Radiologic Evaluation of Trauma
Radiologic Imaging Modalities
The radiologic modalities used in analyzing an injury to the musculoskeletal system are as follows:
Conventional radiography, including routine views (specific for various body parts), special views, and stress views
Digital radiography, including digital subtraction arthrography (DSa) and angiography (DSA)
Fluoroscopy, alone or combined with videotaping
Computed tomography (CT)
Arthrography, tenography, and bursography
Myelography and diskography
Angiography (arteriography and venography)
Scintigraphy (radionuclide bone scan)
Magnetic resonance imaging (MRI).
Radiography and Fluoroscopy
In most instances, radiographs obtained in two orthogonal projections, usually the anteroposterior and lateral, at 90 degrees to each other are sufficient (Fig. 4.1). Occasionally, oblique and special views are necessary, particularly in evaluating fractures of complex structures such as the pelvis, elbow, wrist, and ankle (Figs. 4.2 and 4.3). Stress views are important in evaluating ligamentous tears and joint stability (Fig. 4.4).
Fluoroscopy and videotaping are useful in evaluating the kinematics of joints and fragments. It is also valuable in monitoring the progress of healing.
Computed Tomography
CT is essential in the evaluation of complex fractures, particularly of the spine, pelvis, and scapula, although this modality is useful in the assessment of any fracture near or extending into the joint (Figs. 4.5,4.6,4.7; see also Figs. 7.13B and 7.14B). The advantage of CT over conventional radiography is its ability to provide excellent contrast resolution and accurate measurement of the tissue attenuation coefficient. The use of sagittal, coronal, and multiplanar reformation (see Figs. 9.29B,C and 9.31A,B) as well as reconstruction to create the 3D CT images (Fig. 4.8; see also Figs. 2.8,2.9,2.10) provides an added advantage over other imaging modalities.
Scintigraphy
Radionuclide bone scanning can detect occult fractures or fractures too subtle to be seen on conventional radiographs (Fig. 4.9). This technique is also effective in the differentiation of tibial stress fractures from shin splints. Scintigraphy occasionally aids in making a differential diagnosis of old-versus-recent fractures and in detecting such complications as early-stage osteonecrosis. However, bone scans seldom provide new information about the status of fracture healing and, in particular, static bone scans fail to separate normally healing fractures from delayed healing fractures or those that result in nonunion. Also, a bone scan cannot indicate the point at which clinical union is established. Scintigraphy is, however, helpful in distinguishing noninfected fractures from infected ones. With osteomyelitis, scanning, using gallium citrate (67Ga) and indium-labeled white blood cells (111In), demonstrates a significant increase in the uptake of the tracer. Because 67Ga is also actively taken up at the site of a normally healing fracture, but significantly less than that encountered with 99mTc (technetium) scanning agents, the combination of 67Ga and 99mTc methylene diphosphonate (MDP) has been suggested, using the ratio of uptake of 67Ga to 99mTc to determine whether the fracture is infected. The ratio of 67Ga to 99mTc MDP should be higher in infected fractures than in noninfected fractures. It is very difficult to differentiate pseudoarthrosis from infection at the fracture site. Standard 99mTc and 67Ga bone scans are not helpful, because both may be positive for both conditions. In these instances, 111In white blood cell scanning combined with 99mTc MDP scanning appears to be the best method for determining if a fractured or traumatized bone is infected. For more information regarding recent trials of evaluating infected fractures with new radionuclide agents including immunoglobulins, see Chapter 2.
Arthrography
Arthrography is still occasionally used in the evaluation of injuries to articular cartilage, menisci, joint capsules, tendons, and ligaments (Figs. 4.10 and 4.11), although, in general, it has been replaced by MRI and MR arthrography. Although virtually every joint can be injected with a contrast agent, the examination is most frequently performed in the knee, shoulder, ankle, and elbow articulations.
![]() FIGURE 4.8 Fracture of the acetabulum. 3D CT reconstructed image shows distinctive features of a fracture of the posterior wall of the right acetabulum (arrow). |
![]() FIGURE 4.10 Tear of the medial meniscus. In this patient, double-contrast arthrography of the knee shows a horizontal cleavage tear in the posterior horn of the medial meniscus (arrow). |
![]() FIGURE 4.15 Chondral defects. Axial proton density-weighted fat-saturated MRI of the knee demonstrates subtle defects in the articular cartilage of the right patella (arrows). |
Tenography and Bursography
As already stated in Chapter 2, these procedures at the present time are seldom performed, being replaced by MRI. Tenography used to be done to evaluate the integrity of a tendon, such as peroneus longus and brevis, tibialis anterior and posterior, and flexor digitorum longus. Bursography of the subacromial-subdeltoid bursae complex occasionally demonstrated a partial or full-thickness tear of the rotator cuff.
