Group
T
N
M
PSA
Gleason
I
T1a–c
N0
M0
PSA <10
Gleason ≤6
T2a
N0
M0
PSA < 0
Gleason ≤ 6
T1–2a
N0
M0
PSA X
Gleason X
IIA
T1a–c
N0
M0
PSA <20
Gleason 7
T1a–c
N0
M0
PSA ≥10–<20
Gleason ≤6
T2a
N0
M0
PSA <20
Gleason ≤7
T2b
N0
M0
PSA <20
Gleason ≤7
T2b
N0
M0
PSA X
Gleason X
IIB
T2c
N0
M0
PSA any
Gleason any
T1–2
N0
M0
PSA ≥20
Gleason any
T1–2
N0
M0
PSA any
Gleason ≥8
III
T3a–b
N0
M0
PSA any
Gleason any
IV
T4
N0
M0
PSA any
Gleason any
T any
N1
M0
PSA any
Gleason any
T any
N any
M any
PSA any
Gleason any
General Treatment Strategies
Common Treatment Strategies for Localized Prostate Cancer
General treatment strategies for early-stage prostate cancer include radical prostatectomy, external beam radiation therapy, brachytherapy, androgen deprivation therapy, and watchful waiting per NCCN Version 1.2015 guidelines for prostate cancer treatment. External beam radiation therapy, brachytherapy, and androgen deprivation therapy are often used as bi- or trimodality therapy combinations based on the aggressiveness of the disease in terms of probability of locoregional spread.
Role of Brachytherapy in Prostate Cancer Treatment
Prostate cancer patients with localized, organ-confined disease or limited extraprostatic extension are candidates for prostate brachytherapy. Table 14.2 summarizes general treatment guidelines for patients to be treated with brachytherapy. Patients with NCCN low-risk group disease are candidates for brachytherapy as monotherapy, patients with intermediate-risk group disease are candidates for brachytherapy with or without supplemental external beam radiotherapy, and patients with high-risk group disease should receive supplemental external beam radiotherapy and androgen deprivation therapy [5].
Table 14.2
Suggested treatment algorithm for low-, intermediate-, and high-risk prostate cancer to be treated with brachytherapy as recommended by the American Brachytherapy Society
NCCN low-risk group | NCCN intermediate-risk group | NCCN high-risk group | |
---|---|---|---|
Brachytherapy as monotherapy | Favored | Optional | Not recommended |
Combination brachytherapy and external beam radiation | Not recommended | Optional | Optional |
Inclusion of androgen deprivation | Not recommendeda | Optional | Favored |
Selecting Patients for Brachytherapy
When deciding whether or not to use brachytherapy, either alone or in combination with EBRT, it is useful to consider pretreatment urologic symptoms and prostate size. Acute urologic symptoms may also be more severe with brachytherapy, which makes it less ideal for patients with significant symptoms of benign prostatic hypertrophy (BPH) and those with large glands, the general cutoff being 50 cubic centimeters. Urinary symptoms are commonly rated using the AUA IPSS, which is the same as the BPH symptom score but with a quality-of-life (QOL) question added. In the phase III trial, RTOG 0815, patients with an AUA score of greater than 15 are not candidates for brachytherapy, and the size cutoff is less than 60 g. Also, a history of transurethral resection of the prostate (TURP) is considered a relative contraindication for brachytherapy because of the increased risk of urethral toxicity, including incontinence [6]. Other factors that may contribute to higher acute toxicity include a greater number of needles used to do the implant and the use of hormonal therapy [7].
