivery of portal flow and a blunted response of the diseased hepatocytes to hepatotrophic factors are thought to contribute to the reduced regeneration ability of cirrhotics.30 One novel approach to augmentation of liver regeneration rates in the setting of PVE has been the use of stem cells. Esch et al.31 reported greater absolute and relative FLR volumes after adjuvant stem cell infusion in conjunction with PVE in a small cohort of patients.
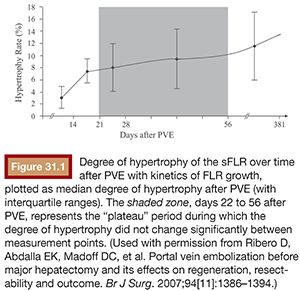
FUTURE LIVER REMNANT DETERMINATION
PVE is indicated when the anticipated FLR is insufficient to support hepatic function, particularly in the perioperative period, before the liver has had time to regenerate. Accurate calculation of the FLR is essential in triaging the potential hepatectomy candidates for whom PVE is indicated. Liver volume is directly correlated with a patient’s size; hence, normalizing the anticipated liver volume to a patient’s size results in a more accurate assessment of the FLR.32,33 This principle led to the proposal and clinical validation of a standardized FLR (sFLR) by Vauthey et al.,33 expressed as a ratio of the FLR over the total estimated functioning liver volume (TELV): sFLR = FLR/TELV.
Computed tomography (CT) volumetry serves as the standard for FLR measurement as it is accurate within ±5% of estimating normal liver parenchymal volumes (Fig. 31.2).33,34 Several methods have been used to measure TELV, including those based on CT volumetry, body surface area (BSA), or body weight. Vauthey et al. derived the following formula for estimating TELV by analyzing liver size and BSA in 292 Western adults: TELV = −794.41 + 1,267.28 × BSA, which has been demonstrated to be the least biased and most accurate in adult patients by meta-analysis as compared to similar formulas.35,36 Other formulas for determining TELV from CT volumetry are both tedious and imprecise because measurements of the tumor volume must be performed and excluded from the overall liver volume using this method. Ribero et al.37 verified that CT volumetry was less accurate than BSA for calculating sFLR by identifying a subset of patients for whom CT volumetry underestimated the risk of hepatic insufficiency. Chun et al.38 found the body weight method to be equally as predictive as BSA; however, a more recent study comparing direct volumetric liver measurement and estimated liver volume based on BSA found the TELV method to be superior (P < .005).39
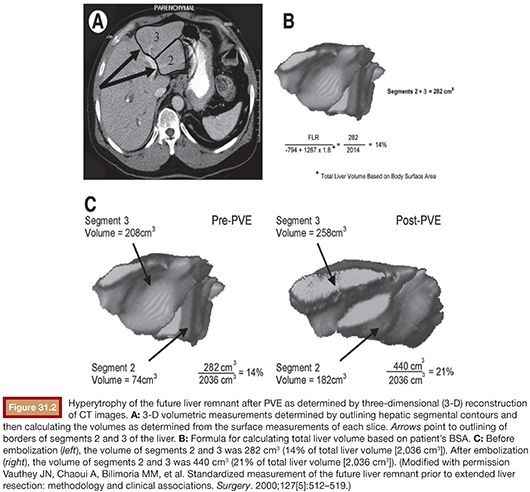
INDICATIONS
Outcomes in Healthy Livers
Multiple studies have demonstrated that hepatectomy in a setting of sFLR less than 20% is associated with increased postoperative complications.10,13,40 As a result, the National Comprehensive Cancer Network treatment guidelines from 2013 (category IIA) endorse an sFLR of greater than 20% as a minimum threshold for patients without underlying liver disease to safely undergo hepatectomy, with consideration for PVE to be performed on patients below that threshold.5 Ribero et al.10 found that both sFLR less than 20% and degree of sFLR hypertrophy after PVE less than 5% predicted outcome after resection in a series of 112 patients (Fig. 31.3). Kishi et al.40 published a series of 301 consecutive patients who underwent extended right hepatectomy and found that patients with a preoperative sFLR less than 20% had significantly higher rates of postoperative liver insufficiency and death from liver failure compared with patients with sFLR greater than 20% (P < .05). In addition, patients who underwent PVE before surgery to increase their sFLR from less than 20% to greater than 20% had statistically equivalent rates of liver insufficiency as patients with greater than 20% at baseline (Fig. 31.4). This study confirmed both the sFLR threshold of less than 20% being associated with increased perioperative complications and the beneficial role of PVE in reducing perioperative complication rates in those patients who hypertrophy their liver to an sFLR greater than 20%.
