Sharing the same body cavity, the heart and lungs are closely interconnected by the pulmonary vasculature. An increase or decrease in pulmonary vascular pressures leads to changes in the blood vessels, which directly affect the airways, lung interstitium, alveoli, and pleura. Heart disease often leads to respiratory failure as a result of its impact on gas exchange, water/solute exchange, and pulmonary mechanics. The appearance of lung disease secondary to cardiac disease depends on whether the changes in the pulmonary vascular pressures are acute or chronic. Clinical manifestations of cardiac disease include pulmonary edema, pleural effusion, hypoxemia, pulmonary hypertension, atelectasis, and plastic bronchitis.
Noninfectious pulmonary complications, such as bronchiectasis, prolonged postoperative mechanical ventilation, extubation failure, airway complications (e.g., tracheobronchomalacia, subglottic stenosis, bronchial stenosis), and obstructive sleep apnea, have been extensively described in pediatric patients after cardiac surgery. Although many of the physiologic mechanisms are similar, this chapter concentrates on pediatric heart diseases and disorders that have an impact on the pulmonary system.
Overview of the Cardiovascular Circulation
The cardiopulmonary vascular system comprises two components: the pulmonary circulation and the bronchial circulation. The bronchial circulation constitutes a very small portion of the output of the left ventricle, and it supplies part of the tracheobronchial tree with systemic arterial blood. The pulmonary circulation constitutes the entire output of the right ventricle, and it supplies the lung with the mixed venous blood draining from all the tissues of the body. This blood undergoes gas exchange with alveolar air in the pulmonary capillaries. Under normal circumstances, these systems change with the maturational stage of the individual. It is important to understand the vascular changes that occur with normal growth over time. A brief review of the cardiovascular circulation follows.
Pulmonary Circulation
The normal mature pulmonary circulation is a low-resistance system compared with the general systemic circulation. Despite receiving about the same amount of blood flow, the pulmonary arterial pressure is only about 20% of the systemic circulation pressure. The plasticity of the pulmonary circulation can be attributed to two related factors: (1) the pulmonary vessels are thin-walled and dilate with mild increases in pressure, and (2) this augmentation of the vessel radius passively recruits underperfused vessels, which leads to an increase in overall cross-sectional area.
In contrast to the mature pulmonary circulation, the fetal pulmonary resistance is higher than the systemic resistance. The high pulmonary system resistance in the fetus permits shunting of blood from the systemic venous to the systemic arterial circulation via the ductus arteriosus to the aorta and through the foramen ovale to the left atrium. The following three factors contribute to the high fetal pulmonary circulation resistance: (1) In the fetus, the pulmonary arteries are exposed to the full systemic blood pressure via the ductus arteriosus, and their walls are very muscular. (2) The fetal lung exists in an airless state. (3) There is hypoxic vasoconstriction within the intrauterine environment.
With the onset of air breathing and inflation of the lungs, the pulmonary circulatory resistance decreases with the loss of the placental circulation. The inflation of the lungs causes expansion of the pulmonary vessels, and the increase in oxygenation results in vasodilation. With subsequent involution of the arterial muscle, there is remodeling of the pulmonary arteries. This remodeling involves thinning of the arterial muscular wall and the normal branching and growth of the airways and alveoli. Although the pulmonary veins have thinner walls compared with the arteries, veins do have a muscular layer, which can become hypertrophied in response to elevated pulmonary pressure.
Bronchial Circulation
In contrast to the pulmonary circulation, the bronchial circulation is small, carrying only 1% of the cardiac output. The bronchial arteries carry oxygenated blood to the lungs as part of the general systemic circulatory system. Bronchial arteries respond to stimuli, as do the other systemic arteries (dilation in response to hypoxia). Conversely, the pulmonary arteries constrict to hypoxia. The bronchial arteries arise from the aorta and the intercostal arteries, and then divide along with the bronchial divisions. Despite the differences between the pulmonary and bronchial circulations, there are intricate interconnections between them. Anastomotic vessels connect the bronchial arteries to the pulmonary arterioles and to the pulmonary veins. These anastomotic connections permit flexibility of flow to and from pulmonary and bronchial circulations. The drainage of the bronchial arteries to a vascular plexus permits great flexibility of bronchial venous drainage. The bronchial venous drainage can move to either the right or the left side of the heart. Extrapulmonary bronchial vessels supplying large airways drain to the right atrium through the azygos and hemiazygos veins. The intrapulmonary vessels drain to the pulmonary veins and to the left atrium. The venous drainage permits flexibility in drainage route, depending on changing hemodynamic pressures.