The American Brachytherapy Society recommends the following absolute contraindications to TRUS-guided PPB: limited life expectancy, unacceptable operative risks including poor suitability for general or spinal anesthesia, presence of distant metastases, anatomic or surgical absence of the rectum such that TRUS guidance is not possible, large TURP defects which preclude seed placement and acceptable radiation dosimetry, and a history of ataxia-telangiectasia. Recommended relative contraindications include: IPSS >20, history of prior pelvis radiotherapy, prior TURP, prominent median lobe architecture, prostate volume >60 cubic centimeters, and history of inflammatory bowel disease [5]. It is emphasized that published reports demonstrate excellent clinical results in patients with relative contraindications if meticulous patient selection is used and treatment is performed by an experienced brachytherapy team in a multidisciplinary environment.
Combination External Beam Radiation Therapy and Brachytherapy
In theory, the higher incidence of extracapsular extension and seminal vesicle invasion in intermediate- to high-risk patients is more adequately covered by EBRT than brachytherapy, which is the rationale for combining EBRT and brachytherapy alone in these patients. On the other hand, certain intermediate-risk patients are likely to be candidates for brachytherapy alone. In a retrospective review of 668 brachytherapy patients, there was no statistically significant difference in the 8-year actuarial biochemical relapse-free survival (BRFS) between low- and intermediate-risk patients, 98.4 and 98.2 %, respectively [8]. These data suggest that most intermediate-risk patients have a low risk of micrometastatic disease in the pelvis. Even though the risk of extracapsular disease is higher in these patients, these areas may be adequately covered by an implant, since most extracapsular disease is located within 5 mm of the prostate capsule. In another series of combination therapy, where 63.4 % of the intermediate-risk patients had 50 % or more positive biopsy needle cores, there was an 80 % 15-year BRFS [8]. These data suggest excellent long-term control with the combined approach. However, despite high control rates, the additional use of EBRT may be detrimental in terms of higher rates of morbidity. Therefore, additional pathologic factors, such as number or percentage of positive cores, may be helpful to determine whether or not combination treatment should be used. There is a national trial RTOG 0232, which has accrued and closed at the time of publication, that randomized intermediate-risk group patients to brachytherapy alone versus combination therapy. The results of this trial will inform decision making regarding this patient group.
Imaging Correlations with Emphasis on Image Fusion Guidance
Only recently has imaging played a significant role in the diagnosis and staging of localized prostate cancer. Historically, bone scans and CT scans were used to assess for pelvic nodal metastases and distant metastatic disease, but there was little role for imaging to evaluate the primary tumor. However, with the influx of modern technologies, such as high-resolution ultrasound and multiparametric MRI, the primary tumor site can be anatomically and oncologically well characterized, leading to better staging and risk stratification of patients and refining the intraoperative interventional management of these patients. Accurate definition of the location of the tumor in the prostate gland improves the staging of prostate cancer and advises the patient’s management team regarding decisions such as whether LDR or HDR brachytherapy may be a superior approach for a given patient or if supplemental treatments like external beam radiation therapy or androgen deprivation therapy should be recommended for a given patient.
Diagnosis
Transrectal ultrasound (TRUS) was the first modality widely to evaluate the local extent of prostate cancer and remains the most widely used imaging modality for biopsy guidance and image-guided treatments including brachytherapy source placement. However, even with improvements in ultrasound technology such as color Doppler, color power Doppler, microbubble contrast enhancement, and ultrasound elastography, TRUS is unable to reliably differentiate prostate cancer from normal prostate glandular tissue, and TRUS-guided biopsy can miss up to one-third of clinically significant prostate cancers [9, 10].
MRI of the prostate gland was first performed in the mid-1980s with excellent anatomic visualization [11]. The T2-weighted images provide excellent spatial resolution and can differentiate the more hyperintense peripheral from the lower signal intensity central and transition zones. In the T2 images, a focus of prostate cancer can appear with low signal intensity in the area of hyperintense parenchyma of the normal peripheral zone [12]. Hypointense lesions can also represent foci of benign hyperplasia, prostatitis, focal prostate atrophy, or post-biopsy hemorrhage or similar processes. The degree of signal reduction in a cancer lesion correlates, in some cases, with the grade and growth pattern of the tumor [13, 14]. T2-weighted MRI also offers the enormous benefit of assessing the extent of local disease. Extracapsular extension can be identified through asymmetry of the neurovascular bundles, obliteration of the rectoprostatic angle, irregular bulging of the prostatic margin, and low-signal tumor in the periprostatic fat. Seminal vesicle invasion can be similarly identified. Figure 14.1 demonstrates a T2 hypointense lesion with extracapsular extension.
