7Radiation risk estimation for persons exposed by radioiodine as a result of the Chornobyl accident As a result of the Chornobyl accident in 1986, much of the territories of Ukraine, Belarus, and Russia were subjected to radioactive contamination, and inhabitants of these territories to radioactive exposure. The most significant was the thyroid exposure due to intake of iodine radioisotopes, primarily of 131I (Likhtarev et al., 1993a, 1993b, 1995b). Already in 5–6 years after the accident a dramatic increase began to be exhibited in the thyroid cancer incidence of children and adolescents, which resided in the areas where the estimates of thyroid exposure doses occurred to be quite high (Likhtarev et al., 1995a; Buglova et al., 1996). In fact, the growth of thyroid cancer incidence of children and adolescents caused by internal thyroid exposure from Chornobyl fallout was the main statistically significant remote effect of the Chornobyl accident. It comes as no surprise that the phenomenon caused enormous interest of radio-epidemiologists all over the world so that a series of studies was conducted in Ukraine, Belarus, and Russia (Jacob et al., 2006; Likhtarov et al., 2006a; Tronko et al., 2006; Kopecky et al., 2006; Zablotska et al., 2011). The interpretation of results of most radio-epidemiologic studies was based on a number of assumptions, primarily on the estimates of exposure doses. The assumptions include the following: –It was recognized that the dose estimates include uncertainty which is typically significant. –Even in the cases where the level of dose errors turned out to be determined, the analytical procedures of risk analysis ignored that fact. As a result of the above-mentioned general properties of the dosimetric support for epidemiological studies, it was the merely stochastic nature of thyroid cancer cases that was taken into account in analytical procedures of risk analysis, whereas the exposure doses of subjects were assumed precise. Studies performed by Kukush et al. (2011) and Masiuk et al. (2016) demonstrated that the dose uncertainties can be quite correctly taken into account in the process of risk analysis. Some difficulty lies in the fact that the main sources of dose uncertainties are related to errors in estimation of the weight of exposed organ, instrumental measurements of radioactivity of the organ at certain moment, and the ecological component of dose. The papers by Kukush et al. (2011) and Masiuk et al. (2016) show that the estimates of thyroid weight and ecological component of thyroid dose include Berkson error, and the instrumental measurements contain the classical error. The size of Berkson error is easily estimated by the Monte Carlo method (Likhtarov et al., 2014), while a specific analysis is required to estimate the size of the classical error. Conducting any campaign of mass measurement of radioiodine isotopes uptake in thyroid gland (further called thyroid dosimetric monitoring) is always limited in time for solely physical reasons. This is due to the fact that the most long-lived radioisotope of iodine 131I has a half-life of 8 days, and within 5–8 weeks after the accidental emission of radioisotope mixture in the environment the gamma radiation from the radioiodine gets invisible against the background of cesium radioisotopes. Since the deployment of thyroid dosimetric monitoring is always associated with necessity to solve a lot of organizational and technical issues, consequently the monitoring itself should be carried out in less than a month. The need for coverage by measurements as many people in the areas undergone by the accident as possible and being combined with short term of the monitoring leads to the fact that such a monitoring has significant differences from laboratory studies. First of all they are: attraction of staff without any experience in dosimetry, use of nonspecialized equipment and many types of devices, reducing endurance in the measurements, and simplified calibration of devices. All this leads to the values of measurement errors that are significantly larger than in laboratory studies, and also to the appearance of new error components, which would be avoided during laboratory tests. The thyroid dosimetric monitoring in the areas affected by the Chornobyl accident was conducted by special emergency teams under supervision of the Ministry of Public Health of the UkSSR. Advisory assistance was provided to them by a team from the Research Institute of Hygiene of Maritime Transport (Leningrad city), which developed a general method of measurement and provided emergency crews by the referent sources of radioisotope 131I necessary for calibration of devices. The first measurements were taken in mobile radiometric laboratories (MRL), in which the spectrometers brought from the city of Leningrad were used. The purpose of these measurements was to obtain initial estimates of doses and estimates of risk level due to the radioiodine pollution. After obtaining the first results, it was decided to expand the scope of measurements by connecting to them local