(5.1)
A(t) = the radioactivity at time t.
A 0 = the radioactivity at time t = 0.
λ = the decay constant characteristic of each radionuclide.
e = the base of Napierian logarithms.
The half-life (T 1/2) is related to the decay constant (λ) by the equation:

The half-life is measured with a suitable detection apparatus such as an ionization chamber, a Geiger–Müller counter, and a scintillation counter to determine chronological changes in radioactivity under the same measuring or geometrical conditions. The radioactivity chosen, having regard to experimental conditions, must be of a sufficiently high level to allow detection within a suitable period of time.

(5.2)
5.2.3.2 Gamma-Ray Spectrum Determination
In general gamma-ray spectrometry is used to obtain the characteristic spectrum of a radionuclide that emits gamma rays such as positron emission radionuclides. The gamma spectrum is unique to that nuclide and is characterized by the number of photons of particular energies emitted according to decay scheme. This property contributes to the identification of radionuclides present in a radiopharmaceutical preparation and to their quantification. Furthermore, gamma spectroscopy allows the estimation of the degree of radionuclidic impurity by detecting peaks other than those expected.
5.2.4 Radiochemical Purity
Radiochemical purity is defined as the ratio, expressed as a percentage, of the activity of the radionuclide concerned which is present in the radiopharmaceutical preparation in stated chemical form, to the total radioactivity of that radionuclide present in the radiopharmaceutical preparation. Since the radiochemical form of the radiopharmaceutical determines its biodistribution, the control of radiochemical impurities is important to avoid the patient unnecessary radiation exposure because the radiochemical impurities could have a different biodistribution and not specific uptake which may affect and invalidate the clinical outcome of PET imaging studies. The nature of contaminants will depend on the radionuclide production and on the production process of the radiopharmaceutical as well as the impurity profile of the starting material employed. Radiochemical impurities may also originate from the chemical changes of radiopharmaceutical during storage. Measurement of radiochemical purity requires the use of a method to separate the different labeled chemical species which may be present in the radiopharmaceutical preparation. Chromatographic methods are commonly used in radiochemical determination because they can effectively separate and quantify the radioactive species in the sample. For 18F-FDG preparation, radiochemical impurities include 18F− fluoride ion or radiolabeled intermediates and by-products derived from the synthesis process, such as 18F-2-fluoro-2-deoxy-D-mannose (18F-FDM) and partially or fully acetylated derivatives of 18F-FDG.
5.2.5 Radionuclidic Purity
The radionuclidic purity is defined as the ratio, expressed as a percentage, of the radioactivity of the radionuclide concerned to the total radioactivity of the radiopharmaceutical preparation. Radionuclidic impurities can contribute significant effects on the patient’s overall radiation dose as well as impact the image quality. Radionuclidic impurities can originate from secondary nuclear reactions during the target irradiation as a result of isotopic impurities present in the irradiated material as well as in the target body. The specific monographs prescribe the required radionuclidic purity and may set limits for specific radionuclidic impurities. The most generally used method for radionuclidic purity testing is the gamma spectrometry. For example, the control of radionuclidic purity in 18F-radiopharmaceutical EP monographs prescribes the determination of the amount of 18F and radionuclidic impurities with a half-life longer than 2 hours. The preparation to be examined is retained for at least 24 hours to allow the 18F to decay to a level that permits the detection of such impurities.
5.2.6 Chemical Purity
Chemical purity addresses to not radioactive materials in the PET radiopharmaceutical, including by-products, solvents, and other residual components used in the production process. not radioactive materials (e.g., stabilizers, additives, etc.) that are intentionally added to the PET radiopharmaceutical may also be included in this category. Chemical impurities are considered all not radioactive substances that can either affect radiolabeling or directly produce adverse biological effects in patients. In monographs, chemical purity is controlled by specifying limits on chemical impurities.