Lymphatic Circulation
The pulmonary lymphatic circulation lies in the connective tissue of the lung. There is continuous filtration of liquid and protein through the lung microcirculation. Passive flow of liquids proceeds along a pressure gradient to reach the lymphatic capillaries and is returned via active pumping to the systemic circulation. Disruption of the intravascular pulmonary pressures also alters the lymphatic circulation.
Cardiovascular Lesions That Increase the Work of Breathing
Generally, three types of cardiovascular problems cause disturbances in mechanical function of the lungs and increase the work of breathing: (1) large volume left-to-right shunts, (2) outflow or inflow obstruction of the systemic ventricle, and (3) vascular anomalies that obstruct the airways.
Large Volume Left-to-Right Shunts
Lesions that allow communication between the systemic and pulmonary circulations and cause a large left-to-right shunt are the most frequent congenital cardiac anomalies. These include common problems such as ventricular septal defect (VSD), atrial septal defect (ASD), and patent ductus arteriosus (PDA), and defects with similar physiologic consequences, but more complex anatomy, such as single ventricle, aorticopulmonary window, and truncus arteriosus. These lesions are characterized by the recirculation of oxygenated blood through the lungs and pulmonary vascular congestion. The shunt produces excessive pulmonary blood flow and increased return of pulmonary venous blood to the left side of the heart. In addition, when the abnormal communication is large and occurs at the level of the ventricles or great vessels, the high pressure of the left side of the heart is transmitted to the pulmonary circulation, causing pulmonary artery hypertension.
The volume of blood traversing the pulmonary circulation in the presence of an anomalous intracardiac communication or between the great arteries depends on the size of the communication and the relative resistances of the pulmonary and systemic circulations. When the communication is small, it offers a high resistance to the passage of blood. As a result there is a large pressure decrease between the high-pressure chamber and vessel (usually, the left ventricle or aorta) and the low-pressure chamber or vessel (usually, the right ventricle or pulmonary artery), and the left-to-right shunt is limited. When the communication is large, there is virtually no resistance to the flow of blood from the systemic to the pulmonary side of the circulation. Consequently, the pressures become equal in both sides, and the size of the shunt is no longer governed by the size of the communication, but rather by the relative magnitudes of the systemic and pulmonary vascular resistances. Because the pulmonary vascular resistance of the term newborn is very high, it is not surprising that even large communications such as those caused by a large VSD or PDA produce little left-to-right shunt for days, or even weeks, after birth. As pulmonary vascular resistance declines postnatally, signs of pulmonary congestion develop quickly.
Numerous factors have been identified that influence the decrease in pulmonary vascular resistance associated with the transition to extrauterine life. In the immediate postnatal period, the expansion of the alveoli, the increase in alveolar PO 2 , and the decrease in the alveolar PCO 2 cause recruitment of pulmonary capillaries and dilation of pulmonary arterioles, decreasing pulmonary vascular resistance. As the arterial musculature becomes thinner, and new vessels gradually grow in the lungs, pulmonary vascular resistance decreases even further. Finally, the postnatal decline in hematocrit—so-called physiologic anemia—also contributes significantly to the decrease in pulmonary vascular resistance by reducing blood viscosity.
