Pulmonary Scintigraphy
Søren Hess
LEARNING OBJECTIVES
1. Summarize the basic challenges in the diagnosis of pulmonary embolism (PE).
2. Describe basic imaging strategies in PE.
3. Perform and interpret ventilation-perfusion pulmonary scintigraphy (V/Q scan).
4. Apply V/Q scanning for non-PE problem-solving.
INTRODUCTION
Acute pulmonary embolism (PE) is a common, ubiquitous, and potentially life-threatening condition. Overall annual occurrence is estimated to be 300,000 to 700,000 in the United States and Europe with annual incidence rates of 75 to 269/100,000 increasing with age to 700/100,000 in patients above age 70. With increasing age demographics, incidences are expected to rise and physicians in virtually every field of clinical medicine will have to care for patients with suspected PE, not only in settings classically associated with high incidences such as emergency departments with a reported incidence of 1/400, but also in patients with chronic medical or psychiatric conditions. Effective anticoagulant treatment is available to reduce morbidity and mortality but is itself associated with potentially dangerous side effects such as bleeding. Thus, accurate diagnosis is imperative to institute correct and timely treatment but also to limit the number of patients subjected to the risks of anticoagulation. However, PE is a notoriously difficult clinical diagnosis due to nonspecific signs and symptoms, and imaging is usually necessary to confirm or exclude the diagnosis. This chapter outlines the basic features of radionuclide ventilation-perfusion pulmonary scintigraphy (V/Q scan) (1,2).
CLINICAL ASPECTS OF PULMONARY EMBOLISM
Basic work on thrombus and embolus including clinical definitions date back to the mid-nineteenth century and German pathologist and polyhistor Rudolph Virchow: a thrombus is a clot forming in the vasculature, and an embolus is a fragment dislodged from a thrombus and transported by the blood stream to another location where it lodges. Although PE is commonly referred to as a blood clot in the lung, the initial fragment may also arise from fat, air, amniotic fluid, or even cement from joint prostheses or vertebroplasty. Nonetheless, PE most commonly arises from deep venous thromboses (DVT) in the lower extremity and there is a close connection between lower extremity DVT and PE, which are together termed venous thromboembolism (VTE). Nearly 50% of proximal DVT progress to PE, and lower extremity DVT, can be found in most cases of PE, whereas non-symptomatic or silent PE is seen in 40% of DVT (3,4).
The pathophysiologic basis for DVT begins with the so-called Virchow’s triad, which describe the three overarching risk factors for DVT: stasis (e.g., immobilization from long-haul travel or bed rest), endothelial damage (e.g., surgery or indwelling catheters), and hypercoagulability (e.g., hereditary disorders, or secondary to pregnancy, disease, or medication). While the factors described by Virchow’s triad may increase the risk of DVT formation, addressing these risk factors, for example, moving about during flights to reduce risk of VTE may be preventative. It is important, though, that risk increases with increasing risk factors present. For example, the risk of DVT from long-haul flights remains low for the average traveler without additional risk factors (3,5).
While history and physical exam are important to ascertain the presence of potential risk factors, signs and symptoms are usually nonspecific (Table 11.1). In symptomatic patients, acute onset dyspnea, chest pain, syncope, and hemoptysis are often present alone or in combination—but these symptoms are also present alone or in combination in many patients presenting to the emergency department (ED) without PE. The same is the case with clinical findings. While heart rate, blood pressure, arterial oxygen saturation, and respiratory frequency should be recorded in patients with acute cardiopulmonary symptoms, no firm differences in physiological signs are found between patients with or without PE (6).
EKG and chest radiographs are also important examinations, primarily for differential diagnoses. Several so-called specific PE-associated findings are described, for example, S1Q3T3-pattern on EKG (S-wave in lead I, Q-wave in lead III, and inverted T-wave in lead III), and Westermark’s sign on chest radiography (focal oligemia). Both signs are in fact nonspecific, the S1Q3T3-pattern is a general sign of right heart strain more than PE specifically, and neither sign is present in more than about 10% of cases (3,6).
In order to improve the selection of patients for further testing, clinical prediction scores have been developed, for example, Well’s score, which based on several disposing factors, symptoms, and signs stratifies patients according to clinical probability for
PE, that is, low (˜10%), intermediate (˜30%), or high (˜65%), respectively, or dichotomized as “PE likely” (˜30%) or “PE unlikely” (˜12%). In conjunction with d-dimer, a fibrin degradation product that is increased in VTE, but also in many non-embolic conditions, clinical scores may guide whether more advanced diagnostic imaging is warranted or not. This has become increasingly important as the actual confirmation rate of PE in patients undergoing diagnostic workup has decreased significantly over the past decades, according to some reports as much as from 50% to 5%, which suggests a significant overutilization of advanced diagnostic procedures (5).