To characterize and determine the quantity of potential chemical contaminants in the final product, several methods may be used, including gas chromatography (GC), HPLC, or TLC. Using the example of 18F-FDG, a colorimetric test for the detection of Kryptofix 2.2.2 has been developed. With this test, one can interpret whether the level of Kryptofix 2.2.2 is within the acceptable regulatory limits in the EP monograph for 18F-FDG. Another example of chemical purity determination is in EP monograph of 11C-methionine where is reported the HPLC method for testing the chemical impurities L-homocysteine thiolactone and from DL-homocysteine which derive from the synthesis of this radiopharmaceutical.
5.2.6.1 Residual Solvents
The determination of residual solvents in PET radiopharmaceutical preparations is also a part of the chemical purity testing. Residual solvents are defined as organic volatile chemicals that are used or produced in the manufacture of drug substances or excipients or in the preparation of drug products. The residual solvents are present at trace levels in pharmaceutical substances and their products. These solvents may be the result of the radiopharmaceutical synthesis or from packaging and storage. PET radiopharmaceutical preparations should contain low levels of residual solvents. The EP residual solvent chapter reported the concentration limit and the classification for each solvent set in the International Conference of Harmonization of Technical Requirements for Registration of Pharmaceuticals for Human Use guideline for residual solvents (ICH Q3C R5) [2] which describes the solvents grouped in three classes according to their toxicity:
Class 1 solvents to be avoided in the manufacturing process because of their known unacceptable toxicities or deleterious environmental effects.
Class 2 solvents to be limited because they are associated with less severe toxicity.
Class 3 solvents with low toxic potential and lower risk to human health.
Other solvents for which no adequate toxicological data were found are classified. The residual of these solvents in the radiopharmaceutical preparation should be justified.
Residual solvents are usually determined by chromatographic techniques such as gas chromatography (GC).
5.2.7 Enantiomeric Purity
Enantiomers are either of a pair of optical isomers that are mirror images of each other. Enantiomers exhibit stereoisomerism because of the presence of one or more chiral centers. In the clinical use of chiral radiopharmaceutical, high enantiomeric purity is important since the biological inactive enantiomer could become a disturbing background source of radiation that interferes with interpretation of the signal of active enantiomer. For this reason the enantiomeric purity has to be verified where appropriate. The limit for each enantiomeric impurity is reported in the pharmacopoeia monograph of the radiopharmaceutical: for example, the limit for D-11C-methionine is 10 % of the total radioactivity of the 11C-methionine batch.
5.2.8 Sterility
PET radiopharmaceutical preparations must be prepared using precautions designed to exclude microbial contamination and to ensure sterility. The test for sterility [3] is carried out under aseptic conditions by inoculating the sample in two culture media Soybean-Casein Digest Medium and Fluid Thioglycollate Medium and by incubating for not less than 14 days. Soybean-Casein Digest Medium is a culture media for aerobic bacteria and fungi, while Fluid Thioglycollate Medium is primarily intended for the culture of anaerobic bacteria. Growth promotion tests should be performed by incubating reference bacteria in the two media. Bacterial growth should be visible within the period of incubation. Results of the growth promotion would indicate that both Soybean Casein Digest Medium and Fluid Thioglycollate Medium are capable of supporting bacterial growth; hence, results of the sterility test are reliable. A system suitability test should be performed using two methods: by transferring the solution to be tested in a membrane and by adding to the final portion of sterile diluents used to rinse the filter an inoculum of a small number of viable microorganism (the same used for growth promotion test) (membrane filtration) or by transferring the solution to be tested to the culture medium and by adding to the medium an inoculum of a small number of viable microorganism (the same used for growth promotion) (direct inoculation). The solutions are then incubated for not more than five days. If clearly visible growth of microorganism is obtained after incubation, visually comparable to that in the control vessel without product, the product possesses no antimicrobial activity under the conditions of the test so the test for sterility may then be carried out without further modifications. If clearly visible growth is not obtained, the conditions should be modified in order to eliminate antimicrobial activity of the product, and the system suitability should be repeated.