Myelography and Diskography
Angiography
Magnetic Resonance Imaging
MRI plays a leading role in the evaluation of trauma to bone, cartilage, and soft tissue. MRI evaluation of trauma to the knee, particularly abnormalities of the menisci and ligaments, has a high negative predictive value. MRI can be used to screen patients before surgery, so that unnecessary arthroscopies are avoided. MRI is probably the only imaging modality that can demonstrate so-called bone contusions (see Fig. 2.33). These abnormalities consist of posttraumatic marrow change resulting from a combination of hemorrhage, edema, and microtrabecular injury. Meniscal injuries, such as bucket-handle tears, tears of the free edge, and peripheral detachments, can be accurately diagnosed. Other subtle abnormalities of various structures and posttraumatic joint effusion can also be well visualized (Figs. 4.15 and 4.16). Similarly, the medial and lateral collateral ligaments, anterior and posterior cruciate ligaments, and tendons around the knee joint can be well demonstrated (see Figs. 9.13,9.14,9.15) and abnormalities of these structures can be diagnosed with high accuracy. In the shoulder, impingement syndrome and complete and incomplete rotator cuff tears may be effectively diagnosed most of the time (Fig. 4.17). Traumatic lesions of the tendons (such as biceps tendon rupture), traumatic joint effusions, and hematomas are easily diagnosed with MRI. Likewise, this modality is effective to diagnose a tear of the cartilaginous labrum. The changes of osteonecrosis at various sites, particularly in its early stage, may be detected by MRI when other modalities, such as conventional radiography and even radionuclide bone scan, may be normal. MRI of the ankle and foot has been used among others in diagnosing tendon ruptures and posttraumatic osteonecrosis of the talus. In the wrist and hand, MRI has been successfully used in the early diagnosis of posttraumatic osteonecrosis of the scaphoid and Kienböck disease. MRI is strongly advocated as the technique of choice in the evaluation of abnormalities of the triangular fibrocartilage complex, although arthrography, particularly in conjunction with digital imaging and CT, is also a very effective modality.
![]() FIGURE 4.18 A complete fracture. (A) The continuity of the bone (tibia) is disrupted and there is a narrow gap between the bone fragments. (B) A complete fracture of the femur in an adult patient. |
The greatest use of MRI is for evaluating trauma of the spine, the spinal cord, the thecal sac, and nerve roots, as well as for evaluating disk herniation (see Fig. 11.97). MRI is also useful in the evaluation of spinal ligament injuries. The demonstration of the relationship of vertebral fragments to the spinal cord with direct sagittal imaging is extremely helpful, particularly to evaluate injuries in the cervical and thoracic areas.
Fractures and Dislocations
Fractures and dislocations are among the most common traumatic conditions encountered by radiologists. By definition, a fracture is a complete disruption in the continuity of a bone (Fig. 4.18). If only some of the bony trabeculae are completely severed while others are bent or remain intact, the fracture is incomplete (Fig. 4.19). A dislocation is a complete disruption of a joint; articular surfaces are no longer in contact (Fig. 4.20). A subluxation, however, is a minor disruption of a joint in which some articular contact remains (Fig. 4.21). Proper radiologic evaluation of these conditions contributes greatly to successful treatment by the orthopedic surgeon.
In dealing with trauma, the radiologist has two main tasks:
Diagnosing and evaluating the type of fracture or dislocation
Monitoring the results of treatment and looking for possible complications
Diagnosis
The important radiographic principle in diagnosing skeletal trauma is to obtain at least two views of the bone involved, with each view including two joints adjacent to the injured bone (Fig. 4.22). In so doing, the radiologist eliminates the risk of missing an associated fracture, subluxation, and/or dislocation at a site remote from the apparent primary injury. In children, it is frequently necessary to obtain a radiograph of the normal, unaffected limb for comparison.