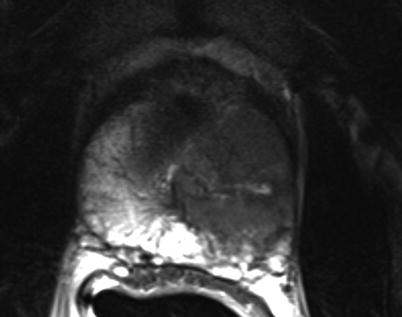
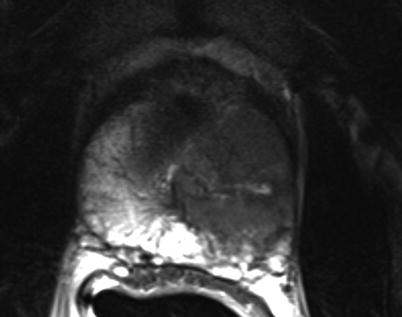
Fig. 14.1
MRI demonstrating a T2 hypointense lesion with extracapsular extension
More recently, the ability to obtain functional information from the MRI refined prostate cancer diagnosis and staging. Specifically, dynamic contrast-enhanced MRI (DCE-MRI), diffusion-weighted MRI (DW-MRI), and proton magnetic resonance spectroscopic imaging (MRSI) are all becoming more widely used in the evaluation of a potential prostate brachytherapy patient. DCE-MRI is able to detect changes in the local vasculature as prostate tumors secrete pro-angiogenic agents, such as vascular endothelial growth factor, leading to recruitment of neovasculature, increased vascular permeability, and increase in volume of the tumor interstitial space [15, 16]. With microcompartmental modeling, the time versus intensity curves of contrast enhancement can be plotted, and the parametric images can be fused with T2-weighed images. DWI-MRI is based on the thermally induced ransom molecular displacement (Brownian motion). An organ’s diffusion properties are related to the amount of interstitial free water and tissue permeability. A prostatic neoplasm generally has more restricted diffusion due to higher cell densities and cancer cell membrane characteristics [17]. MRSI provides metabolic information by determining the chemical concentration of various compounds and metabolites in the prostate architecture. In prostate cancer, the choline-to-citrate ratio is generally used to identify lesions that are at high risk of representing prostate cancer [18].
Each of the MRI techniques offers distinct advantages and limitations, so the ideal use of MRI in prostate cancer is to use a combined multiparametric MRI (mpMRI) approach, where information from each technique is used to complement the information gained from the others. Modern software algorithms are able to register and fuse the information from different techniques to allow functional and anatomic colocalization. Typical mpMRI sequences include T1- and T2-weighted images combined with functional imaging techniques. There is increasing use of mpMRI in the diagnosis and staging of prostate cancer, but no formal recommendations of guidelines are available [19]. Efficient detection of prostate cancer is improved with performing targeted biopsies with MRI guidance, and sampling of the most abnormal area on a prostate MRI is likely to yield a positive diagnosis of prostate cancer [20, 21]. Accurate delineation of the prostate cancer lesions improves patient staging and also informs important brachytherapy treatment decisions regarding the need for supplemental external beam radiation therapy or androgen deprivation therapy.
Intraoperative Imaging
TRUS has been the mainstay imaging modality for intraoperative brachytherapy imaging and treatment planning due to its portability, ease of use, low cost, and safety. Suboptimal source placement, in the past, was frequently a result of poor TRUS imaging during the procedure, a problem that is being mitigated with improvements in ultrasound technology and the ability to fuse ultrasound images with other modalities including CT and ultrasound.