5.2.9 Bacterial Endotoxin Test
Bacterial endotoxins (pyrogens) are polysaccharides from bacterial gram-negative membranes which are water-soluble, heat stable, and filterable. Their presence in a radiopharmaceutical preparation can cause fever and also leukopenia in immunosuppressed patients. To minimize the presence of pyrogens, it is important that preparations are manufactured and dispensed under aseptic conditions. The test for bacterial endotoxins is used to quantify endotoxins, and it is based on the blood-clotting reaction that occurs when bacterial endotoxins activate the blood cell component Limulus amebocyte lysate (LAL) derived from horseshoe crabs (Limulus polyphemus or Tachypleus tridentatus). The test may be conducted with one of the following techniques reported in the EP chapter for bacterial endotoxins [4]:
Gel-clot technique
Turbidimetric technique
Chromogenic technique
The limit for bacterial endotoxins is indicated in the individual monograph.
5.2.9.1 Gel Clot Technique
The gel-clot technique is based on clot formation in the reaction between endotoxins and LAL. The gel-clot method involves the use of a clotting protein that is cleaved by an activated clotting enzyme, at which point the insoluble cleavage products coalesce by ionic interaction to form the gel. Although the entire reaction has not been determined, it is understood that the reaction leading to clot formation involves a cascade of enzyme activation steps.
5.2.9.2 Turbidimetric Technique
This technique observes the changes in turbidity associated with the clotting protein formed in the reaction between endotoxins and LAL. Endotoxin concentration can be determined by the quantitative relationship between endotoxin concentration and turbidity (absorbance or transmission) of the reaction mixture at the end of incubation period (end-point-turbidimetric test) or by measuring the time needed for the reaction mixture to reach a predetermined absorbance or transmission or the rate of turbidity (kinetic-turbidimetric test).
5.2.9.3 Chromogenic Technique
This technique uses a chromogenic peptide which releases a chromophore (e.g., p-nitroaniline (pNA)) by the reaction of endotoxins with LAL. In the presence of endotoxin, the components of LAL are activated in a proteolytic cascade that results in the cleavage of a colorless artificial peptide substrate. Proteolytic cleavage of the substrate liberates p-nitroaniline (pNA), which has an absorbance of 405 nm. Endotoxin concentration can be determined by the quantitative relationship between the endotoxin concentration and the amount of chromophore released at the end of incubation period (end-point-chromogenic test) or by measuring the time needed for the reaction mixture to reach a predetermined absorbance or the rate of color (kinetic-chromogenic test).
5.3 Analytical Techniques Used in Quality Control of PET Radiopharmaceuticals
Chromatographic separation methods are commonly employed in the quality control of PET radiopharmaceuticals. Chromatography is an analytical technique based on the separation of two or more compounds by the distribution between two phases, a stationary and a mobile phase. These two phases can be solid–liquid, liquid–liquid, or gas–liquid. Chromatographic separation methods are used to separate radioactive or not radioactive species in the sample followed by their identification and quantification and are commonly employed in radiochemical, chemical, and enantiomeric purity determinations. The most used chromatographic methods are high-performance liquid chromatography (HPLC), thin-layer chromatography (TLC), and gas chromatography (GC) (Table 5.1).