Left-to-right shunts can cause increased symptoms after the decrease in the pulmonary arterial pressure and pulmonary vascular resistance that normally occurs after birth. As mentioned before, these shunts can be intracardiac (ASD, VSD, or atrioventricular septal defect) or extracardiac (PDA or aorticopulmonary window). Intracardiac shunts usually are associated with increased pulmonary blood flow without an increase in pressure in the early stage of the disease. Extracardiac shunts (depending on their size) may expose the pulmonary vascular circulation to systemic pressures that would cause earlier development of vascular changes. Shunt lesions, such as a VSD, ASD, or PDA, may not cause a significant murmur in a neonate because of the normally elevated pulmonary vascular resistance at birth causing the shunting to be low velocity. As the pulmonary vascular resistance decreases to normal levels over the first 1 to 2 months of life, the shunt becomes more prominent. The normal systemic vascular resistance is about 25 Wood units/m , and normal pulmonary vascular resistance is about 3 Wood units/m , so blood shunts from the left (systemic) circulation to the right (pulmonary) circulation.
Pathophysiology of Pulmonary Edema and Pleural Effusion
One of the main pathophysiologic effects of the left-to-right shunt is to redistribute part of the left ventricular output from the systemic to the pulmonary circulation ( Fig. 4-1 ). When this occurs, pulmonary blood flow increases, increasing the right ventricular afterload, left ventricular preload, left atrial pressure, and the work performed by the heart muscle. In normal circumstances, the venous pressure in the lung is low and varies only a little during the cardiac cycle. An increase in left ventricular end-diastolic or left atrial pressure can cause increased pressure in the pulmonary veins, however, with subsequent pressure increases in the capillary vessels and then in the pulmonary arteries. As a result, fluid starts to accumulate in the interstitium and alveoli, causing pulmonary edema. Edema usually forms in response to increased pulmonary venous pressures. As the intravascular pressure increases, more extravascular fluid is filtered through the pulmonary interstitium to the lymphatic system. When this microvasculature filtration system becomes overwhelmed, there is accumulation of fluid in the interstitial lung tissue. An early feature of this fluid buildup, or edema, is the accumulation of fluid around the bronchovascular bundles. This accrual of bronchovascular bundle fluid can be seen on chest radiographs, particularly in the fissures.
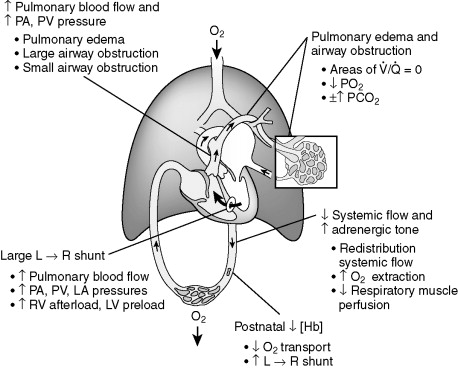
V . / Q . = 0
), and can depress arterial P o 2 and increase arterial P co 2 . Because of the reduced systemic perfusion and increased adrenergic tone, systemic blood flow is redistributed, O 2 extraction increases, and respiratory muscle perfusion is diminished. The postnatal decline in hemoglobin concentration [Hb] further aggravates the circulatory imbalance by decreasing O 2 transport and increasing further the left-to-right shunt.
When the pulmonary lymphatic system cannot clear the interstitial fluid, the fluid accumulation must leave by other means. One route is to form a transudate through the interlobular septa and then to the pleural space, which leads to pleural effusion. When in the pleural space, the liquid is absorbed into the parietal pleural lymphatic system. Transport of interstitial fluid to the pleural space reduces the possibility of alveolar edema, which is associated with altered gas exchange and pulmonary mechanics. In contrast, pleural effusion alone generally is not associated with severe derangements in pulmonary function. Alterations in blood flow and in pulmonary vascular pressures are associated with pathologic changes in the pulmonary arteries ( Table 4-1 ).
Aorta |
---|
Patent ductus arteriosus |
Aorticopulmonary window |
Truncus arteriosus |
Postsurgical shunts |
Blalock-Taussig (subclavian artery to ipsilateral pulmonary artery) |
Potts (descending aorta–left pulmonary artery) |
Waterston (ascending aorta–right pulmonary artery) |
Atrium |
Atrial septal defect |
Common atrium |
Total or partial anomalous pulmonary venous connection |
Transposition of the great arteries with atrial septal defect |
Ventricle |
Ventricular septal defect |
Single ventricle |
Atrioventricular canal |
Associated lesions with ventricular septal defect |
Transposition of the great arteries |
Double-outlet right ventricle |
Tricuspid atresia without pulmonary stenosis |
Mitral atresia |
The earliest change to increased pressure in the pulmonary arteries is medial thickening, which is the response to increased intramural vascular pressure. The medial thickening may come about from hypertrophy and hyperplasia of smooth muscle cells. The next change is an increase in intimal thickening. Intimal thickening can be due to an increase in the number of cells or an accumulation of dense fibrous tissue. The development of irreversible change is heralded by the formation of plexiform lesions ( Fig. 4-2 ).
