Posterolateral (PLC) and posteromedial (PMC) corners of the knee represent complex anatomic regions because of intricate soft tissue and osseous relationships in small areas. Concise knowledge of these relationships is necessary before approaching their evaluation at imaging. Magnetic resonance imaging offers an accurate imaging diagnostic tool to establish normal anatomy and diagnose and characterize soft tissue and osseous injury. It is important to carefully evaluate the PLC and PMC structures on magnetic resonance imaging before planned surgical intervention to avoid potential complications resulting from occult injury.
Key points
- •
Posterolateral and posteromedial corners of the knee are complex anatomic regions; detailed knowledge of anatomic structures and relationships is necessary for appropriate assessment at imaging.
- •
Association between untreated posterolateral and posteromedial corner injuries of the knee and failure of central support structure reconstruction has been established. Accurate diagnosis and characterization of these injuries will improve clinical and surgical outcome.
- •
Posterolateral and posteromedial corner injuries of the knee may be difficult to diagnose clinically. Classic magnetic resonance imaging patterns allow noninvasive diagnosis that can guide management.
Introduction
The posterolateral (PLC) and posteromedial (PMC) corners of the knee are anatomic units composed of a complex arrangement of structures. As referenced in their names, they extend both posteriorly and along the lateral and medial aspects of the knee, respectively ( Fig. 1 ). As the posterior extension of the lateral and medial supporting structures, they act in conjunction with the central supporting ligaments (anterior and posterior cruciate ligaments) to provide static (capsular and noncapsular ligaments) and dynamic (musculotendinous units and their aponeuroses) articular stability. The delineation of fine anatomic detail in these regions and identification of delicate structures are particularly challenging at imaging because of composite anatomy, orientation, and small size of their components. Pathology might be overlooked or misdiagnosed without clear knowledge of the regional morphology, biomechanics, and specific patterns of injury. Moreover, PLC and PMC injuries uncommonly occur in an isolated fashion, more often associated with concomitant injuries that may dominate the clinical picture. Untreated PLC injuries can lead to chronic posterolateral instability and PMC deficiencies may cause persistent valgus instability ; both conditions lead to poor outcome of anterior cruciate ligament (ACL) and posterior cruciate ligament (PCL) reconstruction. It is therefore imperative, in a postinjury or preoperative setting, to provide the referring physician with an accurate description (when possible) of the PLC and PMC structures. The characterization of structural alteration at imaging will allow a reference for clinical evaluation that can guide surgical approach and interrogation, facilitating optimal treatment, and thereby improving patient outcome. Magnetic resonance imaging (MRI) currently is the gold standard imaging strategy in the evaluation of the soft tissues; therefore, the authors focus mainly on this technique. Ultrasound scan has been established as a complementary technique. Plain films and computed tomography play a role in the evaluation of osseous lesions (bony avulsions, cortical stress changes related to chronic injury). This article discusses the anatomy, basic biomechanics, and common injuries of the PLC and PMC complexes, presenting the reader with a systematic approach for their evaluation.
