© Springer International Publishing AG 2017
Pietro Ruggieri, Andrea Angelini, Daniel Vanel and Piero Picci (eds.)Tumors of the Sacrum10.1007/978-3-319-51202-0_2727. Radiation Therapy for Primary Malignant Sacral Tumors
(1)
Section of Orthopaedic Oncology, Department of Orthopaedic Surgery, Massachusetts General Hospital, Boston, MA, USA
27.1 Introduction
Radiation therapy continues to evolve and play an ever larger role in the management of primary tumors of the sacrum. Radiation therapy has become much more precise, and the principle advances in radiation have been in the sparing of normal tissues around tumors. Highly conformal photon radiation, carbon ion radiation, and proton radiation are all the so-called high precision radiation delivery methods. This relative sparing allows much higher doses to be delivered to the tumor rendering previously “radiation-resistant” tumors susceptible to radiation effects. Chondrosarcoma and chordoma have the reputation for being radiation resistant and both are commonly found in the sacrum. However, the literature continues to expand with reports of good local control with radiation as an adjuvant to surgery and, in some cases, as stand-alone treatment. However, the side effects of radiation increase as the dose increases. Even though there is relative sparing of normal tissue, there continues to be off-target effects and the true side effect profile for high precision radiation therapy is not known.
27.1.1 History
Radiation as method of treatment for cancer has been used since Roentgen first introduced X-rays as a diagnostic tool. Initially, radiation as a therapy was delivered in one treatment session similar to how it was used as a diagnostic tool. However, short-term complications such as skin slough tempered the initial enthusiasm for radiation as a therapy. At that time, the most immediate outcomes were that of complications and the concept of radiation-resistant tumors was not yet discussed. In order to avoid short-term complications, radiation oncologists started delivering radiation in divided doses to help prevent local complications. The divided dose or fraction is allowed for the normal structures to recover from the off-target effects of radiation delivery. The downside of fractionating radiation is that the tumor cells are also allowed to recover. Radiation oncologists began developing other methods of protecting normal structures such as changing the angle which radiation entered the body. This allowed some of the off-target effects of radiation to be shared by other normal structures rather than delivering to the same structures as it had been done initially. Even with these changes the total dose of radiation was limited by the off-target affects seen in sensitive structures such as the lining of the alimentary track or the skin. For axial tumors, the spinal cord was a rate-limiting structure owing to the known toxicity manifested in the spinal cord after 50 Gy of radiation. Most studies using radiation for primary bone tumors did not exceed doses of 40 Gy, and this proved to be an ineffective dose for most primary malignant bone tumors, which is why radiation has the reputation for being an ineffective salvage in cases where surgery has failed or thought impractical to apply. The so-called radiation-resistant tumors are actually resistant only at the doses historically delivered since all cells are susceptible to radiation when high enough doses are used. The principle advance in radiation therapy has been minimizing the off-target effects on normal structures; thereby allowing the radiation oncologist to increase the doses of radiation used.
27.1.2 Photon Radiation
Photon radiation is the most common type of radiation used to treat cancer, and it is the same type of radiation used in diagnostic X-rays. Photon radiation is most commonly produced by a linear accelerator in which alternating electromagnetic waves are used to accelerate elections towards a target. Conventional radiation therapy using photons was introduced previously, and it is far and away the most common type of radiation used today to palliate cancer. Many advances have been made to conventional radiation that enable the more precise delivery of the electrons. One means by which precision has been improved is by the use of real-time three-dimensional imaging such as en suite computed tomography. This allows for highly accurate depictions of the target. The patient and the target (tumor) are necessarily held in position and motion is limited to the greatest degree possible by using various harnesses and positioners. Most modern radiation suites place patients on a table, which can be moved in six plains in order to maximize the effectiveness of having imaging capacity. These methods help to localize the target and hold the target into position. The beam of radiation is also rotated about a gantry, again to help minimize off-target effects of radiation.
The beam of radiation can be further modified in order to maximize on target and minimize off-target affects. The so-called intensity-modulated radiation therapy (IMRT) involves using smaller beams of radiation about the size of a number 2 pencil. The radiation oncologist can modulate the energy delivered in each beam to help protect normal structures. Computer software has greatly facilitated this method of treatment. In fact, IMRT uses what is known as reverse planning where the dose delivered to the tumor is planned first. The software then helps decide how to deliver the radiation while sparing normal structures by modulating the beams of radiation utilized. The net effect is to allow higher doses of radiation to be used because radiation deliver is much more precise with tolerances of 2–3 mm when the target can be fully immobilized [1].
27.1.3 Carbon Ion Radiation
Unlike photon radiation, carbon ion radiation relies on accelerating carbon to nearly 70% of the speed of light. The principle advantage of carbon ion therapy is that the amount of radiation delivered increases with depth into the patient and then drops off precipitously in what is known as a Bragg peak. This is in contrast to photon radiation in which higher entry doses are seen which gradually taper off until the radiation passes through the target. There is both a high entry dose and a relatively high exit dose with photon radiation, whereas there is a smaller entry dose and essentially no exit dose with carbon ion therapy. The location of the Bragg peak can be adjusted based on where its effect is most needed. The lack of an exit dose and a smaller entry dose allow the total dose to the target to be increased when compared to conventional photon radiation. The principle disadvantage of carbon ion therapy is that a specialized facility is required for its delivery. The costs of these facilities are high. Carbon ion facilities exist in Japan and several are in use or under development in Europe [1].