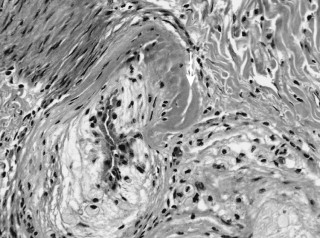
As the pulmonary vascular disease gradually worsens from the increased pressure, the pulmonary vascular resistance also increases and results in decreased pulmonary blood flow. When the pulmonary vascular resistance becomes higher than the systemic vascular resistance, the shunt flow (initially left-to-right shunt) reverses direction, leading to a right-to-left shunt. At this point, the elevated pulmonary vascular resistance with a right-to-left shunt is defined as Eisenmenger syndrome.
Clinical Findings of Acute Increased Pulmonary Venous Pressure
Patients with acute pulmonary edema may be asymptomatic in the early stages. On auscultation, the lung sounds can be normal, or there may be mild wheezing. As the condition progresses with alveolar edema, the patient may experience tachypnea and possible cough productive of foamy secretions. At this stage, coarse crackles (rales) are usually heard. Conditions associated with acute increase of pulmonary venous pressure are outlined in Table 4-2 .
Aortic regurgitation |
Left atrium |
|
Left ventricular failure |
|
The chest radiograph is more sensitive than the history or physical examination in detecting acute elevation of pulmonary venous pressure ( Fig. 4-3 ). The chest radiograph shows increased vascular markings and changes in the cardiac silhouette, depending on the underlying heart disease. Kerley B lines are seen in the periphery of the lungs, indicating accumulation of fluid in the interlobular septa. Alveolar edema is represented radiologically as fluffy infiltrates. Pleural effusion may also appear, usually bilaterally beginning with blunting of the costophrenic angles ( Fig. 4-3 ).
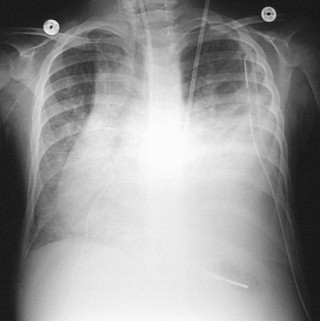
Even with interstitial edema and pleural effusion, pulmonary function changes little in patients with acute increased pulmonary venous pressure. Alveolar edema is associated with significant decreases in the PaO 2 , however. With increased alveolar flooding, there is also a decrease in lung volume and compliance. Airway resistance increases because of the decrease in lung volume and liquid filling the airway lumen.
Pulmonary edema renders the alveoli unstable, and it makes the lung stiff, increasing the work that the respiratory muscle must perform to maintain adequate ventilation. Pulmonary edema is not the only mechanism, however, by which the work of breathing becomes greater in subjects with a left-to-right shunt. These patients can also develop extrinsic airway compression. Pulmonary “overcirculation” along with pulmonary hypertension may cause extrinsic compression of the main and lobar bronchi by enlarged pulmonary arteries (usually affecting the right main stem bronchus, the lingular and left upper lobe bronchi, or the left main stem bronchus) or by distention of the left atrium (affecting the left lower lobe bronchus). In addition, there is usually compression of small intraparenchymal airways by engorged peribronchial vessels or by bronchial wall or peribronchial edema.
The term “cardiac asthma” is often applied to describe the wheezing caused by compression of large and small intrathoracic airways. Affected infants often may have respiratory symptoms so prominent that they overshadow or mask the underlying cardiac disease. The shunt may be recognized only when a clinician notes the coexistence of cardiomegaly and air trapping on a chest radiograph. Bronchial compression at these locations also can produce lobar emphysema or atelectasis. This complication is seen most frequently in 2 to 9-month-old infants. The age range predilection may be linked to the gradual decrease of pulmonary vascular resistance over the first few months of life that results in an increase in pulmonary blood flow and the small airway caliber and less cartilaginous airway support in infants. Evaluation for underlying cardiac disease also should be part of the assessment of an infant or young child with atelectasis or lobar emphysema.