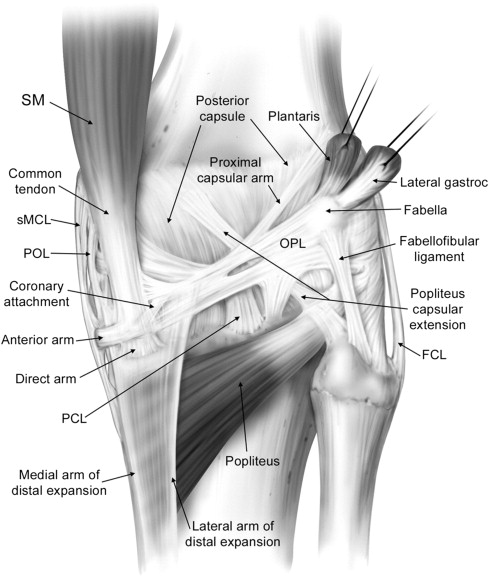
Introduction
The posterolateral (PLC) and posteromedial (PMC) corners of the knee are anatomic units composed of a complex arrangement of structures. As referenced in their names, they extend both posteriorly and along the lateral and medial aspects of the knee, respectively ( Fig. 1 ). As the posterior extension of the lateral and medial supporting structures, they act in conjunction with the central supporting ligaments (anterior and posterior cruciate ligaments) to provide static (capsular and noncapsular ligaments) and dynamic (musculotendinous units and their aponeuroses) articular stability. The delineation of fine anatomic detail in these regions and identification of delicate structures are particularly challenging at imaging because of composite anatomy, orientation, and small size of their components. Pathology might be overlooked or misdiagnosed without clear knowledge of the regional morphology, biomechanics, and specific patterns of injury. Moreover, PLC and PMC injuries uncommonly occur in an isolated fashion, more often associated with concomitant injuries that may dominate the clinical picture. Untreated PLC injuries can lead to chronic posterolateral instability and PMC deficiencies may cause persistent valgus instability ; both conditions lead to poor outcome of anterior cruciate ligament (ACL) and posterior cruciate ligament (PCL) reconstruction. It is therefore imperative, in a postinjury or preoperative setting, to provide the referring physician with an accurate description (when possible) of the PLC and PMC structures. The characterization of structural alteration at imaging will allow a reference for clinical evaluation that can guide surgical approach and interrogation, facilitating optimal treatment, and thereby improving patient outcome. Magnetic resonance imaging (MRI) currently is the gold standard imaging strategy in the evaluation of the soft tissues; therefore, the authors focus mainly on this technique. Ultrasound scan has been established as a complementary technique. Plain films and computed tomography play a role in the evaluation of osseous lesions (bony avulsions, cortical stress changes related to chronic injury). This article discusses the anatomy, basic biomechanics, and common injuries of the PLC and PMC complexes, presenting the reader with a systematic approach for their evaluation.
Posterolateral corner of the knee
Anatomy, Biomechanics, and Mechanism of Injuries to the Posterolateral
Seebacher and colleagues in 1982 introduced a three-layered approach in the anatomic description of the lateral supporting structures of the knee, using a similar three-layer concept previously assumed in their description of the medial side supporting structures ( Fig. 2 ). In Seebacher’s original description of the lateral supporting structures, the superficial layer (I) consists of the iliotibial tract and its expansion anteriorly and the biceps femoris tendon (BFT) and its expansion posteriorly. The peroneal nerve lies deep to it, posterior to the BFT. The middle layer (II) is incomplete and consists of the lateral patellar retinaculum anteriorly and the 2 patellofemoral ligaments posteriorly. The proximal patellofemoral ligament joins the lateral intermuscular septum, the distal patellofemoral ligament attaches to the fabella (when present) or at the femoral insertion of the posterolateral joint capsule or lateral head of the gastrocnemius. Included in the middle layer is the patellomeniscal ligament, which travels from the patella to the lateral meniscus, reaching inferiorly the lateral tibial tubercle of Gerdy, running deep to the iliotibial tract. The deepest layer (III) is composed of the lateral extent of the joint capsule and is attached to the edges of the tibia and femur. Posterior to the overlying iliotibial tract, the capsule divides into 2 laminae (superficial and deep). The superficial lamina travels superficial to the lateral collateral ligament and ends posteriorly at the fabellofibular ligament. The deeper lamina travels deep to the lateral collateral ligament, passes along and attaches to the edge of the lateral meniscus, giving rise to the coronary ligament and ultimately reaching the arcuate ligament. The deep and superficial laminae of the posterolateral capsule are always separated from each other with the lateral inferior genicular artery, considered an anatomic landmark, between them (see Fig. 2 ). Further cadaveric, MRI and ultrasound scan evaluations of specific PLC anatomy followed Seebacher’s original work to delineate the imaging counterpart of his anatomic description. Because of the increasing interest in the anatomy and biomechanics of the PLC corner of the knee in the orthopedic literature, the need for standardization and a systematic approach to the nomenclature of the lateral complex structures and PLC has been addressed. For a systematic approach to these structures we consider the superficial layer (first layer) comprising the lateral fascia, iliotibial band and biceps femoris tendon. The middle layer (second layer) comprises the patellar retinaculum, and patellofemoral and patellomeniscal ligaments. The deep layer (third layer) consists of the lateral collateral ligament (fibular collateral ligament), the lateral coronary ligament (lateral meniscotibial ligament), the arcuate ligament , the popliteus tendon-muscle unit , the popliteofibular ligament , the fabellofibular ligament , and the lateral joint capsule with its attachment to the lateral meniscus edge. The deep layer is the most anatomically variable of the 3 layers and the one constituting the posterolateral corner of the knee complex. We briefly describe the components of the third inner layer and their anatomic relations.