Several institutions are exploring the concept of focal boost treatment strategies, where the whole prostate gland is treated but areas of dominant tumor are given a boost dose. In local failures after primary radiation therapy, there is a body of evidence that suggests the local recurrence happens at the dominant tumor locations [22, 23]. A focal brachytherapy boost could improve the therapeutic ratio of brachytherapy treatments by dose-escalating areas of bulky tumor while still allowing minimal increased dose to adjacent normal tissues.
Focal Brachytherapy
Due to the multifocal nature of prostate cancer, the use of any definitive radiotherapy has included the whole prostate gland as a target volume. With the advent of PSA screening and advanced imaging techniques, outlined above, that improve staging and finely hone risk group stratification, whole-gland treatment may represent overtreatment of low-grade, well-defined disease. Therefore, the concept of focal brachytherapy is being explored for patients with unifocal dominant lesions [24] with the assumption that the dominant lesion is responsible for total tumor volume, risk of recurrence, and Gleason score. In addition to brachytherapy, a variety of other focal ablative approaches are being explored, including photodynamic therapy, high-intensity ultrasound, cryotherapy, and thermal laser ablation.
Postoperative Dosimetry
A successful prostate implant demonstrates adequate coverage of the prostate gland and limitation of dose to adjacent normal tissues including the rectum, bladder, urethra, and neurovascular bundles. As detailed above, the ABS recommends post-implant dosimetry for all patients undergoing prostate brachytherapy. In this process, implanted sources are localized and correlated with prostatic anatomy to calculate the dosimetric parameters of interest. This process allows the radiation oncologist to correct systematic deficiencies in overall implant technique while identifying patients who are at risk for local failure or toxicity development. It is acknowledged that given the real-time nature of brachytherapy, there will be spatial variation in source placement from the intraoperative plan to the post-plan, and therefore a range of post-plan variabilities is to be anticipated.
The first published report of CT for post-implant dosimetry was in the 1990s, which allowed for the representation of organ and dose distribution together in spatial registration [25]. In contemporary practice, CT is recommended to evaluate post-implant dosimetry and is used in the vast majority of cases [26]. While CT is inexpensive and widely available and allows for easy identification for implant prostate seeds, it is not optimal for identification of the periphery of the prostate, particularly at the apex and base of the gland. Several authors have reported large uncertainties in prostate contours in post-implant CT scans [27, 28]. Additionally, the CT volume is generally different from the intraoperative volume and remains a confounding issue in the analysis and reporting of dosimetric outcomes [29].
Techniques
Brachytherapy of the prostate can be performed with a high-dose rate technique or a low-dose rate technique. LDR brachytherapy is an outpatient, minimally invasive, interventional procedure with a rapid recovery and ability to return to normal activities in a short period of time. In LDR prostate brachytherapy, permanent radioactive sources are placed in the prostate gland which deliver a certain amount of radiation before becoming nonradioactive. HDR brachytherapy is similar but may require a short inpatient stay and is performed with a temporary insertion of a high-dose radioactive source. Modern PPB techniques using ultrasound guidance and template-based placement of applicator needles were developed over 25 years ago and are widely still in use [30, 31].
Various interventional approaches regarding brachytherapy have been published with small differences in technique. There is not a uniformly accepted best defined approach nor a medicolegally defined standard for LDR or HDR brachytherapy. The standard approach for LDR seed implantation is to utilize a transperineal approach under TRUS guidance. Template guidance is used for needle placement. Figure 14.2 demonstrated the TRUS-guided transperineal approach with needle template in place. Some institutions use a preplanned approach, where a preoperative TRUS study is done to identify applicator needle and seed placement which is then later recreated in actual operative procedure. However, many centers are moving to an intraoperative approach, where needles are placed real time in the operative room with source placement finalized during the procedure after applicator needles have been placed. The intraoperative approach allows for a dynamic, real-time application of brachytherapy sources and obviates the challenging requirement to recreate the same patient and applicator anatomy used in the preplanning session.