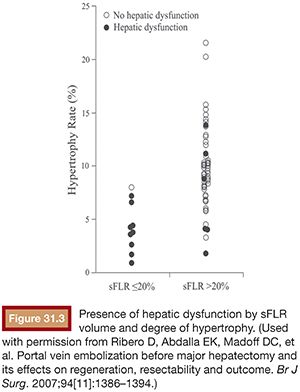
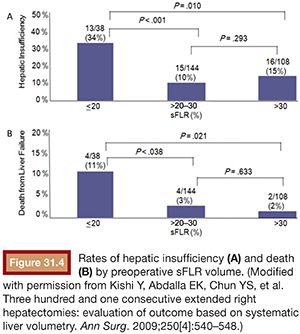
Recently, Shindoh et al.41 have proposed the kinetic growth rate (defined as degree of hypertrophy at initial volume assessment divided by number of weeks elapsed after PVE) as a predictor of postoperative complications after hepatectomy as compared to the sFLR. They analyzed a series of 107 patients who underwent right PVE and subsequent right hemihepatectomy or extended right hepatectomy and found the kinetic growth rate to be the most accurate predictor of postoperative hepatic insufficiency and mortality when compared to sFLR or degree of hypertrophy measurements using receiver operating characteristic analysis (Fig. 31.5). Of the three measures, a kinetic growth rate cutoff value of less than 2.0% per week demonstrated the highest accuracy (81%), with sensitivity of 100% and specificity of 71% in predicting postoperative hepatic insufficiency.
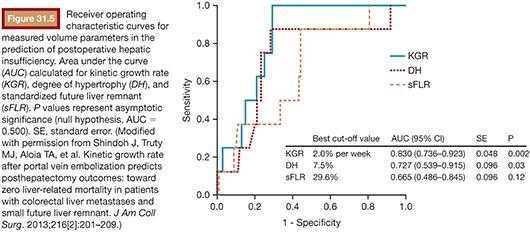
Outcomes in Diseased Livers
Liver regeneration occurs at a reduced rate and capacity in diseased livers.21,29,30,42 This observation has directly correlated to clinical outcomes. De Meijer et al.43 performed a meta-analysis of four studies involving 1,000 patients and found that patients with greater than 30% steatosis of the liver had significantly higher risk of postoperative complications and postoperative death compared with patients without steatosis (relative risk and 95% confidence interval 2.01 and 1.66 to 2.44 vs. 2.79 and 1.19 to 6.51). Similarly, several series evaluating outcomes after extensive hepatic resection demonstrated higher rates of both postoperative hepatic insufficiency and mortality in cirrhotic patients.44,45 Hence, higher sFLR cutoffs are considered for patients with additional risk factors such as hepatic steatosis, hepatotoxic chemotherapy exposure, and compensated cirrhosis.
Cirrhosis
For patients with well-compensated cirrhosis (i.e., Child- Pugh class A) who are considered for resection, an sFLR greater than 40% is recommended. In support of this recommendation, Shirabe et al.46 demonstrated that all cases (n = 7) of postoperative hepatic insufficiency occurred when the FLR was calculated to be less than 250 mL/m2 (which corresponds to a calculated sFLR of <40%) in a series of 80 cirrhotic patients who underwent major hepatic resection. A prospective alternative allocation trial in which 28 patients with chronic liver disease were allocated to PVE or no PVE before resection further validated the higher minimum sFLR cutoff of less than 40%; the PVE group had a mean sFLR size of 35% and demonstrated significantly lower incidence of complications including liver failure.47
Chemotherapy
Accelerated tumor growth after PVE has been reported for both primary and metastatic liver tumors.48–51 Progression of disease after PVE may preclude curative intent surgery; a 20% dropout rate after first-stage resection due to progression of disease has already been reported in two-stage hepatectomy series.52,53 Neoadjuvant chemotherapy can be administered in an attempt to provide tumor control in the interim between PVE and resection; however, concerns have been raised about its potential deleterious effect on liver function, liver hypertrophy, and lack of efficacy in preventing progression of disease.