The lateral collateral ligament (fibular collateral ligament), is an extracapsular structure, has a tubular shape, and measures approximately 3–4 mm in diameter and 7 cm in length. It originates from the lateral femoral condyle, arising from a small depression posterior to the lateral femoral epicondyle and 2 cm proximal to the joint line. It courses distally and posteriorly to attach to the posterior portion of the lateral aspect of the fibular head (see Fig. 1 ).
The lateral coronary ligament (or meniscotibial portion of the midthird lateral capsular ligament or lateral meniscotibial ligament) attaches the lateral meniscus to the lateral tibial plateau and functions as a meniscal stabilizer. The lateral coronary ligament is composed of short confluent ligamentous bands attached to the peripheral portion of the meniscal body and to the lateral tibia several millimeters inferior to the articular surface, occasionally resulting in a small synovial recess.
The arcuate ligament has an inverted Y shape. The 2 superior arms consist of a lateral limb (upright), coursing upward along the joint capsule and extending to the lateral femoral condyle, and a medial limb (arcuate), crossing over the popliteal tendon, attaching to the posterior capsule, and merging with the oblique popliteal ligament. The lower limb attaches to the fibular head. The arcuate ligament lies deep to the lateral inferior genicular artery, and its presence is variable, ranging from 24% to 80% in previous studies.
The popliteus muscle originates from the lateral aspect of the lateral femoral condyle, attaches to the posterior horn of the lateral meniscus via the popliteomeniscal fascicles (anteroinferior, posterosuperior, posteroinferior) and to the apex of the fibula via the popliteofibular fascicles, extending distally to the posteromedial aspect of the tibial shaft (see Fig. 1 ). The popliteus muscle is always present, whereas the posteroinferior popliteomeniscal fascicle may be absent.
The popliteofibular ligament originates at the level of the musculotendinous junction of the popliteus muscle and courses distally and laterally to insert on the fibular styloid. The popliteofibular ligament is a wide tendinous band, approximately the same width or wider than the popliteus tendon, and is reportedly present in between 94% and 98% of the population. The proximal myotendinous portion of the popliteus muscle and the popliteofibular ligament originating from it form an inverted Y-shaped structure. Therefore, the 3 arms of the inverted Y are the superior portion of the popliteus myotendinous unit, the popliteofibular ligament, and the inferior portion of the popliteus myotendinous unit distal to the origin of the popliteofibular ligament.
The fabellofibular ligament is the distal edge of the capsular arm of the short head of the biceps femoris muscle, and, as such, is present in all knees. Usually, it is a delicate structure but is more robust in appearance when the bony fabella is present but less consistent and more subtle in nature when a cartilaginous fabellar analog is present. We would like to reinforce the concept addressed from LaPrade regarding the presence of at least a fabellar cartilaginous analog in every knee. When the fabella is present, the fabellofibular ligament arises from the lateral margin of the fabella, and, when absent, it originates from the posterior aspect of the supracondylar process of the femur. Extending from the fabella or the fabellar analog, the fabellofibular ligament extends toward the fibula, parallel to the lateral collateral ligament, and inserts distally on the lateral aspect of the tip of the fibular head styloid process, posterior to the fibular insertion of the biceps femoris tendon (see Fig. 1 ). Previous studies addressed the variable presence of the fabellofibular ligament, ranging from 51%–87%.
