Pulmonary Perfusion Imaging with CT
Sonja Sudarski, MD
Thomas Henzler, MD
▪ Introduction
Multiple approaches to assess pulmonary perfusion by means of CT have emerged over the past decades. In the year 2000, Schoepf et al.1 used dynamic multisection electron beam CT for the assessment of pulmonary blood flow (BF). However, the technique was limited to a z-axis coverage of 7.6 cm and required a long patient breath hold. Moreover, the technique used in this feasibility study was associated with a substantial amount of ionizing radiation limiting its clinical acceptance. Major basic research on the feasibility of multidetector CT pulmonary perfusion imaging has been performed by the group of Wildberger2,3,4 and 5: in 2001, perfusion-derived color maps of the density of lung parenchyma in single-energy CT pulmonary angiography (CT-PA) of patients with suspected pulmonary embolism (PE) were generated.3 A later animal study by the same group used a subtraction technique to compare density values of whole-thorax multidetector CT scans before and after intravenous contrast injection in a single breath hold; however, this technique was again limited by the breath-hold length and the additional radiation dose the patient was exposed to.2 Moreover, one has to acknowledge that the term perfusion was misleading in these studies since only one contrast phase during the first pass of contrast material was imaged by CT. Thus, the term first-pass perfusion seems to be more appropriate also within the context of today’s dual-energy CT (DE-CT).
To date, the probably most important step towards the broad introduction of first-pass pulmonary perfusion imaging into clinical routine was the advent of DE-CT: the principle of DE-CT was initially introduced in the late 1970s and allows for the differentiation of materials based on their x-ray attenuation at different tube voltages. DE-CT has been investigated for a variety of organ systems. At that time point, with regard to thoracic imaging, limited temporal resolution and respiratory misregistration were the main reasons wherefore DE-CT did not enter clinical routine at that time. Different vendors reintroduced DE-CT in the second half of 2000 using different technical approaches like the dual-source CT (DS-CT) technology, single-source rapid kV switching, or a dual-layer approach to separate different x-ray spectra.6,7,8 and 9
Since then, pulmonary DE-CT imaging has been intensively studied10,11,12,13,14 and 15: DE-derived iodine maps allow the visualization of parenchymal iodine distribution, which can be considered as a surrogate for pulmonary first-pass perfusion.
Ventilation and Perfusion of the Lung
To properly interpret perfusion imaging, the interaction of ventilation and perfusion has to be considered in the first place: In the presence of hypoxia without presence of hypercapnia, blood flow will be directed to lung segments and alveoli with a higher oxygen concentration. Therefore, pulmonary arteries will constrict in the presence of low oxygen blood levels. This physiologic phenomenon of hypoxic pulmonary vasoconstriction is generally known as the Euler-Liljestrand mechanism.16 Although the process might seem paradoxical at first impression, the redistribution of blood flow to higher-ventilated areas of the lung increases the area involved in gaseous exchange and consequently improves the ventilation-perfusion ratio and arterial oxygenation as a last consequence. This compensation mechanism can be observed in patients with chronic obstructive pulmonary disease and heart failure or as well in healthy individuals at altitude.
Due to patient’s supine position during a CT scan, lung perfusion images have to be seen in the light of an abolished craniocaudal perfusion gradient in an upright thorax and a gravity-dependent gradient in ventrodorsal direction instead.
Furthermore, morphologic alterations of small pulmonary vessels have to be considered, as larger cross-sectional areas have been shown to influence pulmonary perfusion.17
DE-CT-PA is consequently able to provide both morphologic and functional information for an accurate assessment of lung physiology, that is, vascular morphology, regional perfusion, and ventilation.