Radiographic Evaluation of Fractures
The complete radiographic evaluation of fractures should include the following elements: (a) the anatomic site and extent of a fracture (Fig. 4.23); (b) the type of fracture, whether it is incomplete, as seen predominantly in children, or complete (Fig. 4.24); (c) the alignment of the fragments with regard to displacement, angulation, rotation, foreshortening, or distraction (Fig. 4.25); (d) the direction of the fracture line in relation to the longitudinal axis of the bone (Fig. 4.26); (e) the presence of special features such as impaction, depression, or compression (Fig. 4.27); (f) the presence of associated abnormalities such as a fracture with concomitant dislocation or diastasis (Fig. 4.28); and (g) special types of fractures that may occur as the result of abnormal stress or secondary to pathologic processes in the bone (Fig. 4.29). The distinction between an open (or compound) fracture, one in which the fractured bone communicates with the outside environment through an
open wound, and a closed (or simple) fracture, one that does not produce an open wound in the skin, should preferably be made by clinical rather than radiographic examination.
open wound, and a closed (or simple) fracture, one that does not produce an open wound in the skin, should preferably be made by clinical rather than radiographic examination.
![]() FIGURE 4.22 Adjacent joints. The radiograph of a suspected fracture of the femoral shaft should include the hip and knee articulations. |
![]() FIGURE 4.23 Site and extent. Factors in the radiographic evaluation of a fracture: the anatomic site and extent. |
![]() FIGURE 4.24 Incomplete and complete fractures. Factors in the radiographic evaluation of a fracture: the type of fracture, incomplete or complete. |
![]() FIGURE 4.25 Alignment. Factors in the radiographic evaluation of a fracture: the alignment of the fragments. |
![]() FIGURE 4.26 Direction. Factors in the radiographic evaluation of a fracture: the direction of the fracture line. |
![]() FIGURE 4.27 Special features. Factors in the radiographic evaluation of a fracture: special features. |
![]() FIGURE 4.28 Associated abnormalities. Factors in the radiographic evaluation of a fracture: associated abnormalities. |
![]() FIGURE 4.29 Special types. Factors in the radiographic evaluation of a fracture: special types of fractures. |
In children, the radiographic evaluation of fractures, particularly of the ends of tubular bones, should also take into consideration the involvement of the growth plate (physis). Localization of the fracture line has implications with respect to the mechanism of injury and possible complications. A useful classification of injuries affecting the physis, metaphysis, epiphysis, or all of these structures has been proposed by Salter and Harris (types I to V) and has been expanded by Rang (type VI) and Ogden (types VII to IX) to include four additional types of fractures (Fig. 4.30). Although the injuries described by Rang and Ogden do not directly involve the growth plate, the sequelae of such trauma affect the physis in the same way as the direct injuries described by Salter and Harris. In type VI, which involves only the peripheral region of the growth plate, the injury may not always be associated with a fracture. It may result from a localized contusion, trauma-induced infection, or severe burn. Type VII injury consists of a purely transepiphyseal fracture that, if the epiphysis is not completely ossified, may not even be detectable on the conventional radiograph. Type VIII injury involving the metaphyseal region may be complicated by damage to the blood vessels supplying the growth plate, and in type IX, an injury to the periosteum may interfere with the membranous mechanism of bone formation. All such trauma, but particularly types IV and V (see Fig. 4.72), may lead to growth disturbance with consequent limb-length discrepancy.
Indirect Signs as Diagnostic Clues
Although the diagnosis of most fractures can be made from conventional radiographs, some subtle, nondisplaced, and hairline fractures may not be apparent at the time of injury. In such instances, certain indirect signs of fracture provide useful diagnostic clues.
![]() FIGURE 4.30 Classification of the growth plate injuries. The Salter-Harris classification of injuries involving the growth plate (physis) together with Rang and Ogden additions. |
Soft-Tissue Swelling. Skeletal trauma is always associated with an injury to the soft tissues, and in almost all cases of acute fracture, there is some radiographic evidence of soft-tissue swelling at the fracture site (Fig. 4.31A). The absence of soft-tissue swelling, however, virtually excludes the possibility of an acute fracture (Fig. 4.31B).
Obliteration or Displacement of Fat Stripes. Subtle fractures, particularly in the distal radius, carpal scaphoid, trapezium, and base of the first metacarpal, result in obliteration or displacement of fascial planes. On the lateral view of the wrist, one can detect a radiolucent stripe representing a collection of fat between the pronator quadratus (quadratipronator) and the tendons of the flexor digitorum profundus. A fracture of the distal radius results in a change in the appearance of the pronator quadratus fat stripe, which may be anteriorly (volarly) displaced, blurred, or obliterated (MacEwan sign) (Fig. 4.32).
