Invasive vascular imaging is based on the technique described by Sven Ivar Seldinger in 1953 ( Fig. 7.1 ). This elegant innovation, now known by Seldinger’s name, eliminated the need for surgical exposure of a blood vessel before catheterization, thus allowing the transfer of angiography from the operating room to the radiology department. Virtually all vascular invasive procedures and devices use this technique.
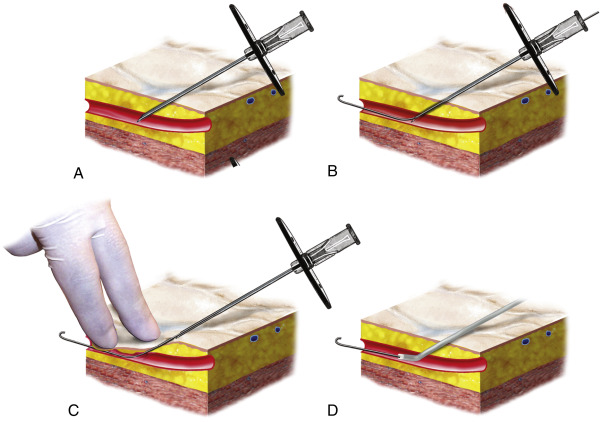
Preprocedural Patient Evaluation and Management
Every invasive procedure begins with a patient evaluation, determination of the appropriateness of the examination, and formulation of a procedural plan. In most cases the angiographer performing the procedure will have seen the patient previously in consultation and assumed primary responsibility for management of the disease to be diagnosed. A brief directed history should be obtained, with attention to the symptoms or signs that precipitated the study. Essential historical areas to cover include prior surgical procedures (especially vascular); evidence of atherosclerotic disease in “index” vascular beds, such as prior myocardial infarction or stroke; diabetes, with attention to medications; status of renal function; allergies; and known previous exposure to iodinated contrast agents. Office records or the patient’s chart should be reviewed for similar information. Special attention should be applied to operative notes and reports from previous angiograms because these provide valuable information that may alter the entire approach to the procedure. Most importantly, personal review of old angiograms or correlative imaging is essential before embarking on an invasive procedure.
The preprocedural physical examination is focused on the status of the vascular system and selection of a vascular access site. The person who will perform the procedure should conduct this examination. The quality of the pulses and the presence of an aneurysm (as suggested by a broad prominent pulse) should be recorded using a consistent system. Suspected integumentary infection, fresh surgical incisions, a large abdominal pannus, or a scar over the vessel all impact selection of an access site. Pulses distal to the anticipated access site must be evaluated because one of the potential complications of angiography is distal embolization. Furthermore, if an intervention is performed, this baseline information is important to help determine procedural endpoints. The physical examination should include both right and left sides of the patient so that a different access site can be used during the procedure if necessary. When an upper extremity approach is anticipated, the brachial blood pressure in both arms must be obtained.
Patients should be well hydrated before the procedure. Outpatients should not be instructed to fast after midnight but encouraged to drink clear liquids until 2 hours before their scheduled appointment. In the preprocedural area, an intravenous infusion of 5% dextrose in 0.5% normal saline should be begun at 100 mL/h in normal patients. Fluid rates and characteristics should be adjusted in diabetic patients, patients on dialysis, and patients with congestive heart failure. Inpatients should have an established intravenous infusion in place before arriving in the angiographic suite. Most hospitals have established guidelines for oral intake before invasive procedures that must be followed, but remember that these are generally not designed for patients about to receive large doses of nephrotoxic contrast materials.
There are no laboratory studies that are absolutely necessary before starting an invasive vascular procedure; most problems that can be predicted from abnormal laboratory studies occur after the catheter is removed (e.g., bleeding, renal failure). A low platelet count is the single most important predictor of postprocedural bleeding complications. The commonly acquired minimal laboratory studies are coagulation (international normalized ratio [INR], prothrombin time, activated partial thromboplastin time, and platelet count) and serum creatinine value. Patients with renal failure undergoing central venous procedures that might entail intracardiac manipulation (e.g., central line placement) may require measurement of serum potassium concentration.