Pulmonary edema, and the mechanical disturbances that it produces, also impairs gas exchange within the lung. In the presence of a left-to-right shunt, overall ventilation to perfusion is low, and there are areas that are perfused but not ventilated (true right-to-left intrapulmonary shunt). However, the recirculation of arterial blood through the lung and the high saturation of pulmonary arterial blood (owing to the left-to-right shunt) minimize the effects, of intrapulmonary right-to-left shunting on arterial oxygen saturation. Arterial PO 2 and PCO 2 are usually near-normal, unless there is respiratory fatigue caused by increased respiratory rate and effort.
Patients with ASD are usually asymptomatic in early life, although they may have an increased incidence of respiratory tract infections. With the onset of pulmonary hypertension (usually in adulthood), dyspnea and fatigue may occur. Patients with left-to-right shunts at the aortic and ventricular levels may develop biventricular congestive heart failure in infancy. Symptoms include dyspnea, grunting, apnea, and poor feeding. The early congestive heart failure also predisposes these infants to respiratory infections and poor growth. When the disease has progressed to Eisenmenger syndrome, all patients have cyanosis (at rest and during exercise) and dyspnea. The clubbing that is apparent is due to chronic hypoxia; the peripheral tissue capillaries dilate to increase the oxygen supply, causing digital swelling. Other symptoms that occur after disease progression to Eisenmenger syndrome are chest pain, syncope, and hemoptysis. The hemoptysis can be caused by pulmonary infarction from low pulmonary blood flow or congestion, polycythemia, or bronchial arterial bleeding.
Patients with PDA characteristically have a continuous murmur. When the disease progresses to Eisenmenger syndrome, a patient with an uncorrected PDA has differential cyanosis (upper body is pink and lower body is blue owing to right-to-left shunt beyond the left subclavian artery). Isolated digital clubbing of the toes without clubbing of the fingers may occur because of the difference in upper versus lower body oxygenation.
Treatment of Large Volume Left-to-Right Shunts
Although corrective or palliative surgery is the ultimate therapy for each of the conditions described in this section, initial medical management is essential for stabilizing an infant with critical congestive heart failure. For an infant with large left-to-right shunt, treatment traditionally has focused on improving myocardial function, removing excess fluid accumulated in the lungs, and, in some patients, providing assisted respiration. These infants have increased caloric needs, and close assessment of growth is an essential part of their management and part of the decision-making process in determining the timing of corrective surgery. They have increased energy requirements and sometimes have difficulty feeding secondary to shortness of breath.
In an acutely decompensated infant, an intravenous inotropic agent, such as dopamine, dobutamine, or isoproterenol, is most useful. For long-term use, a medication that can be given enterally, such as digoxin, is more appropriate. Diuretics can improve respiratory function by controlling the accumulation of pulmonary edema. In particular, the combination of diuretics and inotropic drugs can decrease filling pressure in the left atrium and reduce pulmonary microvascular pressure, attenuating edema formation. Depending on the specific lesion and the size of the patient, often interventional cardiac catheterization offers an alternative to cardiac surgery. These less invasive techniques are currently widely used for the closure of secundum ASDs and PDAs.
Clinical Manifestations of Chronic Increased Pulmonary Venous Pressure
Several conditions are associated with chronic increased pulmonary venous pressure ( Table 4-3 ). The gradual onset of increased pulmonary venous pressure leads to an almost imperceptible onset of symptoms. These early symptoms may include dyspnea, fatigue, and decrease in endurance or exercise tolerance. When these patients are otherwise stable, symptoms may be minimal. Acute increases in cardiac output (e.g., fever or exercise) or decreases in filling time (e.g., tachyarrhythmias) can lead to sudden worsening of dyspnea ( Fig. 4-4 ). Hemoptysis is a late complication and is caused by rupture of the dilated bronchial anastomotic vessels.