Table 5.1
Chromatographic methods employed in radiochemical, chemical, and enantiomeric purity determination in some PET radiopharmaceuticals described in EP monographs. NR not required
Radiochemical purity | Chemical purity | Enantiomeric purity | |||||
---|---|---|---|---|---|---|---|
Radiopharmaceutical | TLC | HPLC | TLC | HPLC | GC | TLC | HPLC |
18F-FDG | X | X | X | X | X | NR | NR |
11C-methionine | X | X | – | X | X | X | – |
11C-acetate | – | X | – | X | X | NR | NR |
18F-NaF | – | X | – | X | NR | NR | NR |
18F-FLT | X | X | X | X | X | NR | NR |
18F-FDOPA | X | X | – | X | X | X | – |
18F-FMISO | X | X | X | X | X | NR | NR |
68Ga-Edotreotide | X | X | X | X | X | NR | NR |
5.3.1 High-Performance Liquid Chromatography (HPLC)
High-performance liquid chromatography (HPLC) is a type of liquid chromatography used to separate and quantify compounds that have been dissolved in solution. HPLC utilizes a column that holds packing material (stationary phase), a pump that moves the mobile phase through the column, an injector to introduce the sample in a small volume, and a detector that shows the retention times of the molecules for identification and quantification. Retention time varies depending on specific chemical or physical interactions between the stationary phase, the molecules being analyzed, and the solvent used as a mobile phase. Types of HPLC generally depend on phase system used in the process. Following types of HPLC are commonly used in the quality control of radiopharmaceuticals:
- 1.
Normal-phase chromatography (NP-HPLC)
- 2.
Reversed-phase chromatography (RP-HPLC)
- 3.
Ion-exchange chromatography
NP-HPLC separates analytes based on polarity. NP-HPLC uses a polar stationary phase and a not polar mobile phase. The polar analyte interacted with and is retained by the polar stationary phase. RP-HPLC has a not polar stationary phase and an aqueous, moderately polar mobile phase. RP-HPLC operates on the principle of hydrophobic interactions, which result from repulsive forces between a polar eluent, the relatively not polar analyte, and the not polar stationary phase. In ion-exchange chromatography, retention is based on the attraction between analyte ions and charged sites bound to the stationary phase. Ions of the same charge are excluded and eluted with the mobile phase. There are different types of detectors that can be used for HPLC. The detector is used to detect the presence of a compound passing through and to provide an electronic signal to a data-acquisition device. In HPLC analysis of PET radiopharmaceuticals, radio-detectors are used such as NaI scintillator or BGO radiodetector to determine the radiochemical purity. Radioactivity may be measured by integration using an automatic-plotting instrument or a digital counter. The ratios of the areas under the peaks give the ratios (or percentage) of the radioactive concentration of the compounds (Fig. 5.1). Moreover, the retention time of each compound allows radiochemical identification by comparison with solutions of the same not radioactive chemical substances (cold standard) using a suitable detection method. Ultraviolet (UV–VIS), electrochemical, conductivity, and refractive index (RI) detectors are commonly connected to radiodetectors in order to determine the radiochemical identity of the radiopharmaceuticals and the amount and identification of chemical impurities.