Two separate series, one by Pawlik et al.54 and another by Vauthey et al.,55 have demonstrated an association of oxaliplatin with sinusoidal dilation and irinotecan with steatohepatitis. In the series by Vauthey et al.,55 the presence of steatohepatitis in patients who had undergone resection was correlated to increased 90-day mortality (14.7% vs. 1.6%; P = .001; odds ratio [OR] = 10.5; 95% CI, 2.0 to 36.4). Given these findings, Shindoh et al.56 performed a retrospective analysis on a series of 194 patients with colorectal liver metastasis to determine the optimal FLR for patients treated with neoadjuvant chemotherapy. The authors found that both long duration of chemotherapy (defined as >12 weeks) and sFLR less than or equal to 30% were predictors of hepatic insufficiency (OR = 5.4, P = .004; OR 6.3, P = .019, respectively) (Fig. 31.6). No cases of postoperative mortality and only two cases of postoperative hepatic insufficiency were reported if the sFLR is greater than 30%, indicating that an sFLR greater than 30% may be a more appropriate cutoff value in patients who have received neoadjuvant chemotherapy, particularly if the duration of treatment is more than 12 weeks.
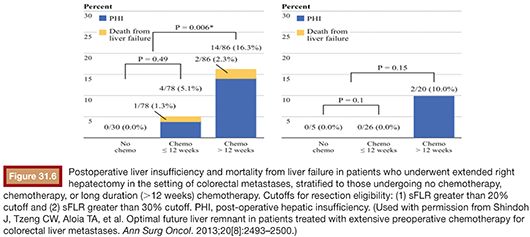
In addition, the effect of systemic neoadjuvant chemotherapy on liver hypertrophy after PVE has been addressed by several studies. Zorzi et al.57 reviewed FLR hypertrophy after PVE in patients with colorectal liver metastases who underwent PVE either with concomitant neoadjuvant chemotherapy (n = 43) or without chemotherapy (n = 22) before resection. The chemotherapy group, which included 26 patients treated in part with the vascular endothelial growth factor (VEGF) receptor blocker bevacizumab, demonstrated similar rates of hypertrophy when compared to the no chemotherapy group at 4 weeks after PVE. Similarly, Covey et al.58 also reported on patients with colorectal liver metastases who underwent PVE either with (n = 47) or without (n = 53) neoadjuvant chemotherapy. Both groups showed no significant difference in median contralateral liver growth after PVE. However, in a small series of 15 consecutive patients by Beal et al.,59 the volume increase of the anticipated FLR was reduced in the setting of chemotherapy (median of 89 vs. 135 mL, range of 7 to 149 vs. 110 to 254 mL; P = .016).
Chemotherapy has not been proven to prevent progression of disease between PVE and resection. A recent study examined the effect of chemotherapy on disease progression between the first and second stages of a two-stage hepatectomy.60 Of the initial 47 patients who underwent first-stage resection, 25 patients (53.2%) were treated with subsequent chemotherapy compared to 22 (46.8%) patients who did not receive interval chemotherapy. Eleven patients (23.4%) failed to complete second-stage hepatectomy due to progression of disease. There was no statistically significant difference in the number of patients with progression of disease between the groups treated or not treated with interval chemotherapy (n = 12 vs. n = 13; P = .561). The authors concluded that chemotherapy after stage 1 resection does not guarantee lower progression of disease rates, within the limitation that the study groups were not randomized.
TWO-STAGE HEPATECTOMY AND OUTCOMES OF EMBOLIZATION VERSUS LIGATION OF THE PORTAL VEIN
Two-stage hepatectomy has been developed to increase the number of patients with bilobar colorectal liver metastasis amenable for resection.61 During the first-stage treatment, tumor within the projected FLR is resected or, in some cases, ablated. Once the FLR is cleared of tumor, portal blood flow is directed toward the FLR either by portal vein ligation (PVL) or PVE of the ipsilateral tumor-bearing liver. Once adequate FLR hypertrophy is achieved, the second-stage hepatectomy targets the remainder of liver metastases, typically requiring a right or extended right hepatectomy. Brouquet et al.52 studied the outcomes of 65 patients with colorectal metastases who underwent first-stage hepatectomy, 47 of whom completed the second-stage resection in comparison to nonsurgical patients. Both study groups had disease confined to the liver and demonstrated an objective response to systemic chemotherapy. The overall 5-year survival rate of the surgical group was 51% compared to 15% for the medical group (P = .005). For the 47 patients who completed the second-stage resection, 5-year survival was improved to 64%. Hence, resection conferred a clear additional survival benefit.