When the prothrombin time or INR is abnormal, fresh frozen plasma given the day or night before is useless or even dangerous because an INR drawn just after the plasma has been infused may be normal, but by the time the procedure is performed, the effect may dissipate. Fresh frozen plasma infused shortly before and during the procedure provides maximal correction when it is needed most. An abnormal activated partial thromboplastin time is usually due to administration of unfractionated heparin, which can be turned off when the patient arrives in the angiography suite. Because the half-life of unfractionated heparin is about 90 minutes, most patients will correct sufficiently for manual compression by the end of the procedure. Perhaps more importantly, platelet transfusion to restore a count to more than 50,000/μL is an empirical cutoff used in many departments for patients having an arterial access.
In the presence of an abnormal serum creatinine value, the risk of postprocedural renal failure should be weighed against the benefits of the procedure. Every hospital and practice should have guidelines for contrast administration to patients with abnormal renal function. Regardless of the renal protective strategy, the patient should be well hydrated before and after the examination. Renal protective strategies should be followed to maximize renal protection.
Basic Safety Considerations
Operator precautions against exposure to body fluids should be applied to all situations, even for patients with no known risk factors. Masks, face shields or other protective eyewear, sterile gloves, and impermeable gowns are the minimal measures. Closed flush and contrast systems decrease the risk of splash exposures. All materials used during the case should be disposed of in waste containers designed and labeled for biological waste.
Sharp devices (e.g., needles, scalpels) should be carefully stored on the work surface in a red sharps container or removed immediately after use. Recapping needles is not advised owing to the puncture risk. The best sharps containers contain a foam block into which the point of the sharp device can be safely imbedded. At the end of the case, the angiographer can dispose of the sharps in one of the ubiquitous hard red plastic sharps receptacles. Puncture wounds from contaminated needles or scalpels are not only painful but also potentially life-altering events. If an accidental splash, puncture, or any other exposure occurs, immediate consultation with a physician experienced in management of exposure to occupational biohazards is essential.
Radiation exposure to the patient and staff should be kept to a minimum. Use fluoroscopy only when needed to move catheters or guidewires. Prolonged fluoroscopy at high magnification with the x-ray tube in one position has been associated with radiation burns to the patient. Exposure can be reduced during long cases by use of pulsed fluoroscopy modes. The typical pulse rate of 15 pulses per second can be decreased by 50% or more with only a minor degradation in image quality.
Accumulative radiation exposure to the angiographer can be substantial. Angiographers should wear wraparound lead, thyroid shields, leaded glasses, and radiation badges. Careful coning of the x-ray beam during the case can reduce scatter. The operator’s hands should never be seen on the fluoroscope during the case. When the angiographer must remain in the procedure room during filming, portable leaded shields should be positioned between the x-ray source and the physician.
Ergonomic considerations are important during invasive vascular imaging. Many angiographers develop degenerative spine disease in the neck and back. Careful attention to the design of angiographic suites, especially the positioning of controls and monitors, can reduce twisting and bending. Similarly, patients should be positioned on the procedural table to minimize contortions on the part of the operator. The patient’s comfort also requires careful consideration. For long procedures, careful padding of pressure points, especially when the patient is under general anesthesia, is important.
Tools
Access Needle
All angiographic procedures begin with a vascular access needle. There is great variety in vascular access needles, but all are designed to allow introduction of a guidewire through a central channel ( Fig. 7.2 ). The simplest needle is a one-piece open needle with a sharp beveled tip. The guidewire is introduced directly through the needle once the tip is fully within the bleeding vessel lumen. This style of needle can be used for both arterial and venous punctures. Two-piece needles usually have a central sharp stylet that obturates the lumen and extends slightly beyond the needle tip. These needles have a blunted atraumatic beveled tip when the stylet is removed. The sharp stylet allows the needle to puncture the vessel, but once it is removed the risk of vascular injury from the blunt needle tip is theoretically removed. The stylet can be solid or hollow. In the latter case, blood may be visualized on the stylet hub once the vessel lumen is entered. With all styleted needles, the stylet must be removed to insert the guidewire. Needles with stylets are generally used only for arterial punctures. The most common sizes for vascular access needles of the type described above are 19- or 18-gauge in diameter and 2¼ to 5 inches long.