Terry and Ramin have pointed out the usefulness of recognizing the scaphoid fat stripe, which is usually visible as a thin radiolucent line paralleling the lateral surface of the scaphoid bone between the radial collateral ligament and the synovial sheath of the abductor pollicis longus and the extensor pollicis brevis. In most fractures of the carpal scaphoid, radial styloid, trapezium, or base of the first metacarpal, the scaphoid fat stripe is obliterated or displaced. This finding is most apparent on the dorsovolar view of the wrist (Fig. 4.33).
Periosteal and Endosteal Reaction. The fracture line may not be visible, but the periosteal or endosteal response may be the first radiographic sign of a fracture (Fig. 4.34).
Joint Effusion. This finding, which results in the radiographic appearance of the fat-pad sign, is particularly useful in diagnosing elbow injuries. The posterior (dorsal) fat pad lies deep in the olecranon fossa and is not visible in the lateral projection. The anterior (ventral) fat pad
occupies the shallower anterior coronoid and radial fossae and is usually seen as a flat radiolucent strip ventrad to the anterior cortex of the humerus. Distention of the articular capsule by synovial or hemorrhagic fluid causes the posterior fat pad to become visible and also displaces the anterior fat pad, yielding the fat-pad sign (Fig. 4.35). When there is a history of elbow trauma and the fat-pad sign is positive, there is usually an associated fracture and every effort should be made to demonstrate it. Even if the fracture line is not demonstrated on multiple films, the patient should be treated for fracture.
occupies the shallower anterior coronoid and radial fossae and is usually seen as a flat radiolucent strip ventrad to the anterior cortex of the humerus. Distention of the articular capsule by synovial or hemorrhagic fluid causes the posterior fat pad to become visible and also displaces the anterior fat pad, yielding the fat-pad sign (Fig. 4.35). When there is a history of elbow trauma and the fat-pad sign is positive, there is usually an associated fracture and every effort should be made to demonstrate it. Even if the fracture line is not demonstrated on multiple films, the patient should be treated for fracture.
Intracapsular Fat-Fluid Level. If a fracture involves the articular end of a bone (particularly a long bone such as the tibia, humerus, or femur), blood and bone-marrow fat enter the joint (lipohemarthrosis) and produce a characteristic layering of these two substances on the radiograph: the fat-blood interface, or FBI sign (Fig. 4.36). A CT or MRI study can also demonstrate this phenomenon (Figs. 4.37 and 4.38). When the fracture line cannot be demonstrated, the diagnosis should be made on the strength of this sign alone.
Double Cortical Line. This finding indicates a subtle but depressed fracture. The actual fracture line may not be apparent, but the double contour of the cortex reflects impaction (Fig. 4.39).
Buckling of the Cortex. Known as the torus fracture, this may be the only sign of a tubular bone fracture in children (Fig. 4.40). This finding is sometimes identified more easily on the lateral than on the frontal projection.
Irregular Metaphyseal Corners. This sign, which is secondary to small avulsion fractures of the metaphysis, indicates a subtle injury to the bone caused by a rapid rotary force exerted on the ligaments’ insertion. As a result, small fragments of bone are separated from the metaphysis. These corner fractures are often present in infants and children who sustain skeletal trauma, and they should be looked for particularly if battered child syndrome, also known as “shaken baby syndrome” or parent-infant trauma syndrome (PITS), is suspected (Fig. 4.41).
Radiographic Evaluation of Dislocations
Dislocations are more obvious than fractures on conventional radiographs and, consequently, are more easily diagnosed (Fig. 4.42). Some display such a characteristic appearance on frontal projection (anteroposterior view) that this single examination suffices (Fig. 4.42C). However, the same principle of obtaining at least two projections oriented at 90 degrees to each other should apply. Supplemental radiographs are occasionally necessary, and in some instances, CT is required for the exact evaluation of a dislocation.
Monitoring the Results of Treatment
Radiography plays the leading role in monitoring the progress of fracture healing and in detecting any posttraumatic complications. Follow-up radiographs should be taken at regular intervals to evaluate the stage and possible associated complications of fracture healing and other complications that may follow a fracture or dislocation. If radiographs are ambiguous in this respect, CT is the next technique to apply.