PE, that is, low (˜10%), intermediate (˜30%), or high (˜65%), respectively, or dichotomized as “PE likely” (˜30%) or “PE unlikely” (˜12%). In conjunction with d-dimer, a fibrin degradation product that is increased in VTE, but also in many non-embolic conditions, clinical scores may guide whether more advanced diagnostic imaging is warranted or not. This has become increasingly important as the actual confirmation rate of PE in patients undergoing diagnostic workup has decreased significantly over the past decades, according to some reports as much as from 50% to 5%, which suggests a significant overutilization of advanced diagnostic procedures (5).
Table 11.1 PROPORTION OF SELECTED SYMPTOMS, SIGNS, AND BASIC DIAGNOSTIC RESULTS IN PATIENTS WITH AND WITHOUT PULMONARY EMBOLISM (BASED ON REFERENCE [6]) | |||||||||||||||||||||||||||||||||||||
---|---|---|---|---|---|---|---|---|---|---|---|---|---|---|---|---|---|---|---|---|---|---|---|---|---|---|---|---|---|---|---|---|---|---|---|---|---|
|
OVERALL IMAGING STRATEGIES IN PULMONARY EMBOLISM
The clinical diagnosis of PE remains difficult (6), and according to current international literature, including a recent guideline and a meta-analysis from our own group, controversies remain regarding the diagnostic modality of choice in patients with suspected PE (5,7,8,9,10,11).
Over the years, several imaging modalities have been employed in the diagnostic workup of suspected PE, that is, catheter-based pulmonary angiography, magnetic resonance imaging, V/Q scan, computer tomography angiography (CTA), and positron emission tomography (8,12,13,14). In recent years, the choice has been narrowed to CTA and V/Q scan—both have a place in suspected PE, but with different advantages and shortcomings, and controversies remain regarding first-line imaging (8).
CTA is available around the clock at most hospitals, offering rapid direct evidence for PE or alternative important diagnoses. CTA may not disclose smaller, peripheral PEs, which may be associated with chronic pulmonary hypertension. The radiation dose to breast tissue may be a consideration in younger females (15), and contrast media is administered with the risk of allergic reactions and it may be relatively contraindicated in patients with renal impairment (7,8,9).
On the other hand, with V/Q scan, the radiation burden may be less and no contrast media is employed, but there may be limited access to scintigraphy and radiopharmaceuticals in some settings. Ventilation or aerosol studies can be a challenge with patients in respiratory distress (7). Previously, V/Q scan was characterized by a substantial number of nondiagnostic studies, and it offered no alternative diagnoses (7,15,16,17). Recent developments in 3D-imaging and fusion with low-dose CT have ameliorated this (7,8,18).
PRACTICAL ASPECTS OF V/Q SCAN
The basic principles of V/Q scan have remained virtually unchanged for more than 50 years and are as the name implies based on combined assessment of perfusion and ventilation.
Pulmonary perfusion is assessed by intravenously injected radiolabeled microparticles (i.e., 99mTc-labeled macroaggregated albumin; 99mTc-MAA). The usual adult receives 200,000 to 500,000 particles that comprise an administered patient dose of 100 to 200 MBq mix uniformly with venous blood and distribute uniformly throughout the pulmonary vasculature before they lodge in the precapillary arterioles because the particles are larger (10-90 µm) than the vessel diameter (<15 µm). Tracer distribution is subsequently registered by the gamma camera, but if the vasculature is blocked by an embolus, particles will not reach the vessels distal to it. Thus, obstructed regions remains devoid of radioactivity and appears as photopenic perfusion defects in the scintigraphic images with a wedge-shaped appearance as a consequence of the segmental anatomy of the lungs (19). It seems counterintuitive to essentially induce multiple micro emboli, but due to the vastness of pulmonary vasculature, the particles only transiently block less than 0.1% of the capillary bed. They are subsequently hydrolyzed to smaller particles and phagocytized with a biologic half-life of 3 to 4 h. The dose should be reduced in pregnant women and children, and the number of particles reduced should be reduced to 50,000 to 100,000 in patients with suspected of right-to-left shunt (to minimize the risk of systemic emboli) or pulmonary hypertension (to reduce the risk of severe pulmonary deterioration as the particles lodge more centrally due to reduced lumen) (20), while infants and children typically are prescribed 10,000 to 50,000 particles.