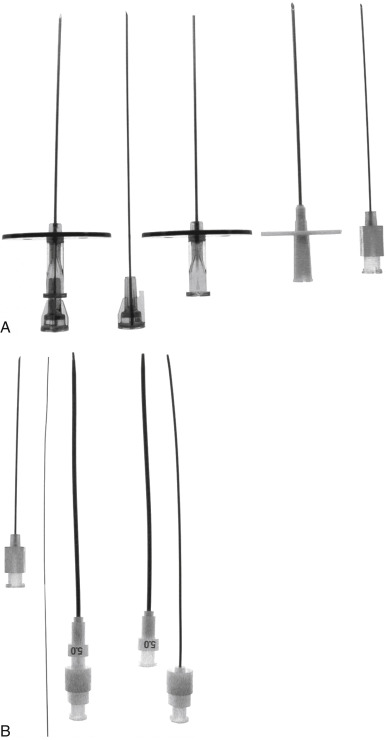
Microaccess systems in which a small-diameter access needle is used to enter the vessel and then converted to a larger short, plastic introducer with a larger lumen are useful for both arterial and venous punctures. These systems usually employ a sharp, open, bevel-tipped 21-gauge needle for access. These needles are often modified to enhance visualization by ultrasound during the access procedure. After entering the blood vessel, a short, floppy-tipped 0.018-inch guidewire is inserted. The needle is removed over the guidewire and exchanged for a 4F or 5F dilator through which a 3F dilator has been coaxially inserted. Once the dilator assembly has been inserted, the 0.018-inch guidewire and the 3F dilator are removed, leaving the dilator with the larger lumen behind.
Guidewires
Guidewires are available in a number of thicknesses, lengths, tip configurations, stiffnesses, and materials of construction ( Fig. 7.3 ). In general, the guidewire thickness (always referred to in hundredths of an inch—e.g., 0.038-inch) should be the same as or slightly smaller than the diameter of the lumen at the tip of the catheter or device that will slide over it. Guidewires that are too big will jam, usually at the tip of the catheter. However, if a guidewire is much smaller than the end hole of the catheter or device, there will be a gap between the guidewire and catheter that can cause vessel injury or prevent smooth movement over the guidewire.
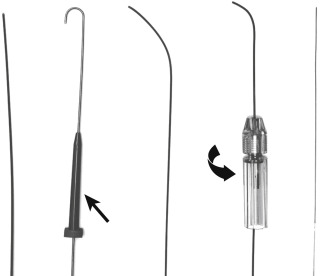
The most commonly used style of guidewire has a central stiff core around which is tightly wrapped a smaller wire, just like a coiled spring ( Fig. 7.4 ). The outer wire is often welded to the core at the back end but not the tip. The purpose of the coiled outer wrap is to decrease the area of contact between the surface of the guidewire and the tissues. A fine safety wire runs along the length of the inside of the guidewire between the inner core wire and outer wrap and is welded to the outer wrap at both ends. This safety wire prevents the outer wrap from unwinding should the weld break. This is where the term safety guidewire originated.
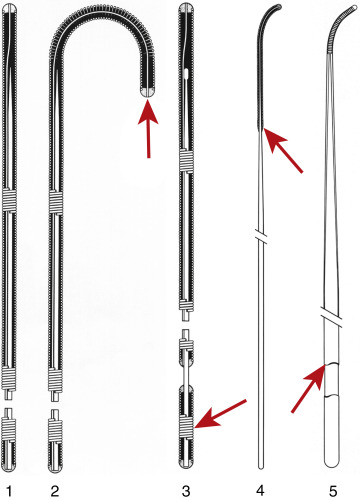
The composition and thickness of the inner core determine the degree of guidewire stiffness. Guidewires that are very flexible are important for negotiating tortuous or diseased vessels. Stiff guidewires provide the most support for introducing catheters and devices. A movable core guidewire is one in which the core is not welded to the tip but can be slid in and out of the spring wrap to adjust the guidewire stiffness as necessary. The mandril guidewire is an important design type in which the outer wrap is limited to the soft tip of guidewire. Most of the guidewire is solid wire. This is a common construction for microguidewires (0.018-inch or smaller) or for extra-rigid large-diameter guidewires.