medical institutions and available equipment. Thus, during the thyroid dosimetric monitoring the measurements were carried out by devices of two main types: energy-selective spectrometers (usually single-channel ones) and integrated radiometers (Likhtarev et al., 1995b; Likhtarov et al., 2015). Table 7.1 presents data of all the models of spectrometric and nonspectrometric devices that were used in various oblasts of Ukraine, as well as the appropriate number of measurements performed by the devices. Table 7.1: The peculiarities of the thyroid dosimetric monitoring in 1986: types and models of the devices used, the number of measurements, the duration of the monitoring. As seen from Table 7.1, more than half of all the measurements were made by integrated radiometers SRP-68-01. Using devices of this type, the greatest amount of the measurements was performed in Zhytomyr, Odessa, and Chernihiv Oblasts, and also in the Crimea. As to measurements made by the spectrometric instruments, the most of them were done by specialized thyroid radiometer GTRM-01ts (mainly in Chernihiv and Zhytomyr Oblasts) and also by universal window radiometers NC-150 and NC-350 produced by the plant “Gamma” (Hungary). In whole, the most number of the measurements were performed in Zhytomyr, Chernihiv, Odessa Oblasts, and in the Crimea. As a rule, the scheme of measurements was as follows. The measurements were carried out in well-ventilated premises with hourly wet cleaning. To reduce background of radiation, the detectors of devices were protected with lead collimators. For field radiometers SRP 68-01 with factory kitting without collimator, the homemade collimators were used made of scrap materials7. During the measurement, the detector device was being brought to the neck of a person measured, before having cleaned with a cotton wool wet in alcohol, then single counting the number of pulses or their intensity was written down in sheet. Every hour or every day the background in the same point of space was measured and its value was written down to the measuring list. Also, in order to make a calibration, the count of pulses coming from a bottle phantom (cylindrical bottle of 10 ml containing a reference solution of isotope 131I) was being fulfilled either hourly or daily. The results of measurements performed in the same settlement in the same day and by the same device and by the same team were recorded in so-called measuring list. (A typical list contained the results of 100–200 individual measurements, although in some cases the number of measurements performed by the team during the day could be about thousand.) In conformity with the established requirements to the measurement data, there were also to be recorded: the personal data of person (name, date or even year of birth), information on dosimetric team, on type of measuring device, the results of the device’s calibration (calibration factor), and the value of radiation background in the room. Unfortunately, usually not all the data mentioned above were written down in the lists. Some of them had to be recovered during several cycles of the data processing. Throughout June 1986, the bodies of people residing in contaminated areas were continuing to accumulate cesium radioisotopes, while 131I was continuing to disintegrate quickly. In this regard, in early June the thyroid dosimetric monitoring was decided to be finalized. Some measurements of thyroid activity against the background of growing cesium exposure lasted until the end of June, but 98% of all measurements were made up to June 6, i.e., the bulk of the monitoring was held during a period less than a month. Thus, a huge organizational work done in short terms gave medical workers a unique array of data with more than 150 000 measurements of 131I content in the thyroid of residents from the most contaminated areas of northern Ukraine: Zhytomyr, Kyiv, and Chernihiv Oblasts. Of these, about 112 thousand of measurements were conducted among children and adolescents aged from 0 to 18 years (Likhtarev et al., 1993a, 1993b, 1995b; Likhtarov et al., 2015). At the beginning of mass measurements of 131I content in the thyroid, a considerable part of children and adolescents from the suffered areas was removed to summer vacation spots in southern, the least suffered Oblasts of Ukraine, and the population of the 30 km zone adjacent to the Chornobyl nuclear power plant was evacuated completely. Therefore, about 47,000 measurements were performed at the territory of 10 Oblasts being rather far from Chornobyl, whereas about 103 000 measurements were made within the three northern Oblasts of Ukraine, in the areas distinguished by significant radionuclide contamination. In general, the estimate of the content Q of iodine radioisotopes in the thyroid being the result of a direct measurement is defined as where Kb is a calibration factor (CF) of measuring device from the bottle phantom; G is correcting coefficient to the CF which takes into account the difference of the measurements geometry between reference source (the bottle phantom) and a subject