27.1.4 Proton Radiation
Proton radiation relies on the acceleration of protons and, similar to carbon ion, the radiation dose has a Bragg peak pattern rather than a gradual drop off with depth seen with conventional photon radiation. It is the Bragg peak that allows for higher doses of radiation to be used as there are no exit doses which limits the off-target affects of radiation. Similar to carbon ion facilities, proton accelerators are costly to build but there are now over 20 facilities in the United States either in use or in development [1].
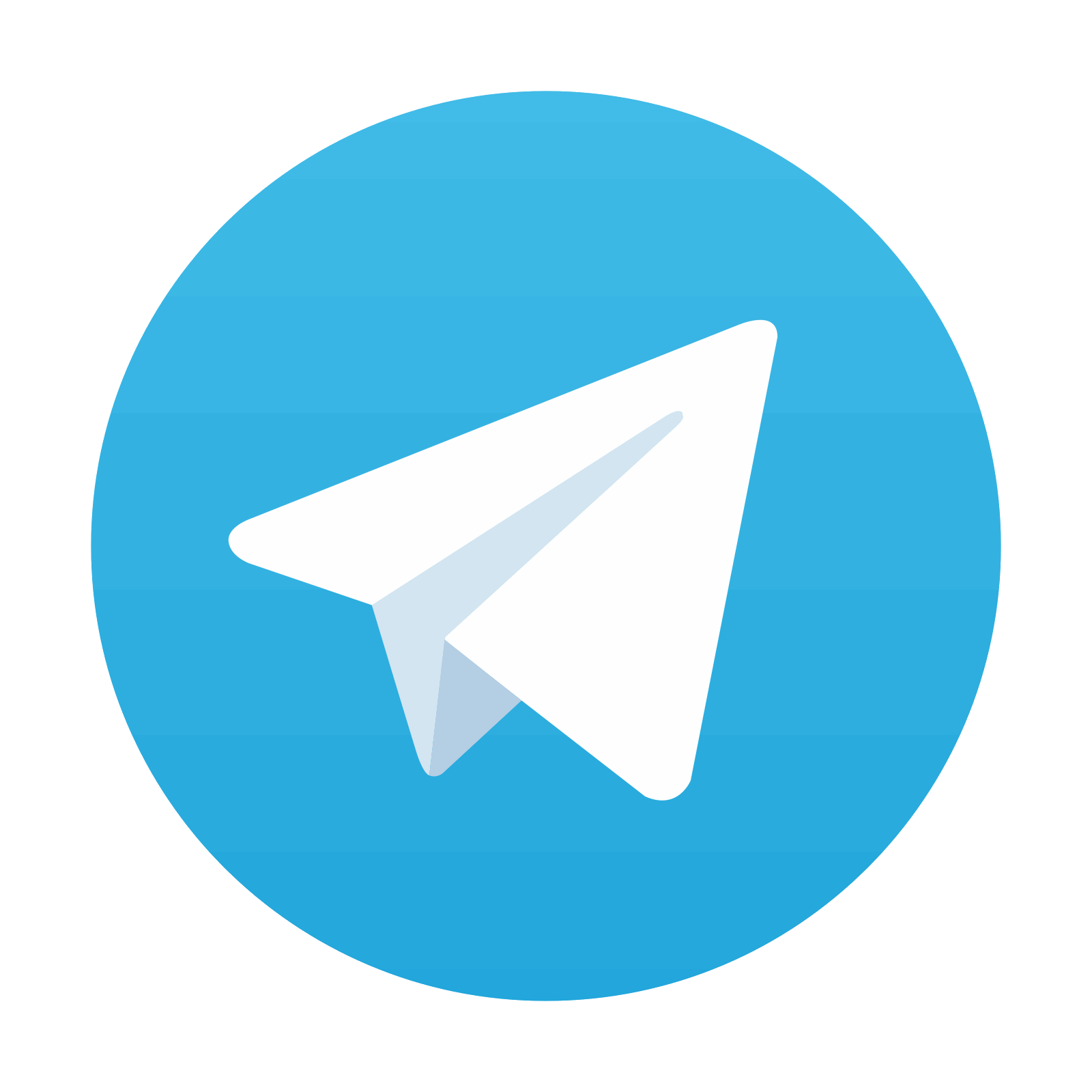
Stay updated, free articles. Join our Telegram channel

Full access? Get Clinical Tree
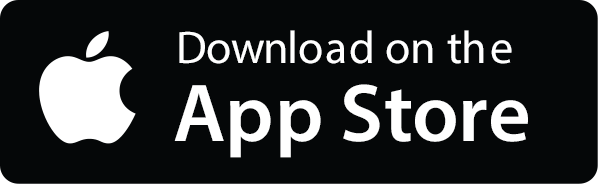
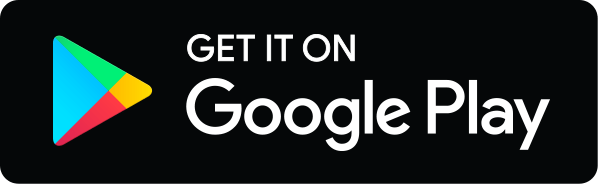