The features of the taper of the core at the leading end of the guidewire determine the softness or “floppiness” of the tip. The length and transition of the taper define the characteristics of the tip. The longer and more gradual the transition, the longer and floppier the tip. Bentson guidewires and movable core guidewires with the core retracted have the softest tips. With all guidewires, it is the soft end that goes in the patient.
A curved tip at the end of the guidewire provides an additional degree of safety in diseased vessels. As the guidewire is advanced, the rounded presenting part bounces over plaque rather than digging into it. A curve can be added to most straight guidewires by gently drawing the floppy tip across a firm edge (e.g., a fingernail, closed hemostat), much like curling a ribbon. A special type of wire is the tip-deflecting guidewire, which allows the operator to mechanically vary the radius of the curve while in the patient. These guidewires have stiff tips and should never be advanced beyond the end of the catheter.
A wide range of special-purpose guidewires such as wires coated with slippery hydrophilic substances, highly torqueable guidewires, kink-resistant Nitinol-based wires, and microwires are available. These guidewires have been the difference between routine success and failure in many challenging cases. The hydrophilic-coated guidewire is the most commonly used specialty guidewire; its central core is coated with an outer layer of hydrophilic material. This coating drastically reduces friction between the guidewire, blood vessel wall, and catheter. However, unless kept moist, hydrophilic guidewires actually become much stickier than a regular guidewire. When this happens, it is almost impossible to advance a catheter over the guidewire and easy to inadvertently pull the entire guidewire out of the body during an exchange. Of note, these guidewires should not be inserted through vascular access needles because the nonradiopaque coating can be easily sheared off by the metal edge of the needle if withdrawn.
The length of the average guidewire used in angiography is 145 to 160 cm. In circumstances in which a great deal of guidewire is needed inside the body, or the devices and catheters to be placed over the guidewire are very long, an “exchange-length” guidewire (260–300 cm long) is used. Extra-long guidewires are not used for routine cases because the excess length outside the body is cumbersome and easily contaminated.
Dilators
Vessel dilators are short, tapered, plastic catheters usually made of a stiffer material than diagnostic angiographic catheters ( Fig. 7.5 ). The sole purpose of a dilator is to spread the soft tissues and the blood vessel wall to make introduction of a catheter or device easier. By inserting progressively larger dilators over a guidewire, a percutaneous puncture with an 18-gauge needle can be increased to almost any size. Sequential dilation is important to minimize trauma to the vessel, since incremental steps in size (1F–2F) can be accomplished with much less resistance than one giant step. The initial dilator size after puncture with an 18-gauge access needle should be 5F. Larger dilators can follow as necessary. Dilation of a puncture site beyond 50% of the expected diameter of the artery may obviate manual compression, because the muscular layer of the artery can no longer contract after removal of the catheter.
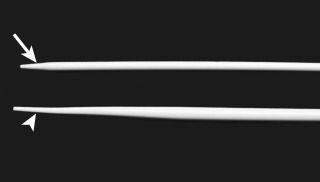
Catheters
Angiographic catheters are usually made of plastic (polyurethane, polyethylene, Teflon, or nylon). The exact catheter material, construction, coatings, inner diameter, outer diameter, length, tip shape, side-hole pattern, and end-hole dimensions are determined by the intended use ( Fig. 7.6 ). Catheters used for nonselective aortography are thick-walled (to handle large-volume high-pressure injections) and often curled at the tip (the “pigtail,” which keeps the end of the catheter away from the vessel wall) with multiple side holes proximal to the curl (so most of the contrast medium exits the catheter in a cloud). Conversely, selective catheters are generally thinner-walled with a single end hole because injection rates are lower and directed into a small vessel. Precise control of the movement of a selective catheter, especially at the tip, is important. These catheters usually have fine metal or plastic strands incorporated into the wall (“braid”) ( Fig. 7.7 ). This results in a catheter tip that is responsive to gentle rotation of the shaft.