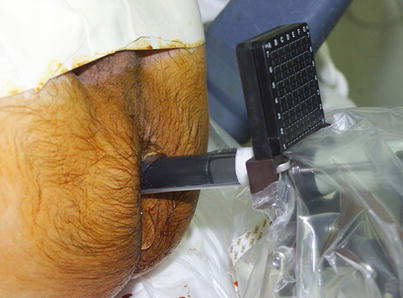
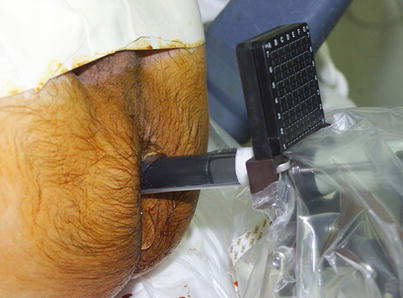
Fig. 14.2
Transrectal ultrasound-guided approach with needle delivery template used for prostate brachytherapy
The TRUS system used for implantation should be capable biplanar imaging and provide high-resolution ultrasonic frequencies between 5 and 12 MHz [5]. The system should be capable of displaying an electronic grid that is calibrated and synchronized with the transperineal template docked to the ultrasound probe. Dedicated FDA-approved brachytherapy planning software should be used for the planning portion of the brachytherapy procedure. There have been multiple reports for the use of intraoperative fluoroscopy to complement ultrasound imaging [32] and for intraoperative dose computation [33], although this fluoroscopy is neither standard nor necessary for LDR interstitial brachytherapy. Similarly, there have been reports of intraoperative cone beam CT imaging (CBCT) for interventional guidance and for dose planning purposes.
During the procedure itself, the patient is anesthetized and placed in the dorsal lithotomy position. The perineum is sterilized and draped and the testicles are retracted away from the perineum. The ultrasound probe is placed into the rectum transanally, and adequate visualization of the prostate in the axial and longitudinal axes is confirmed. Applicator needles are placed into the prostate to facilitate delivery of the radioactive sources, as demonstrated in Fig. 14.3. Once applicator needles are placed, imaging is captured in the treatment planning system (Fig. 14.4), and a spatial reconstruction of the prostate, urethra, rectum, and other adjacent organs of risk is performed. A seed-loading pattern is either then generated with real-time planning or attempts are made to recreate the spatial arrangement of a preplan. Multiple effective strategies to source placement have been reported, including the use of a Mick applicator to place loose seeds individually [34] (Fig. 14.5), the use of preloaded needles where all the sources in a given needle are prearranged and deployed in a single motion [35], and the use of afterloading techniques [36]. During placement of sources, a “modified peripheral” loading pattern is generally recommended, in which most seeds are placed at the periphery. The radioactive sources can be “loose” and placed one at a time or can be “stranded,” where all the seeds in a given needle are linked via a bioresorbable polymer. The potential advantages and disadvantages of each approach have been reported, especially for loose seed migration with loose seed placement [37–46], but all approaches have been found to be safe and effective as long as appropriate dosimetric end points are ultimately met.