of the measurements; Ith and Ibg show the device indication8 during the measurement of thyroid gland and gamma background, respectively; fsh is the coefficient of gamma background screening by body of the subject and is a function dependent on both the subjects’ anthropometric parameters and spectral characteristics of the background. According to the literature, fsh is in the range 0.9–1 (Pitkevich et al., 1996; Zvonova et al., 1997). The CF is determined by measurement of the reference radiation source with its activity Qref being known in advance. When calibrating by the bottle phantom G = 1 (due to the definition of correction coefficient) and fsh = 1, then from (7.1) we have i.e., CF is numerically equal to radioactivity, which corresponds to the device’s indication unit and is the value reversed to the sensitivity of the device. In general, the device sensitivity can vary in time for many reasons (e.g., because of the temperature dependence of the parameters of electronics). Therefore, to minimize errors in the measurement results it is advisable to calibrate the device immediately prior to the measurements of the subjects. This in turn implies that the dosimetric team should have a reference radiation source. During mass monitoring in 1986, the devices’ calibration was performed using a bottled phantom. The calibration factors Kb obtained through the bottle phantoms should be corrected by a factor G, which accounts for the influence of geometry of the measurements, physical characteristics of the thyroid, and coating tissue in the neck area to a signal of the device’s detector (equation (7.1)). Since the size of thyroid is a function of person’s mass, and therefore, a function of age and gender, the coefficient G is also dependent on age and gender. For calculation of the correction coefficients for devices with collimators of any depth, the human thyroid was modeled as two double-axis ellipsoids of revolution. In this case, the G is the ratio of intensities registered by a detector of radiation coming from a model of thyroid and a model of bottled phantom, provided they have the same content of radioiodine in them. Figure 7.1 demonstrates the CF value received by phantom experiments and corrected by the value of correcting coefficient G (Likhtarov et al., 2015). For comparison, empirical values of the CF are also presented for three age groups obtained in Kaidanovsky and Dolgirev (1997) on volunteers using SRP-68-01 with absent collimator. Thus, in the absence of calibration procedure for the radiometers SRP-68-01 as a value of the CF, we used the value Kb = 90 Bq h/μR adjusted for the age-dependent correction factor G. It is known (Gol’danskii et al., 1959) that at the fixed intensity of emission for a radioactive source n, the probability to register k counts using a measuring device (such as the Geiger–Muller counter) for the time t is defined by the Poisson distribution with parameter nt: Based on (7.3) and the described above measurement methods of radioactivity 131I in the thyroid, we obtain where Q is the radioactivity of 131I in the thyroid, kth is the number of pulses registered by the device when measuring the radioactivity of 131I in the thyroid during the time tth of measurement, kbg is the number of pulses registered by the device when measuring radioactivity background during the time tbg of measurement, fsh is screening coefficient for the background radiation, and K is the age-dependent calibration factor. The latter is the CF of device from the bottle phantom Kb adjusted with the age-dependent geometric correcting factor G, Because for large enough n, the Poisson distribution (7.3) is close to normal law (Molina, 1973), we can write where N(m, σ2) is normal law with expectation m and variance In addition to the statistical error of registration, the values where δK is based on the error of reference source 131I and the device’s error. Using (7.6)–(7.8), expression (7.4) can be modified as where From (7.9), we get Since then substituting (7.11) in (7.10), we get where Inasmuch as Finally, we get the observation model of thyroid radioactivity with the classical additive error: The error δK of the age-dependent calibration factor K specified in (7.5) can be found as
7.1Estimation of error level in direct thyroid radioactivity measurements for children and adolescents exposed by 131I in May–June 1986
7.1.1Peculiarities of organization and performance of the mass thyroid dosimetric monitoring
7.1.2Implementation of thyroid dosimetric monitoring at the territory of Ukraine in 1986
7.1.3Calibration of measuring devices
7.1.4Estimation of errors for direct measurements of the content of radioiodine in the thyroid
,
are intensities of a radioactive source registered during the measurement of thyroid and background, respectively, and
,
are the variances of measurement errors. Index tr means the true value, while mes means the measured one.
and
contain one more instrumental error, with variance
. One can estimate the full variances of measurement errors for both thyroid and background:
and γ2 ∼ N(0, 1).