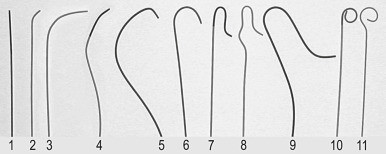
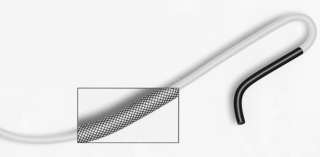
Catheter outer size is described in French gauge (3F = 1 mm), whereas the diameter of the end hole (and therefore the maximum size of the guidewire the catheter will accommodate) is described in hundredths of an inch. The length of the catheter is described in centimeters (usually between 65 and 100 cm). The shape of the tip is named for either something the catheter looks like (“pigtail,” “cobra,” “hockey stick”), the person who designed it (Simmons, Berenstein, Rösch), or the intended use (celiac, left gastric, “headhunter”) (see Fig. 7.6 ) . There are so many different catheters that no one department can or should stock them all. The shape of some catheters (especially Teflon and polyethylene) can be modified by heating the catheter in steam while bending it into the desired configuration. Rapidly dunking the catheter into cool sterile water “sets” the shape.
Complex catheter shapes must be re-formed inside the body after insertion over a guidewire. Any catheter will resume its original shape, provided there is sufficient space within the vessel lumen and memory in the catheter material. Some catheter shapes cannot re-form spontaneously in a blood vessel, particularly the larger recurved designs like the Simmons. There are a number of strategies for re-forming these catheters ( Figs. 7.8, 7.9, 7.10, 7.11, and 7.12 ). These same techniques can be used to create a recurved catheter from a simple angled selective catheter by forming a Waltman loop ( Fig. 7.13 ).
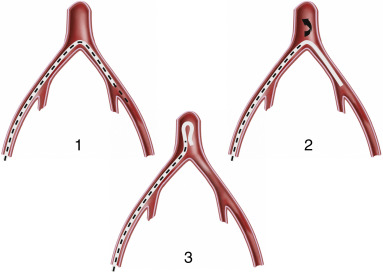
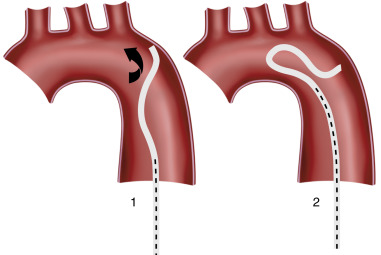
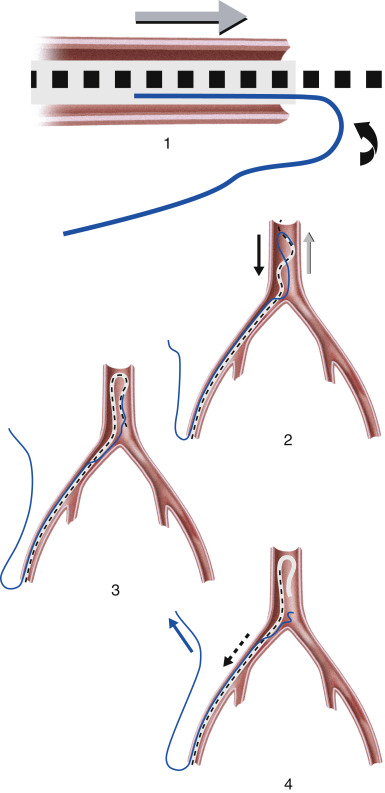
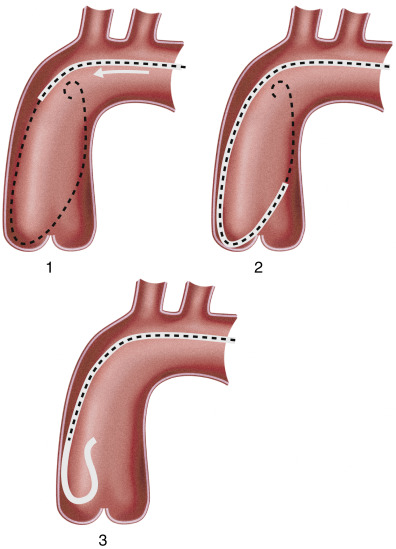
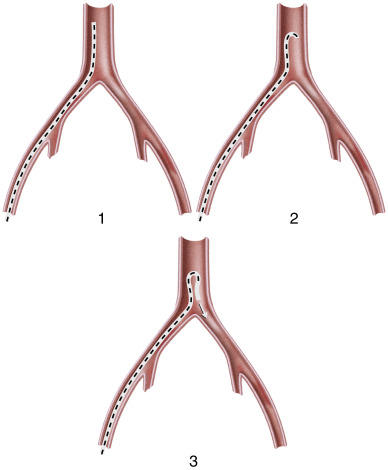
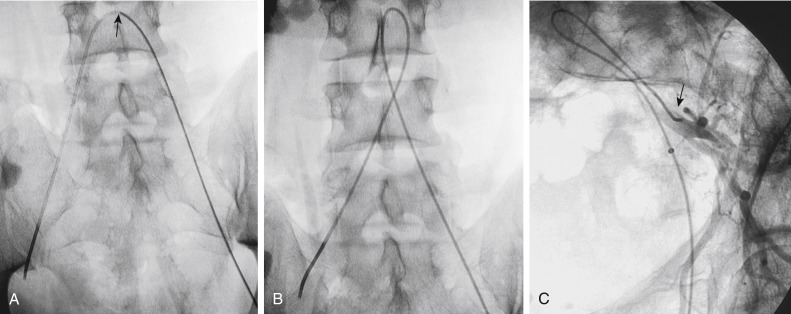
Multiple side-hole straight or pigtail catheters are generally used for nonselective injections. Straight catheters should be advanced over a guidewire, otherwise the tip may cause a dissection or perforation. Pigtail catheters can be safely advanced in normal vessels once the pigtail has re-formed, but should be advanced over a guidewire in abnormal blood vessels.