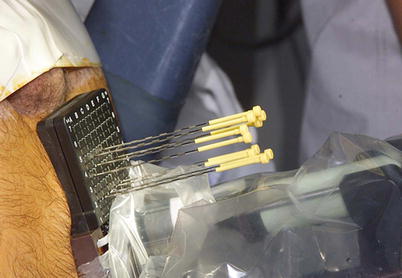
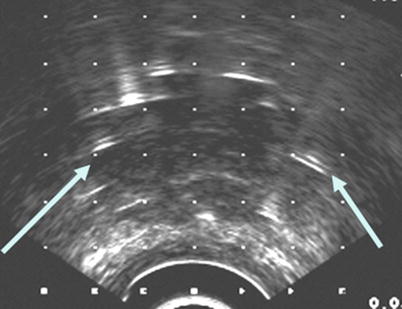
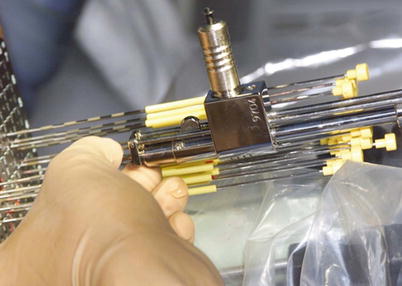
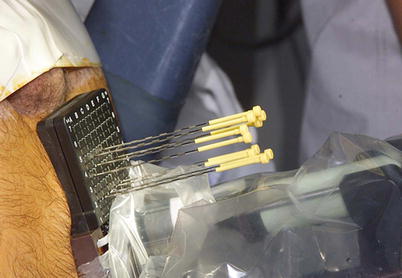
Fig. 14.3
Interstitial needles used for low-dose rate brachytherapy seed placement
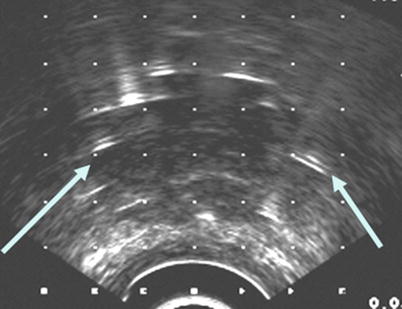
Fig. 14.4
Interstitial delivery needles on transrectal ultrasound-guided image
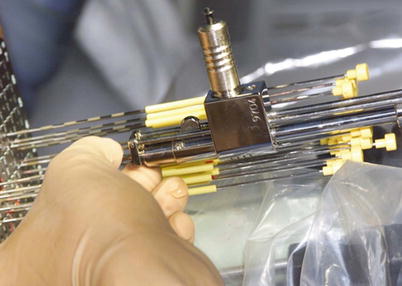
Fig. 14.5
Mick applicator with seed cartridge for delivery of loose, low-dose rate brachytherapy seeds
Generally recommended dose prescriptions for the planning target volume have been published by the ABS and are