DE-CT Techniques
Various approaches for acquiring multiple energy data of an anatomic area of interest have been developed by different vendors. Dual-source technique, single-source rapid kV switching technique, and dual-layer technique are the most common and promising ones with regard to pulmonary imaging: while DS-CT and double-layer technology derive their information from one set of data acquired at identical time points, in fast kilovoltage-switching technique, there is a delay of a few milliseconds between the acquisitions. In DS-DE-CT, two x-ray tubes and two corresponding detectors at different kilovoltages and tube currents are simultaneously run.6 The single-source CT-derived double-layer detectors conduct compartmentalization of detected x-ray photons into energy bins.18
In another single-source DE-CT approach, the rapid kV switching technique, the tube voltage alternates between a high voltage and a low voltage, and for every projection, data are acquired twice. The rotation speed has to be therefore reduced in order to account for the acquisition of these additional projections and the rise and fall times of the voltage modulation. Therefore, the gantry rotation time usually must be at least 0.5 seconds.9,18
Most evidence on DE-CT pulmonary perfusion imaging was gained with the DS-CT technique so far. The DS-DE-CT technique allows differentiation, separation, and quantification of tissue or material with high atomic numbers such as iodine and xenon. The two x-ray tubes and two corresponding detectors are positioned 90 degrees apart from each other in the gantry. Initially, a combination of 80/140 kVp was used for dual-energy scanning. Then, 100 kVp was suggested as an alternative to 80 kVp for dual-energy scanning of thicker body regions and/or larger adults in order to improve image quality by means of noise reduction. Because of the low-attenuation profile of the thoracic region, using 80 kVp is usually sufficient for DE-CT of the chest. In order to compensate for the lower photon output of the low-kilovoltage tube, the reference tube current has to be increased. Nevertheless, this technical approach has been proven to be the best with regard to radiation dose efficiency. In second-generation DS-CT scanner systems, a better separation of x-ray spectra could be achieved by application of a tin filter to the 140-kVp tube, resulting in less photon starvation of low-spectrum photons within the patient and consequently in further noise reduction and dose savings. In third-generation DS-CT scanner systems, both tubes are equipped with tin filters, although in DE-CT mode, only the high-kVp tube is run with the tin filter, at this case with 150 kVp. Three-material decomposition is used for both perfusion and ventilation CT, in which iodine or xenon, respectively, is decomposed from body tissue and air by calculating volume fractions of the three materials for each voxel.
DE-CT thereby is able to provide both anatomic and functional information in a variety of pulmonary diseases by means a contrastenhanced chest scan using only a single contrast material application (Fig. 26-1): furthermore, in the course of image postprocessing, virtual nonenhanced DE-CT-derived images can be created as well, which are then used to characterize pulmonary nodules, which abolishes the need to acquire additional true nonenhanced CT data.
Principle of Dynamic Contrast-enhanced CT
Besides DE-CT-derived iodine maps, with the advent of newer scanner generations and continuously lower radiation doses required for CT examinations, 4-D time-resolved dynamic contrast-enhanced CT (DCE-CT) has been proposed as a new single-energy method to visualize pulmonary perfusion. However, due to additional radiation exposure, DCE-CT has been mainly investigated for different tumor entities in order to quantify tumor perfusion over time so far19,20,21,22,23,24,25,26,27,28,29 and 30: tumor angiogenesis leads to an increase of regional blood flow and blood volume. Newly developed antiangiogenic therapies—so-called targeted therapies—are aiming at activation of molecular antiangiogenic pathways with the overall goal to reduce tumor nourishment. Consequently, imaging modalities used in the diagnostic workup of such patients for the purpose of staging, restaging, and followup examinations have to keep up with the innovations in oncology: with the acquisition of CT angiographic data of multiple repetitive CT scans with only one bolus of iodinated contrast material given and subsequent summation of the gathered information resulting in time-density curves, functional information is added to mere morphometric information. Assessment of contrast enhancement of lung cancer tissue, tumor perfusion, and perfusion changes in the course of therapy by means of dynamic perfusion computed tomography (dPCT) in patients with lung cancer therefore seems to be a promising new approach with regard to evaluation of response to therapy.31,32 Until recently, the biggest drawback of dPCT was the fact that time-density curves could only be acquired for selected transverse sections within the tumors but not for the whole tumor volume, limiting its representative value for response to therapy, especially in large tumor masses.33 Reproducibility of dPCT values was further reduced due to tumor motion during the breathing cycle and differing transverse tumor sections evaluated in different intraindividual examinations as a possible consequence.34 These limitations have been overcome by dPCT of whole tumor volumes with sufficient motion correction, subsequently referred to as dynamic volume perfusion CT (dVPCT).35,36 and 37 DCE-CT-derived dPCT or dVPCT is thereby able to provide quantitative data of blood flow, blood volume, permeability, and the mean transit time of iodinated contrast material through the whole tumor.38,39,40,41,42,43 and 44
Despite robust results regarding visualization of vasculature and promising results regarding visualization of tissue perfusion, to date, the technique of dVPCT is not routinely used, as ideal time points of perfusion imaging are not yet standardized and multiple shuttle protocols are existing with varying scan time points from every 1.5 seconds up to every 6 seconds with introduction of thirdgeneration DS-CT. This chapter will focus on the DE-CT technique, as indications are broader and more evidence as well as established and robust protocols do already exist.