As an alternative to PVE, intraoperative right PVL has been performed during the initial stage of two-stage hepatectomy or as a separate surgical intervention as a means of inducing FLR hypertrophy.62–64 Results from comparative studies between PVE and PVL are mixed, with some studies demonstrating comparable liver hypertrophy, while others showing a liver hypertrophy benefit from PVE.
Both Aussilhou et al.65 (PVE: n = 18; PVL: n = 17) and Capussotti et al.66 (PVE: n = 31; PVL: n = 17) retrospectively compared patients who underwent PVE with patients who underwent PVL during the first stage of a two-stage hepatectomy and found comparable increases in left liver volume in the two groups. Other studies have found inferior FLR hypertrophy after PVL compared to PVE. Broering et al.67 found that increase in left lateral liver volume was significantly higher for the PVE (n = 17) group compared to PVL (n = 17) (188 ± 81 mL vs. 123 ± 58 mL; P = .012) before extended right hepatectomy. In addition, hospital stay was significantly shorter for PVE compared to PVL (4 ± 2.9 days vs. 8.1 ± 5.1 days; P < .01). Robles et al.68 also compared left lobe hypertrophy in two-stage hepatectomy patients who underwent PVL (n = 23) versus PVE (n = 18). This group found that PVE resulted in improved median percentage increase of the FLR compared to PVL (40% vs. 30%, P < .05).68 The inferior hypertrophy after PVL may be explained by portal–portal shunts, which can lead to recanalization of the ligated right portal vein.69
CONTRAINDICATIONS
PVE is as an adjunctive procedure to major hepatectomy. Hence, contraindications to PVE mirror those of hepatectomy. Severe portal hypertension precluding surgery is the only absolute contraindication to PVE. Also, in cases where tumor obstructs the portal system in the liver to be resected, PVE is not necessary as portal flow is already redirected to the FLR.70,71 Relative contraindications include uncorrectable coagulopathy, renal failure, and extrahepatic metastasis. Two-stage hepatectomy has expanded the patients with bilobar hepatic disease burden eligible for PVE and potential curative resection as will be further detailed in the following discussion; however, diffuse hepatic disease burden remains a contraindication to PVE.
TECHNICAL CONSIDERATIONS
Portal Venous Anatomy and Access Routes
Knowledge of standard portal vein anatomy and common variations is essential for both PVE and surgical planning.71 In standard anatomy, the splenic and superior mesenteric veins join to form the main portal vein, which divides into the left and right branches at the hepatic hilum. The right portal branch subdivides into anterior and posterior divisions, which supply Couinaud segments 5/8 and 6/7, respectively. The left portal branch subdivides into branches, which supply segments 4, 3, and 2. In one series of 200 patients studied with CT portography, standard anatomy (type I) was identified 65% of the time (Fig. 31.7).71,72 In the most common variation (type III), the right posterior portal vein is the first branch of the main portal vein, followed by bifurcation of the right anterior and left portal branches.