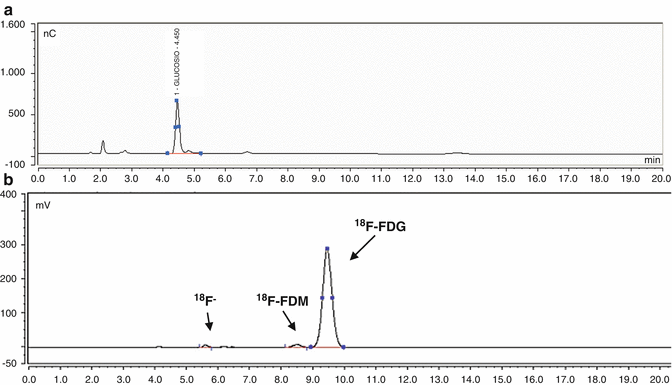
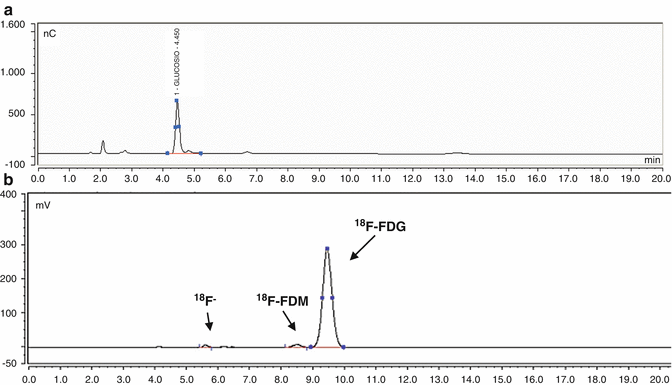
Fig. 5.1
(a) ECD-PAD chromatogram of 18F-FDG preparation, (b) radio-chromatogram: 18F−, free ion fluoride; 18F-FDM, 18F-2-fluoro-2-deoxy-D-mannose. HPLC conditions: stationary phase CarboPac PA10 column, mobile phase NaOH 0.1 M, and flow rate 1 ml/min
5.3.2 Thin-Layer Chromatography (TLC)
Thin-layer chromatography (TLC) is a solid–liquid form of chromatography where the stationary phase is normally a polar absorbent and the mobile phase can be a single solvent or combination of solvents. TLC technique can be used to determine the number of components in a mixture or to verify a substance’s identity. In thin-layer chromatography, the stationary phase is a polar absorbent, usually finely ground alumina or silica particles. For enantiomeric purity determination, a chiral reagent absorbed to an inert support is used. This absorbent is coated on a glass slide or plastic sheet creating a thin layer of the particular stationary phase. Almost all mixtures of solvents can be used as the mobile phase. The TLC plate is marked at the origin and at the front distance. The sample of radiopharmaceutical is spotted to the origin in volume equal or less than 10 μl with microsyringes or micropipettes, and the TLC plate is transferred to the development chamber filled with a small amount of mobile phase (Fig. 5.2a, b). The solvent, which is in the bottom of the chamber, travels up the layer of adsorbent by capillary action, passes over the spot, and, as it continues up, moves the compounds in the mixture up the plate at different rates. The result is the separation of the compounds due to the differences in their affinity to the stationary phase and because of differences in solubility in the solvent. Once the solvent has run to the front mark, the plate is removed.
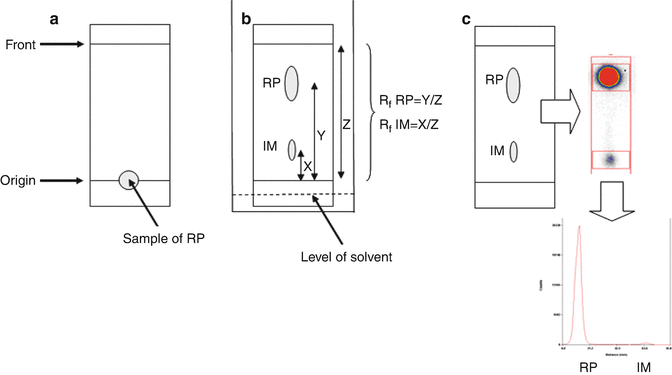
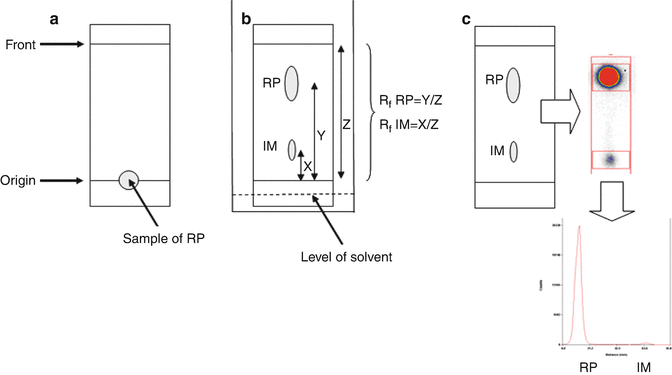
Fig. 5.2
TLC analysis of PET radiopharmaceutical. (a) Sample spotting, (b) development, (c) detection and integration. RP radiopharmaceutical, IM impurity
After development, the support is dried, and the position of radioactive spots is detected by autoradiography or by measurement of radioactivity over the length of the chromatogram using suitable collimated counters. Radioactivity may be measured by integration using an automatic-plotting instrument or a digital counter. The ratios of the areas under the peaks give the ratios of the radioactive concentration of the chemical substances to determine the radiochemical purity (Fig. 5.2c). TLC can also provide a chromatographic measurement known as an R f value. The R f value is the “retardation factor” or the “ratio-to-front” value expressed as a decimal fraction. The R f value can be calculated as:
R f calculation of the spots or areas allows chemical identification by comparison with solutions of the same not radioactive chemical substances (cold standard) using a suitable detection method.