▪ Clinical Applications
Pulmonary perfusion imaging plays an important role in the diagnostic workup of PE, thoracic malignancies, vascular disorders, and parenchymal diseases.8,45
Pulmonary Embolism
Acute PE
CT-PA is the reference standard for the diagnosis of acute PE and has replaced catheter pulmonary angiography and ventilation-perfusion scintigraphy as the first-line imaging method.46,47 Suspected pulmonary embolism is the most common indication of lung perfusion by means of DE-CT in both children and adults.15,48,49,50,51 and 52 Aside from visualizing pulmonary blood pool deficits for diagnosing PE, DE-CT can be used for monitoring success of PE treatment.53
Chronic PE
DE-CT-PA can also allow diagnosis of chronic PE and chronic thromboembolic pulmonary hypertension. DE-CT has also been found to aid in the differentiation of ground-glass attenuation of vascular origin in chronic PE patients (by means of the high iodine content within the areas of ground-glass attenuation) from ground-glass attenuation secondary to bronchioloalveolar diseases.54 Also, image series generated from lower x-ray energies have been reported to be useful for an improved visualization of the systemic collateral supply in chronic PE, originating from bronchial and nonbronchial (i.e., chest wall, abdomen) systemic arteries.55
Emphysema
Chronic obstructive pulmonary disease is defined as airway obstruction due to irreversible parenchymal destruction. Assessment of emphysema severity has gained increasing importance with regard to treatment planning for lung volume reduction surgery. DE-CT can be performed for emphysema quantification and regional perfusion evaluation using virtual unenhanced series and iodine maps simultaneously.56
Functional Resectability
DE-CT pulmonary perfusion imaging can also be used to determine functional resectability not only in patients with emphysema but also in patients with malignancies prior to surgical lobectomy. Preoperative exact quantification of FEV1 to calculate postsurgical FEV1 is necessary in these patients. DE-CT can help to determine the exact percentage a distinct segment owns in overall ventilation.57,58 and 59
Lung Cancer
The feasibility of DCE-CT to characterize pulmonary nodules has been proven by a prospective, multicenter trial evaluating DCE-CT for the classification of benign and malignant pulmonary nodules.60 In solitary pulmonary nodules (SPNs) with a diameter greater than 10 mm, CT perfusion could significantly reduce the number of SPNs, diagnosed as undetermined at morphologic CT, thereby skipping the need for follow-up CT examinations, additional FDGPET studies, biopsy, or even surgery.61
DCE-CT may be furthermore used for the assessment of tumor response to targeted therapy or radiotherapy. Good intraobserver agreement has been reported for lung cancer DCE-CT measurements, encouraging its use for tumor characterization and therapy response monitoring.62 Moreover, whole tumor DCE-CT may allow advanced subclassification of different lung cancer entities.35
Excursion: Angioinvasive Aspergillosis
Another rare indication for DCE-CT is diagnosis of suspected angioinvasive aspergillosis as a severe and life-threatening complication in immunosuppressed patients (Fig. 26-2). DCE-CT can help to accurately identify vessel interruption caused by angioinvasive aspergillosis that has been reported as a relatively specific sign of disease.63
▪ DE-CT Protocols
Adults
Various image acquisition protocols for DS-DE-CT image acquisition have been proposed in the literature; however, currently, only few protocols are available for single-source DE-CT techniques.8 The scanning protocols aim at evaluating both the pulmonary arteries and lung perfusion from a single contrast-enhanced CT examination.64 To evaluate both the pulmonary arteries and lung perfusion, the scanning delay should be slightly longer (e.g., 4 to 7 seconds) than that for regular CT-PA examinations to allow the contrast material to distribute in the lung parenchyma.8,64 Dualenergy lung perfusion CT should be obtained at peak lung parenchymal enhancement in order to avoid underestimation of regional lung perfusion.8
With regard to this, it should be always kept in mind that, in contrast to DCE-CT, the DE-CT-PA-derived perfusion map merely reflects iodine distribution in the lung parenchyma at a given single time point.
Thoracic DE-CT scans should be acquired in the caudocranial direction so that the saline chaser bolus reaches the upper chest by the time this area is acquired to avoid streak artifacts from highly concentrated contrast material in the subclavian vein or superior vena cava.64,65 Patients should be instructed to hold their breath at a shallow inspiratory level during scan acquisition to avoid excessive influx of unenhanced blood from the inferior vena cava from the Valsalva maneuver associated with deep inspiration.66
With regard to contrast material doses, high iodine concentration and high iodine delivery rate have been shown to provide the best image quality of both CT-PA and perfusion map images of the lung through high attenuation within the pulmonary arteries and minimization of beam-hardening artifacts.