Biomechanically the PLC structures resist varus and external rotation forces. With regard to varus forces the lateral collateral ligament is considered the major stabilizer, with a minor contribution from the posterior cruciate ligament and the posterolateral capsule; a minor secondary contribution is made from the anterior cruciate ligament. In relation to external rotation forces, the lateral collateral ligament, popliteofibular ligament, fabellofibular ligament, capsular attachment of the short head of the biceps femoris muscle, and the popliteus tendon play a fundamental role in resisting stress. A role of the PLC in resisting posterior translation of the tibia has been addressed, establishing the popliteomeniscal fascicles as stabilizers of the posterior horn of the lateral meniscus. Isolated injuries of the PLC tend to be rare and commonly occur in conjunction with cruciate ligament lesions. The association of lateral collateral ligament and deep PLC structure injuries increases varus angulation and tibial external rotation, also causing anteroposterior instability of the knee. Deep PLC lesions (preserved lateral collateral ligament), in conjunction with anterior cruciate ligament deficiency, increase anterior translation of the tibia without causing external rotation of the tibia or varus angulation of the joint. When deep PLC structures fail and lateral collateral ligament and anterior cruciate ligament injuries are present, anterior and varus instability with external rotation of the tibia occur. Deep PLC structure deficiency, with lateral collateral ligament and posterior cruciate ligament injury, cause posterior translation, varus angulation, and external rotation of the tibia. Posterolateral corner injuries are frequently associated with acute posterior cruciate ligament tears and have been reported in 62% of patients. Therefore, when a posterior cruciate ligament injury is observed, particular attention should be paid to the PLC area. Injuries of the PLC are less common than injuries of the PMC, but because this anatomic area is subject to a greater stress during motion than the medial side, they tend to be more disabling. PLC injuries most commonly occur via a direct blow to the anteromedial aspect of the proximal tibia in the fully extended knee, with the force directed in a posterolateral direction, but can also occur from a hyperextension injury with external rotation. Anterior rotatory dislocations (varus stress and hyperextension) and posterior rotatory dislocations (varus stress, posteriorly directed blow to proximal tibia and flexion), are also common mechanisms of injury, the latter known as a “dashboard injury.” An undetected PLC injury can lead to chronic instability and failure of efforts to reconstruct the central supporting structures because of deficiency of PLC in resisting biomechanical stress. Testing of the PLC resistance to stress involves varus and external rotation stress tests at different degrees of flexion. The posterolateral rotation test (dial test) is one commonly used for posterolateral instability, assessing increasing external rotation of the tibia in relation to the femur at 30° of knee flexion.
Injuries to the posterolateral complex
Soft-tissues Injuries of the Posterolateral
Lateral collateral ligament (fibular collateral ligament)
Alterations to the lateral collateral ligament are a common feature of PLC injuries. In vitro studies addressed the role of the lateral collateral ligament (LCL) as a restraint to PLC instability and varus angulation in static testing. Nielsen and colleagues noted an increase in varus joint opening with marked posterolateral rotatory instability when an LCL lesion is associated with a posterolateral capsule transection rather than an LCL injury alone. Grood and coworkers addressed the increase of varus angulation at partial knee flexion when a PCL injury is combined with an LCL injury. Gollehon and colleagues showed the principal role of the LCL and PLC deep ligament complex in preventing varus and external rotation of the tibia and the increase of varus rotation and posterior translation in combined injuries of the LCL, PCL, and deep lateral complex. However, LaPrade and Terry evaluated 71 patients presenting with a PLC knee injury and signs of instability but at surgery found an injured LCL in only 23% of the knees. Based on this finding, they suggest that an LCL injury should not be the sole determining factor when diagnosing PLC injuries. Lateral collateral ligament injuries consist of structural alterations that include thickening, tears, soft-tissue avulsions from the femoral attachment, and soft-tissue avulsions (with or without a bony component) from the fibular head. LCL injuries are best visualized in the axial and coronal plane at MRI examination ( Fig. 3 ).