delineated in Table 14.3 [5]. Figure 14.6 demonstrates representative isodose lines from the intraoperative treatment planning system. In general, compliance with the American Association of Physics and Medicine Task Group’s 43 and 137 reports is highly recommended, which give specific guidelines for treatment planning and dosimetric evaluation. There have been no prospective randomized trials to identify the optional dose and dosimetric evaluation of a prostate implant. Stock first reported the concept of D90 (the isodose encompassing 90% of the prostate) and its relationship to treatment outcome [47]. Numerous authors have also reported the predictive value of the V100, or the percentage volume of the prostate receiving 100 % of the prescribed dose [48]. To minimize the risk of urethral toxicity, it is recommended that significant portions of the urethra do not receive greater that 150 % of the prescribed dose [49]. The rectal volume receiving the prescription dose should be <1–2 cc [50
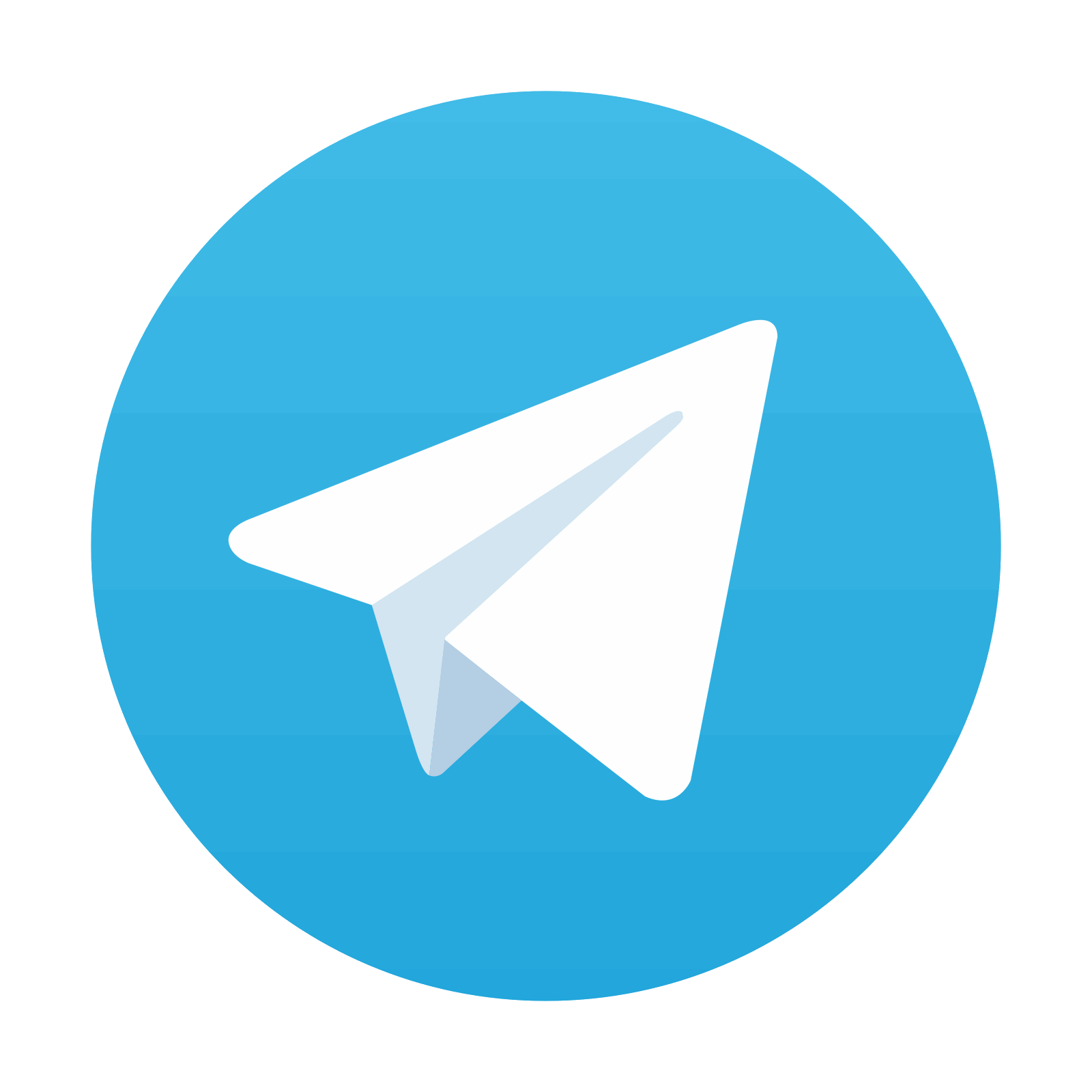
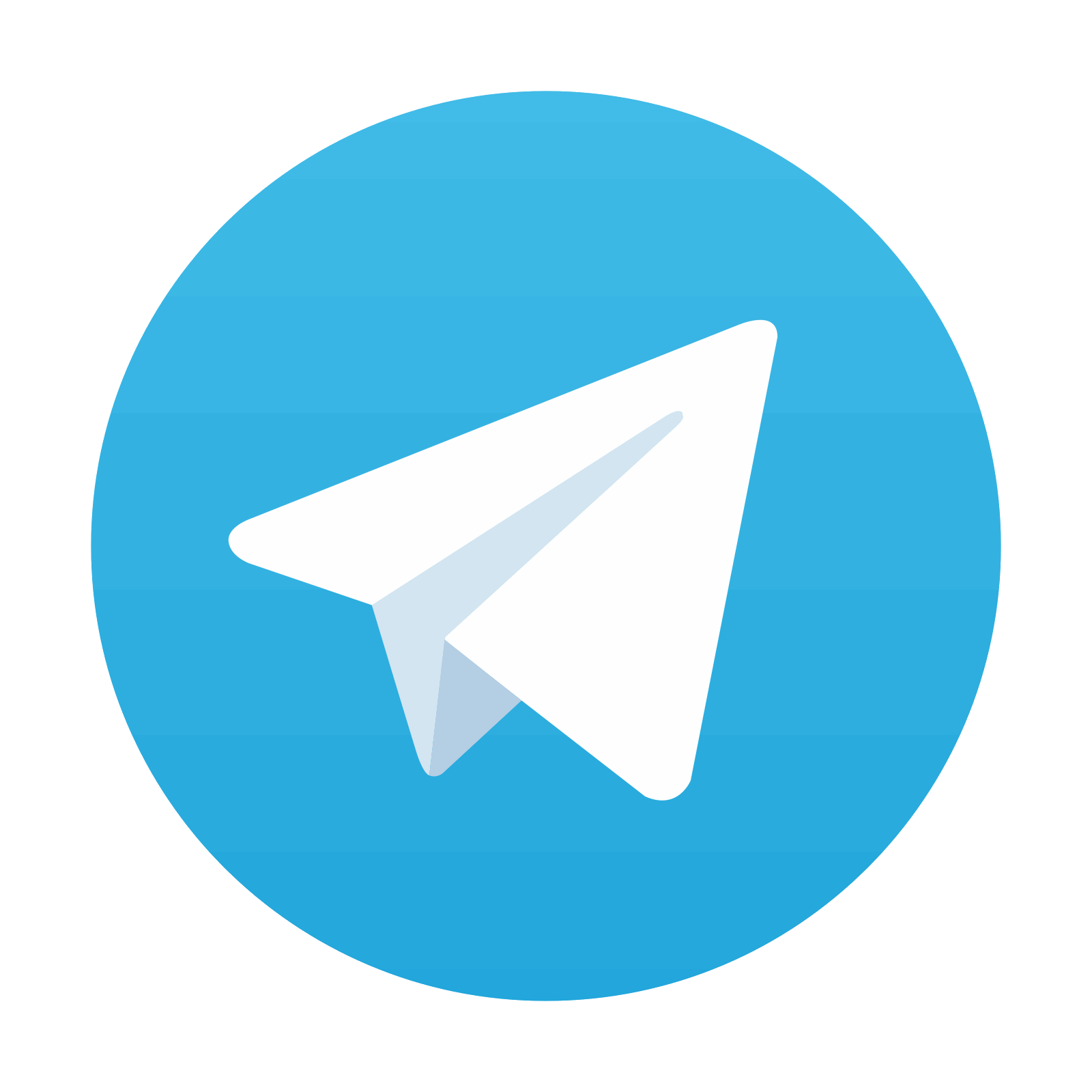
Stay updated, free articles. Join our Telegram channel

Full access? Get Clinical Tree
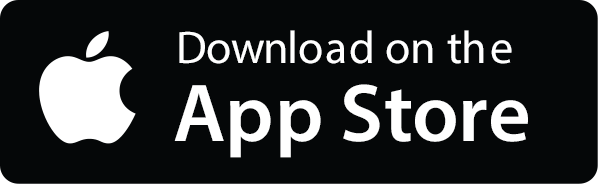
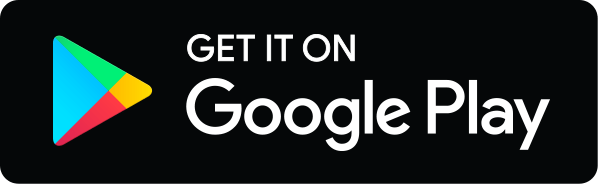