Selective catheters are chosen based on the particular vessel or anatomy that will be studied ( Fig. 7.14 ). The technique used to catheterize a blood vessel with a selective catheter varies with the type of catheter ( Figs. 7.15 and 7.16 ). The Waltman loop is particularly useful in the pelvis for selecting branches of the internal iliac artery on the same side as the arterial puncture.
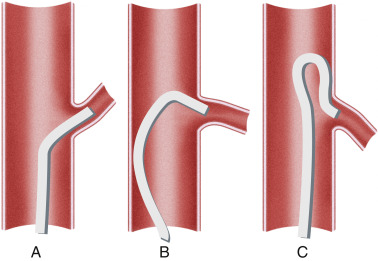
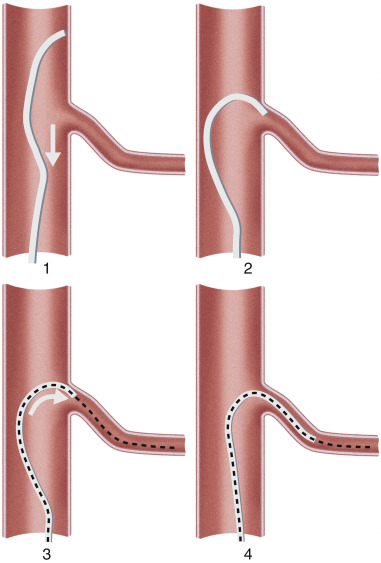
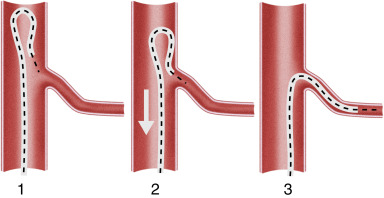
Small catheters (3F or smaller outer diameter) that are specially designed to fit coaxially within the lumen of a standard angiographic catheter are termed microcatheters. These soft, flexible catheters are typically 2F to 3F in diameter, with 0.010- to 0.027-inch inner lumens. They are designed to reach far beyond standard catheters in small or tortuous vessels. These catheters are technologically advanced and have a wide range of characteristics, such as stiffness, braiding, flow rates, and hydrophilic coatings. The ability to reliably catheterize small arteries without creating spasm, dissection, or thrombosis has allowed certain subspecialties (e.g., neurointerventional radiology) to flourish. When using a microcatheter, a standard angiographic catheter that accepts a 0.038- or 0.035-inch guidewire is first placed securely in a proximal position in the blood vessel. The microcatheter is then inserted through the outer catheter and advanced in conjunction with a specially designed 0.010- to 0.025-inch guidewire through the standard catheter lumen. Once a superselective position has been attained with the microcatheter, a variety of procedures can be performed, including embolization, sampling, or low-volume angiography. The small inner lumen and long length result in a high resistance to flow, so microcatheters are not used for routine angiography. Contrast and flush solutions are most easily injected through these catheters with 3 mL or smaller Luer-Lok syringes.