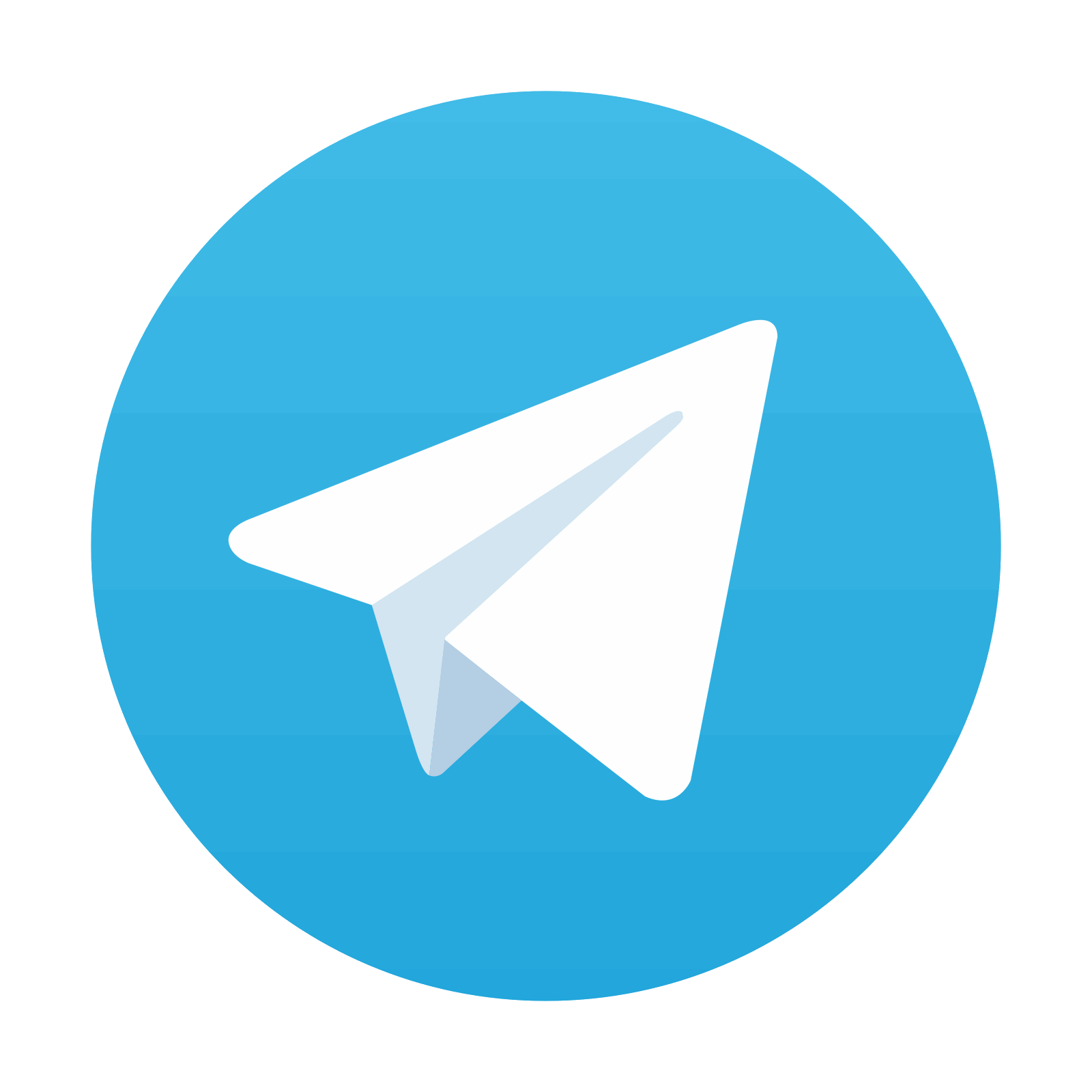
Stay updated, free articles. Join our Telegram channel

Full access? Get Clinical Tree
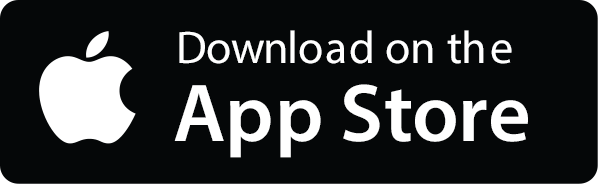
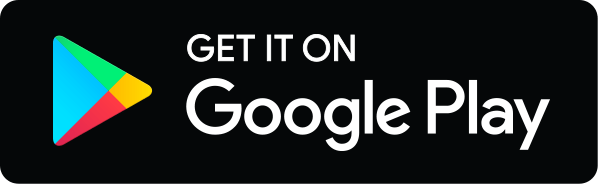