Popliteus muscle and its tendon
Injuries to the popliteus muscle can be intra-articular (at the femoral insertion or at the level of the popliteal hiatus) or extra-articular (muscular or myotendinous portion) in nature, the latter being more frequent. An avulsion at the femoral attachment may appear as an irregular contour of its tendon at the level of the popliteal hiatus with surrounding edema. Partial tears of the myotendinous junction present with increased T2 signal at the level of the muscle-tendon junction on fat suppressed, fluid-sensitive sequences at MRI examination. Complete tendon tears may present as an interruption of the muscle belly appearing as a masslike lesion with surrounding edema. Enlargement of the muscle belly or disruption of muscle fibers may be apparent. Only 8% of all popliteus injuries occur in an isolated fashion, with isolated cases of muscle or tendon lesions reported in skiers, football, soccer, rugby, and polo players. Lesions of the popliteus muscle are usually combined with injuries to other knee structures. Brown and colleagues, in their MRI analysis of popliteus injuries (n = 24), reported combined injuries in 92% of cases, with involvement of its muscular portion in 96% of patients. Associated injuries included ACL (17%) or PCL (29%) tears, combined medial (46%) or lateral (25%) meniscal injuries, and medial (8%) or lateral (4%) collateral ligament lesions. Bone bruises or fractures were reported in 33% of patients. Popliteus muscle injuries are best evaluated on the axial and coronal planes ( Fig. 4 ), and diagnosis of abnormalities on MR imaging plays a fundamental role in the diagnosis of an injury to the popliteus muscle because of the difficulty in assessing this structure at arthroscopy.
Popliteofibular ligament
Visualization and assessment of the popliteofibular ligament can be challenging on MRI. Standard imaging planes (coronal, sagittal, and axial) may depict the structure, but visualization is not always optimal because of its oblique orientation and the delicate nature of the structure; coronal and sagittal planes are in our practice the most useful planes for assessment ( Fig. 5 ). Some advocate the use of a coronal oblique plane, oriented parallel to the direction of the popliteus tendon, with one study showing visualization of the popliteofibular tendon improving from 8% to 53% of the knees. Another study compared coronal oblique fat-saturated T2 with isotropic three-dimensional water excitation double-echo steady state (WE-DESS) sequences. The latter sequence improved the identification of the popliteofibular ligament from 71% to 91% of cases.
Injuries to the popliteofibular ligament consist of ligamentous disruption, avulsion from the fibular insertion, partial tearing, and intrasubstance degeneration in the form of signal alteration within the tendon. Surgical reconstruction of a disrupted popliteofibular ligament has been found to be beneficial in patients with posterolateral external rotatory instability of the knee.
Arcuate ligament
The variable presence and subtlety of the arcuate ligament makes assessment difficult and makes recognizing injuries of the arcuate ligament complex a challenging proposition. The anatomy of the arcuate ligament is debated and can be considered a thickening of the posterolateral capsule. On sagittal images, the arcuate ligament is best identified on images on which the popliteus tendon and fibular tip are both visualized. The straight limb of the arcuate can be found superficial to the popliteus tendon as a delicate linear low-signal structure attaching to the fibular tip ( Fig. 6 ). Dedicated imaging of the PLC in the coronal oblique plane may significantly improve visualization of the arcuate ligament, with an increase from 10% to 46% reported by Yu and colleagues compared with standard coronal imaging.
Increased signal at the level of the posterolateral capsule on fat-saturated fluid-sensitive images should raise concern for capsular disruption and the possibility of associated injury or tear of the arcuate ligament ( Fig. 7 ). Injury to the posterolateral capsule has been suggested as one reason why PLC injuries can occasionally present without a significant knee joint effusion. Baker and coworkers operated on 13 patients with acute PCL injuries and posterolateral instability of the knee, identifying tears of the arcuate ligament complex in all 13. Their results suggest that surgical repair of the arcuate ligament complex improves patient outcome in PCL injuries with posterolateral instability, emphasizing the importance of evaluating this structure on preoperative imaging.
Fabellofibular ligament
The fabellofibular ligament is best visualized on MRI in the coronal plane and is located posteriorly to the lateral collateral ligament and at the far posterior tip of the fibular styloid. Yu and colleagues compared visualization of the fabellofibular ligament in the coronal oblique plane with the standard coronal plane imaging and reported an increase in visualization from 34% to 48% on coronal oblique images. The fabellofibular ligament was seen in only 4% in the sagittal plane. Injuries of the fabellofibular ligament include degeneration, tearing and avulsion from the fibular tip (see Fig. 7 ). Avulsion of the fabellofibular ligament can be associated with an avulsive injury of the direct arm of the short head of the biceps femoris tendon. The inferior lateral genicular artery is a branch of the popliteal artery, which can be used as an anatomic landmark in evaluating the fabellofibular ligament, as it passes around the posterior joint capsule laterally, running anterior to the fabellofibular ligament and posterior to the popliteofibular ligament.