Nance et al.67 reported that a contrast material protocol using a high iodine concentration and a high injection delivery rate for contrast material delivery (iomeprol 400 at 4 mL/s, corresponding to an injection delivery rate of 1.6 g I/s) resulted in the best image quality of both CT-PA images and perfusion map images of the lung by virtue of high attenuation in the pulmonary arteries and minimization of beam-hardening artifacts compared with protocols comprising a lower concentration or lower delivery rate. Despite controversial reports about increased artifacts on iodine perfusion maps when using highly concentrated iodinated contrast material combined with high flow rates, consensus exists about improved diagnostic image quality and the highest target-tract attenuation with these DE-CT-PA protocols.68
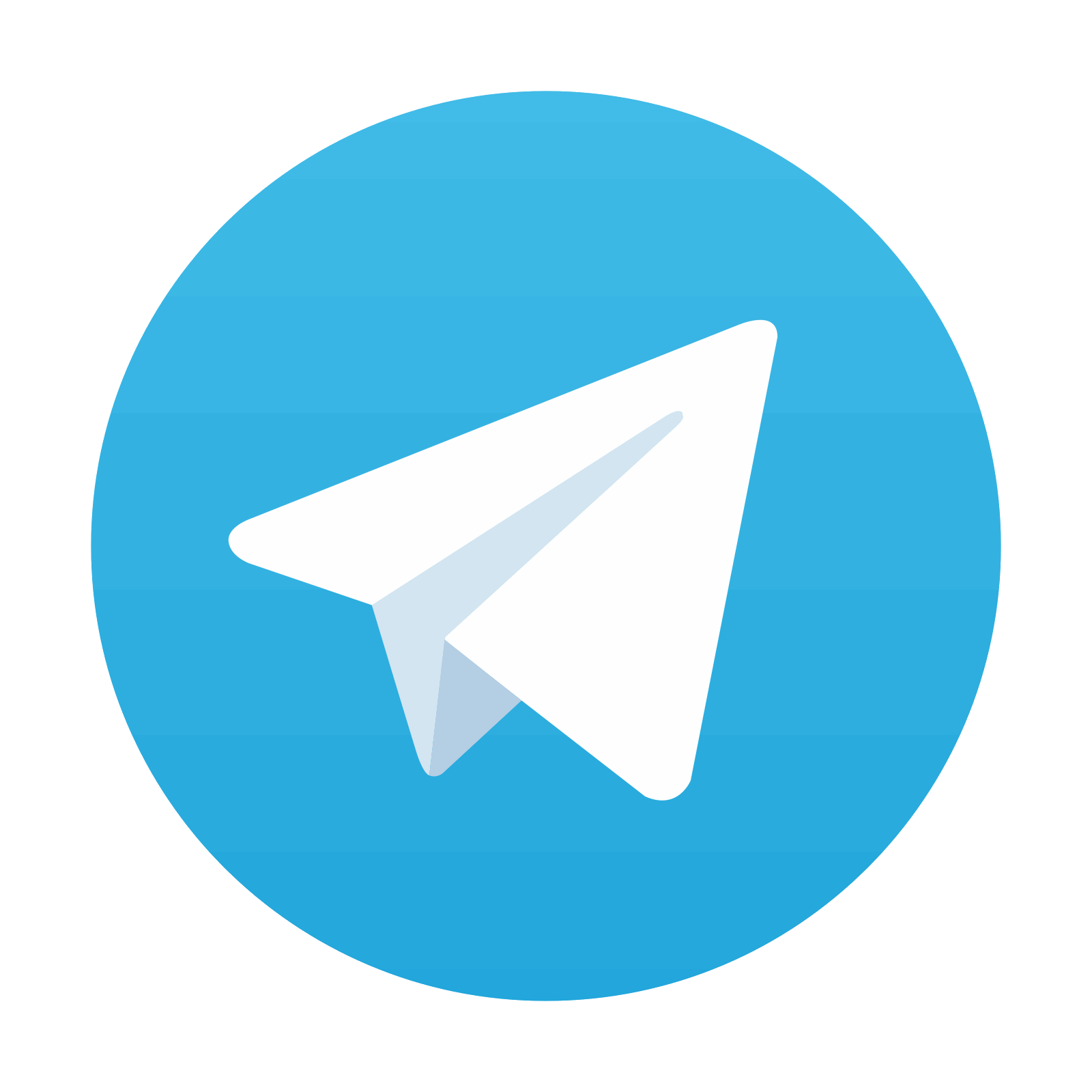
Stay updated, free articles. Join our Telegram channel

Full access? Get Clinical Tree
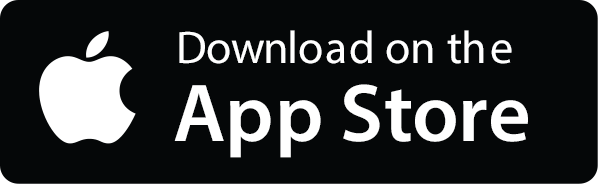
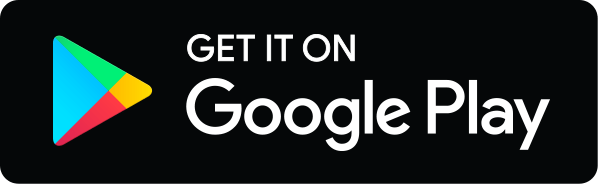