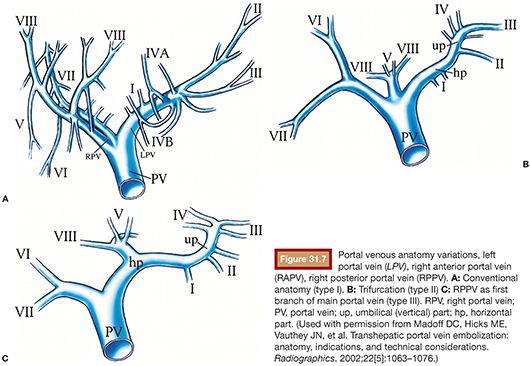
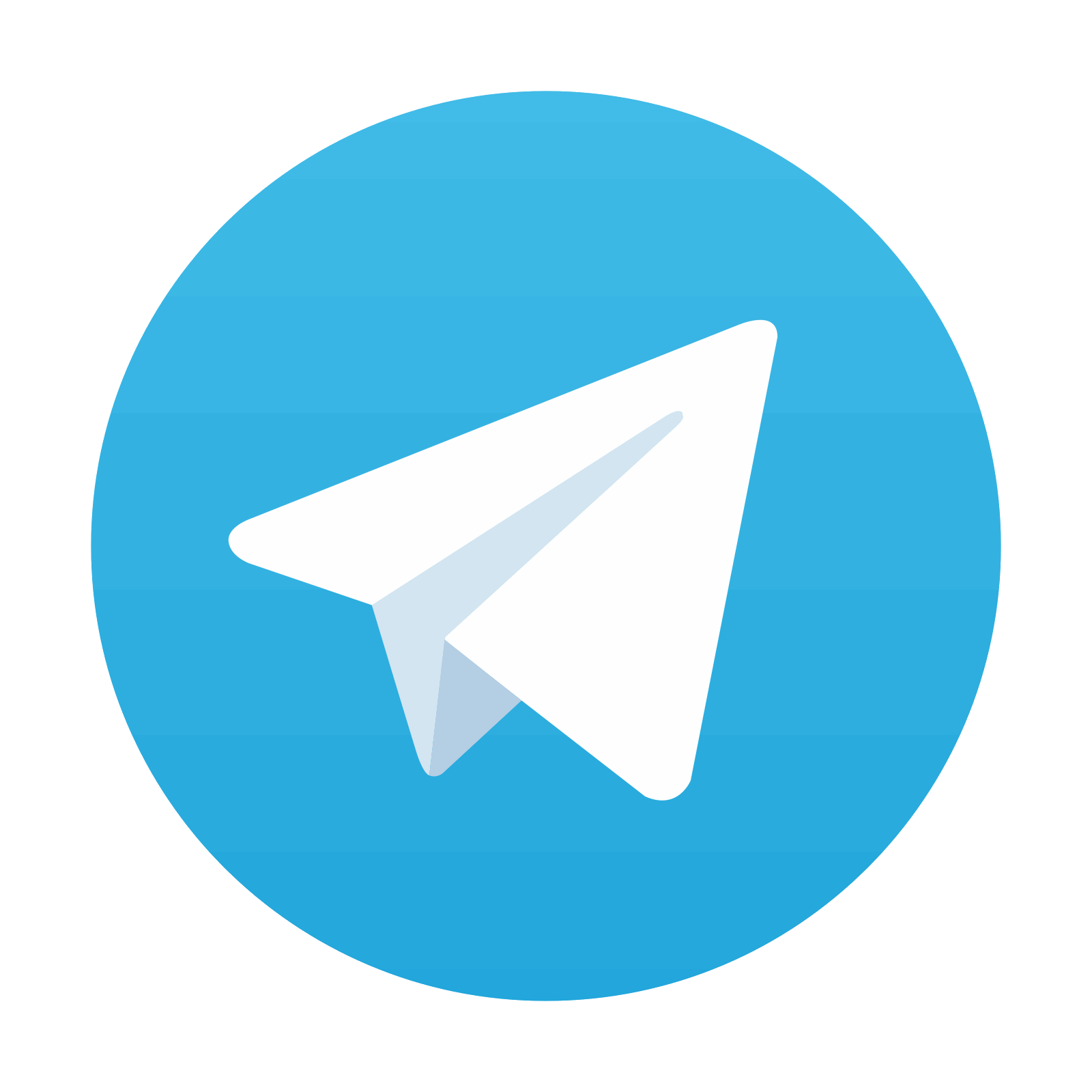
Stay updated, free articles. Join our Telegram channel

Full access? Get Clinical Tree
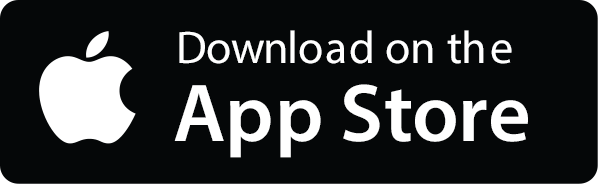
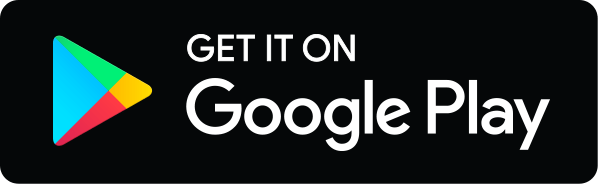