Soft tissue Segond injury (lateral meniscotibial capsular injury)
The soft tissue Segond injury is a soft-tissue avulsion injury described by LaPrade and colleagues, consisting of disruption of the conjoined tibial attachment of the anterior arm of the short head of the biceps femoris muscle and the meniscotibial portion of the midthird lateral capsular ligament, with associated proximal retraction or thickening (see Fig. 3 ; Fig. 8 ).
Myotendinous injuries of the lateral head of gastrocnemius
The proximal myotendinous portion of the lateral gastrocnemius muscle is commonly not included in the classic list of the specific anatomic structures composing the PLC. However, different factors determine its participation in knee stability provided by the posterolateral corner structures. Based on several anatomic relationships, it is clear that this structure serves as an important secondary dynamic stabilizer of the PLC. This is supported by its involvement in accommodating the fabella (or its cartilaginous equivalent) with its attachment to the fabellofibular ligament. Furthermore, the lateral gastrocnemius reinforces the meniscofemoral capsule and is firmly attached to the lateral femoral condyle at the level of the supracondylar process. Clinically, the stabilizing role of the lateral gastrocnemius is recognized in PLC reconstruction, including advancement procedures.
Injuries to the gastrocnemius muscle usually involve the distal myotendinous junction of the medial gastrocnemius (tennis leg). Although primary injuries of the lateral gastrocnemius are rare, the lateral gastrocnemius should be evaluated carefully in cases of posterolateral corner injury because of the secondary stabilizing role of this structure. The lateral gastrocnemius is usually best seen on sagittal images.
Osseous Alterations Associated with Posterolateral Injuries
Arcuate fracture
This injury is an avulsion fracture at the level of the fibular head ( Fig. 9 ). If a pattern of diffuse fibular head edema is present at MRI examination, the injury usually involves the distal lateral collateral ligament insertion and the distal insertion of the biceps femoris tendon to the fibula (LCL and BFT distally form the conjoint tendon). When edema is localized at the medial aspect of the fibular head, the arcuate ligament or popliteofibular insertions are usually involved. Plain films (arcuate sign) and computed tomography (CT) show the bony avulsion at the fibular tip; MRI has a role in depicting ligamentous injuries. The authors advocate the presence of fibular edema at the level of the fibular head on MRI as a diagnostic clue. In their MRI evaluation of 19 knees presenting with an arcuate sign at conventional radiographic examination, Juhng and colleagues, reported a tear of the posterolateral capsule in 67% of the cases and an injury to the cruciate ligaments in 89% (16 knees) of the cases: 9 knees with a combined ACL and PCL injury, 4 knees with isolated ACL, and 3 knees with isolated PCL injuries. A bone bruise or fracture was present in all cases, with 50% showing an anteromedial femoral condyle bone bruise and 28% showing the same feature at the anteromedial tibia. A meniscal tear was present on the medial or lateral side in 28% and 22% of the cases, respectively. An injury of the popliteus muscle was evident in 33% of the cases, and all patients had a joint effusion. Huang and coworkers, in their MRI evaluation of 13 knees presenting with an arcuate sign on plain film, found that in 85% of the patients the avulsed bony fragment from the fibula originated either from the attachment of the popliteofibular ligament or the attachment of the popliteofibular, arcuate, and fabellofibular ligaments at the posterosuperior aspect of the fibular styloid process. All patients presented with both PCL and medial collateral ligament (MCL) injury, but no ACL injuries were reported. A popliteus tendon tear was present only in one case, and 77% of the cases had an injury to the arcuate ligament complex, although integrity of the arcuate, popliteofibular, and fabellofibular ligaments could not be fully assessed. The medial meniscus was injured in 38% of the knees and the lateral meniscus in 46%, with arthroscopic confirmation. Bone marrow edema was present in 38% of the patients (anterior lateral tibial plateau, medial tibial plateau, lateral femoral condyle, medial femoral condyle, patella, posterior tibial plateau and fibular head).