Guiding catheters are another class of catheters designed to make selective catheterization and interventions easier. These catheters can be used in some situations to help position and stabilize standard catheters. Guide catheters are nontapered catheters with extra-large lumens and a simple shape that accepts standard-sized catheters and devices ( Fig. 7.17 ). They are used in circumstances in which standard catheters are difficult to position selectively. The larger outer catheter can provide direction and stability for the inner standard catheter. Guiding catheter size in French gauge refers to the outer diameter. This is the converse of the use of French sizes when describing standard catheters. The inner diameter of the guide catheter is usually described in hundredths of an inch, which must be converted to French gauge to determine which standard catheter will fit (1F = 0.012 inch = 0.333 mm).
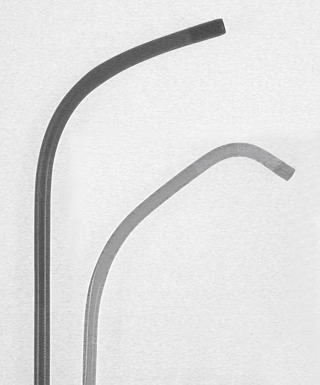
Sheaths
Most vascular interventions and diagnostic procedures are performed through vascular access sheaths. These devices of varying thickness and construction are open at one end and capped with a hemostatic valve at the other ( Fig. 7.18 ). The intravascular end is not tapered, although the edges are carefully beveled to create a smooth transition to the tapered dilator used to introduce the sheath over a guidewire. The valved end usually has a short, clear side-arm that can be connected to a constant flush (to prevent thrombus from forming in the sheath) or an arterial pressure monitor. The purpose of the sheath is to simplify multiple catheter exchanges through a single access site. When not using a sheath, it is unwise to downsize catheters during a procedure (i.e., place a small catheter through a big hole in the artery) because of the risk of bleeding. Devices that are irregular in contour or even nontapered can be introduced through a sheath without fear of trauma to the device or access vessel. In some cases, a long sheath can straighten a tortuous access artery.