5.3.3 Gas Chromatography (GC)
Gas chromatography (GC) is a gas–liquid chromatography which involves a sample being vaporized and injected onto the head of the chromatographic column. The sample is transported through the column by the flow of inert, gaseous mobile phase. Separation of each sample component is achieved by repeated distribution between two phases. Commonly used mobile phases are N2, He, or Ar. GC columns can be packed or capillary. Packed columns contain a finely divided, inert, solid support material (commonly based on diatomaceous earth) coated with liquid stationary phase, while capillary columns can be wall-coated open tubular (WCOT) or support-coated open tubular (SCOT). Wall-coated columns consist of a capillary tube whose walls are coated with liquid stationary phase. In support-coated columns, the inner wall of the capillary is lined with a thin layer of support material such as diatomaceous earth, onto which the stationary phase has been adsorbed. Transport of the analytes is achieved in the gas phase; separation is accomplished in the stationary phase.
The quality of a separation depends on how long the components to be separated stay in the stationary phase and on how often they interact with this phase. The type of interaction between component and phase is determined by the functional groups. The polarity of the phase is a function of stationary phase substituents. The temperature of the column can be varied during the analysis improving the separation. The time taken for a particular compound to travel through the column to the detector is known as its retention time. This time is measured from the sample injection to when a maximum peak height for that compound is displayed. There are several different types of detectors in use. The flame ionization detector (FID) is the most commonly used.
Headspace gas chromatography (HSGC) is a GC technique where the liquid sample is set in a closed vessel until the volatile components reach equilibrium between the sample and the gas volume above. An aliquot of the gas volume (headspace) is sampled and introduced into a GC column for analysis. Direct injection of analytes evaporated through equilibration between liquid phase and gas phase minimized GC system contamination and column deterioration. In addition, the automation of equilibrium and injection procedure reduces analysis time and improves reproducibility. Due to the volatility of organic solvents, GC is commonly used to identify and quantify the presence of residual solvents in radiopharmaceutical preparations.
5.3.4 Gamma Spectrometry
Gamma-ray determination measures gamma photons emitted from radionuclides. Gamma-ray spectrometers use the direct proportionality between the energy dissipated by incoming gamma radiation into the detector and the pulse amplitude at the output of the detector. After amplification and digitization, the pulse amplitudes are analyzed, and the output of the spectrometer is an energy spectrum of detected radiation. Since individual radionuclides emit specific gamma-ray energies, gamma-ray spectra can be used to perform a qualitative analysis of a sample, identifying the radionuclides present. This requires a calibration of the system as a function of energy. Quantitative analysis is also possible, by measuring the areas of specific peaks and correcting for the system efficiency and other factors. The calibration in energy and efficiency must be made for the specific energies of interest or in a range of energies (e.g., 50–2000 keV). To this end, certified sources supplied by an accredited laboratory are used; these sources have specified level of accuracy and are traceable to the international system of measurement [5]. Gamma-ray spectrometry is used in the radiopharmaceutical field for:
The identification of gamma-emitting radionuclidesStay updated, free articles. Join our Telegram channel
Full access? Get Clinical Tree