and
are unknown, the estimate of
will be the following:
7.1.5Errors of the device calibration
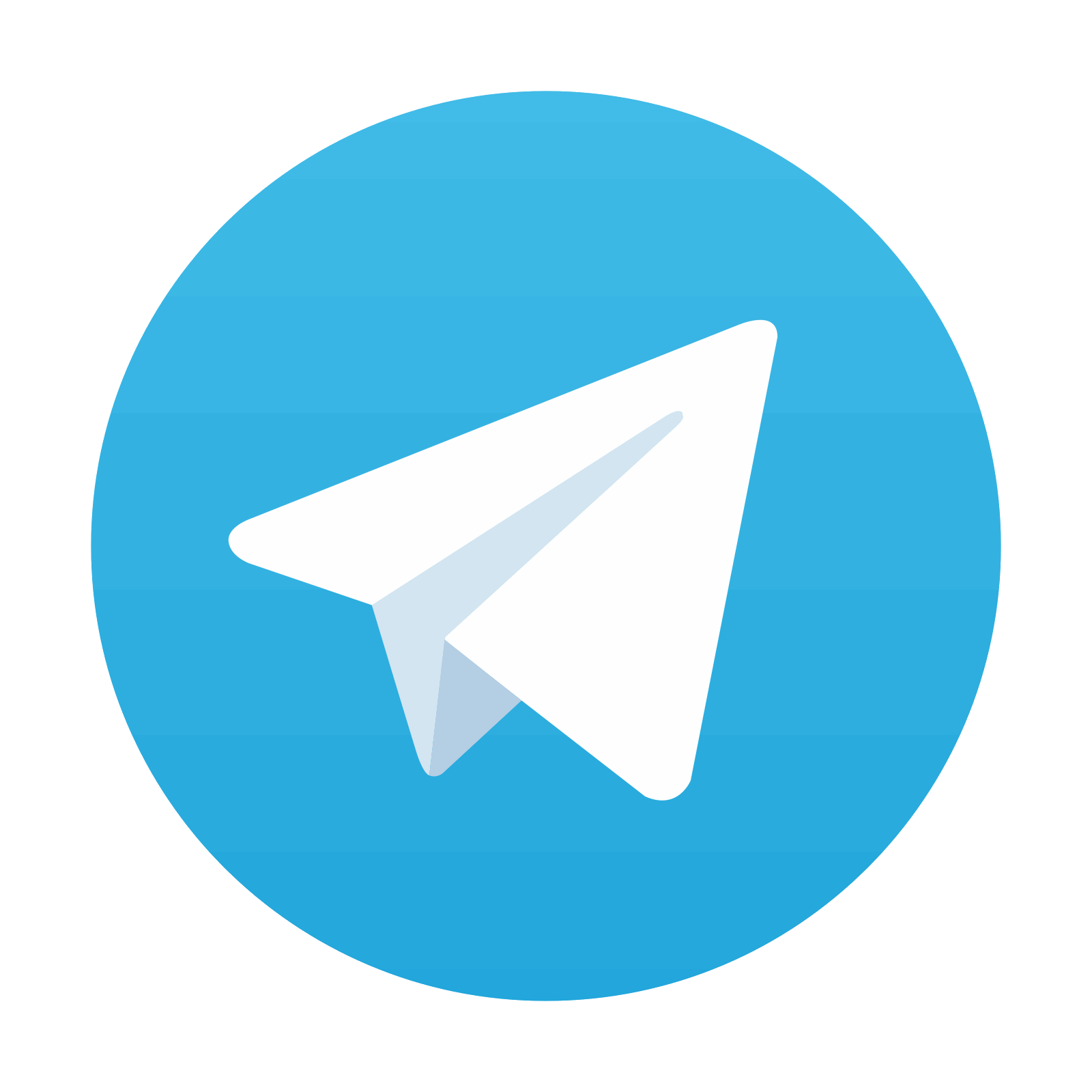
Stay updated, free articles. Join our Telegram channel

Full access? Get Clinical Tree
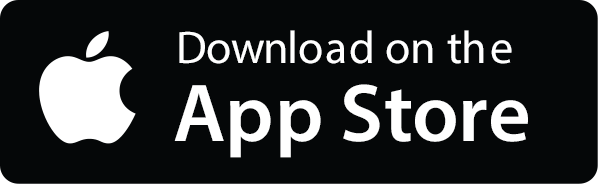
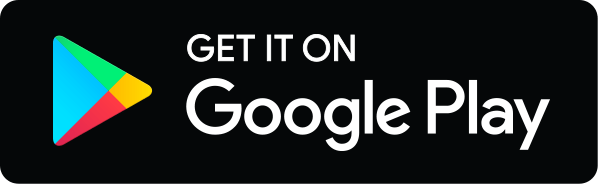