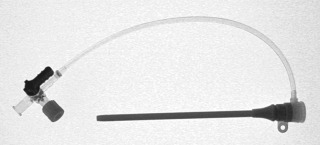
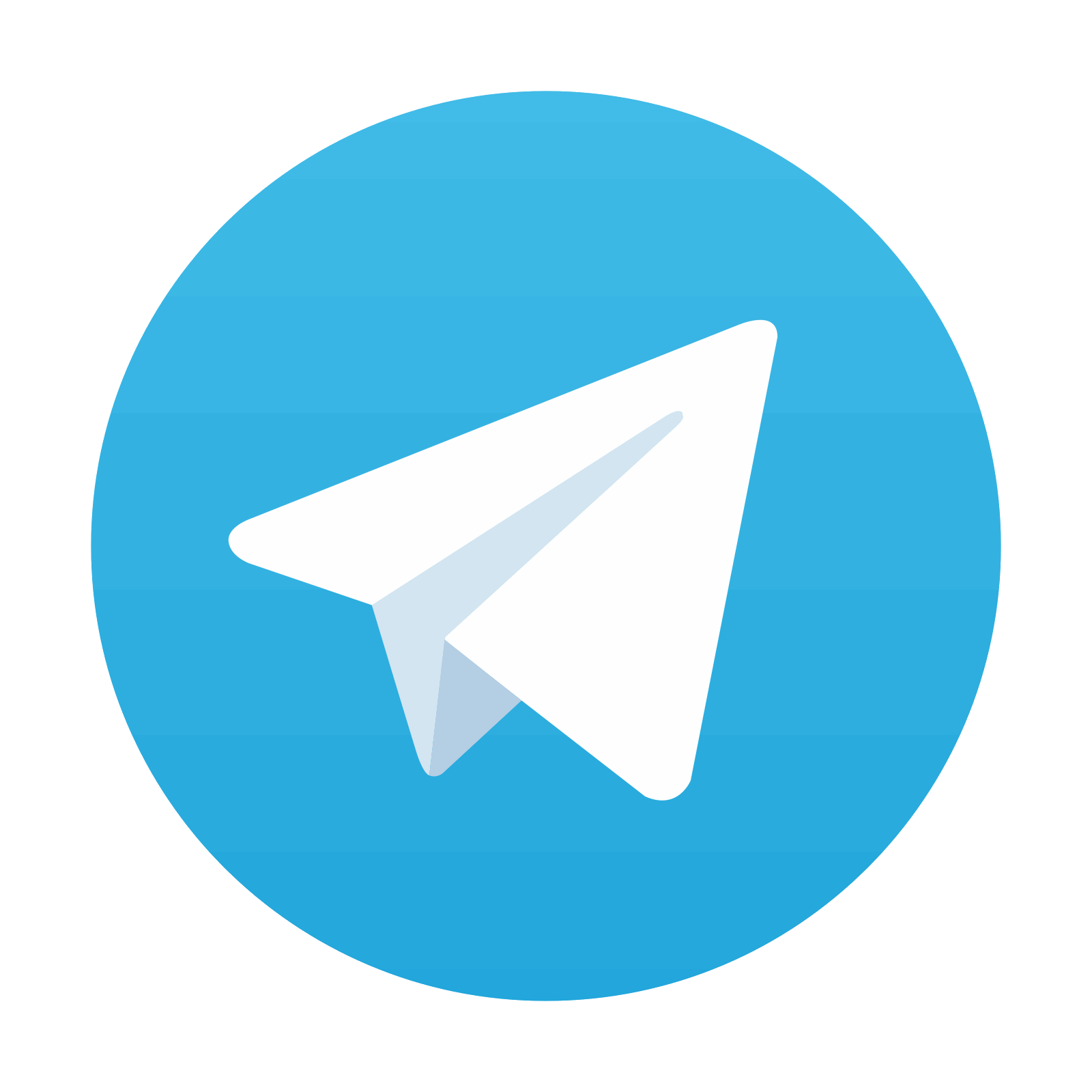
Stay updated, free articles. Join our Telegram channel

Full access? Get Clinical Tree
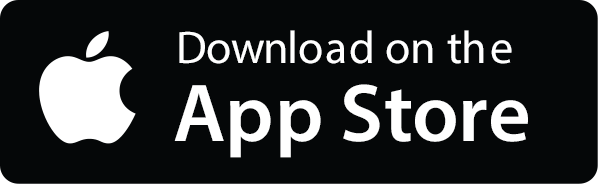
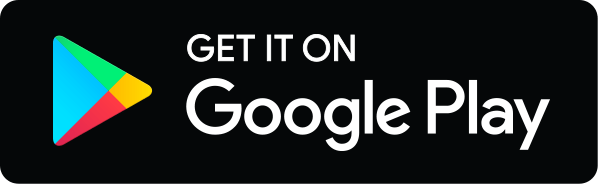