![]() FIGURE 4.37 FBI sign on CT. Axial CT section through the knee joint shows an FBI sign in a patient with tibial plateau fracture (not seen on this image). |
Fracture Healing and Complications
The healing of a fracture can be divided into three phases: inflammatory (reactive), reparative, and remodeling. The inflammatory phase is characterized by vasodilatation, serum exudation, and infiltration by inflammatory cells. It lasts about two to seven days. The reparative phase is characterized by the formation of periosteal and endosteal (medullary) calluses by the periosteal and bone marrow osteoblasts. Mesenchymal cell proliferation and differentiation are accompanied by intense vascular proliferation. The resulting osteoblasts produce collagen at a high rate. This phase lasts about a month. The remodeling phase is characterized by both modeling and remodeling at the site of a fracture to restore the original contours of the bone and its optimal internal structure. The endosteal and periosteal calluses are removed, and the woven immature bone is replaced by a secondary lamellar (cortical or trabecular) bone. If the fracture, particularly in the growing skeleton, has healed with incorrect angulation (malunion), this may be corrected by selectively removing bone from the convex side of the cortex by the process of osteoclastic resorption and adding bone to the concave side by the process of osteoblastic apposition. This phase may last from about three months to one year, or even longer.
Fracture healing depends on many factors: the patient’s age, the site and type of fracture, the position of the fragments, the status of the blood
supply, the quality of immobilization or fixation, and the presence or absence of associated abnormalities such as infection or osteonecrosis (Table 4.1). An average healing time of some fractures is depicted in Table 4.2. Most fractures heal by some combination of endosteal and periosteal callus. Provided that blood supply is adequate, undisplaced fractures and anatomically reduced fractures immobilized with adequate compression heal by primary union. In this type of healing, the fracture line becomes obliterated by endosteal (internal) callus. Displaced fractures, that is, those that are not anatomically aligned or with a gap between fragments, heal by secondary union. This type of healing is achieved mainly by excessive periosteal (external) callus, which undergoes full ossification through the stages of granulation tissue, fibrous tissue, fibrocartilage, woven bone, and compact bone. For the radiologist evaluating follow-up radiographs, the primary indication of bone repair is radiographic evidence of periosteal (external) and endosteal (internal) callus formation (Fig. 4.43). This process, however, may not be radiographically apparent in the early stage of healing. Periosteal response may not be visible on radiographs at sites where there is an anatomic lack of periosteum, for example, in the intracapsular portion of the femoral neck. Likewise, radiographs may not demonstrate endosteal callus formation, because the callus contains only fibrous tissue and cartilage, which are radiolucent. At this early stage of healing, a fracture may be clinically united, that is, show no evidence of motion under stress, yet radiographically, the radiolucent band between the fragments may persist (Fig. 4.44A). As the primary temporarily radiolucent callus is gradually converted by the process of endochondral ossification to more mature lamellar bone, it is seen on the film as a dense bridge (Fig. 4.44B). This constitutes radiographic union.
supply, the quality of immobilization or fixation, and the presence or absence of associated abnormalities such as infection or osteonecrosis (Table 4.1). An average healing time of some fractures is depicted in Table 4.2. Most fractures heal by some combination of endosteal and periosteal callus. Provided that blood supply is adequate, undisplaced fractures and anatomically reduced fractures immobilized with adequate compression heal by primary union. In this type of healing, the fracture line becomes obliterated by endosteal (internal) callus. Displaced fractures, that is, those that are not anatomically aligned or with a gap between fragments, heal by secondary union. This type of healing is achieved mainly by excessive periosteal (external) callus, which undergoes full ossification through the stages of granulation tissue, fibrous tissue, fibrocartilage, woven bone, and compact bone. For the radiologist evaluating follow-up radiographs, the primary indication of bone repair is radiographic evidence of periosteal (external) and endosteal (internal) callus formation (Fig. 4.43). This process, however, may not be radiographically apparent in the early stage of healing. Periosteal response may not be visible on radiographs at sites where there is an anatomic lack of periosteum, for example, in the intracapsular portion of the femoral neck. Likewise, radiographs may not demonstrate endosteal callus formation, because the callus contains only fibrous tissue and cartilage, which are radiolucent. At this early stage of healing, a fracture may be clinically united, that is, show no evidence of motion under stress, yet radiographically, the radiolucent band between the fragments may persist (Fig. 4.44A). As the primary temporarily radiolucent callus is gradually converted by the process of endochondral ossification to more mature lamellar bone, it is seen on the film as a dense bridge (Fig. 4.44B). This constitutes radiographic union.
TABLE 4.1 Factors Influencing Fracture Healing | ||||||||||||||||||||||||
---|---|---|---|---|---|---|---|---|---|---|---|---|---|---|---|---|---|---|---|---|---|---|---|---|
|
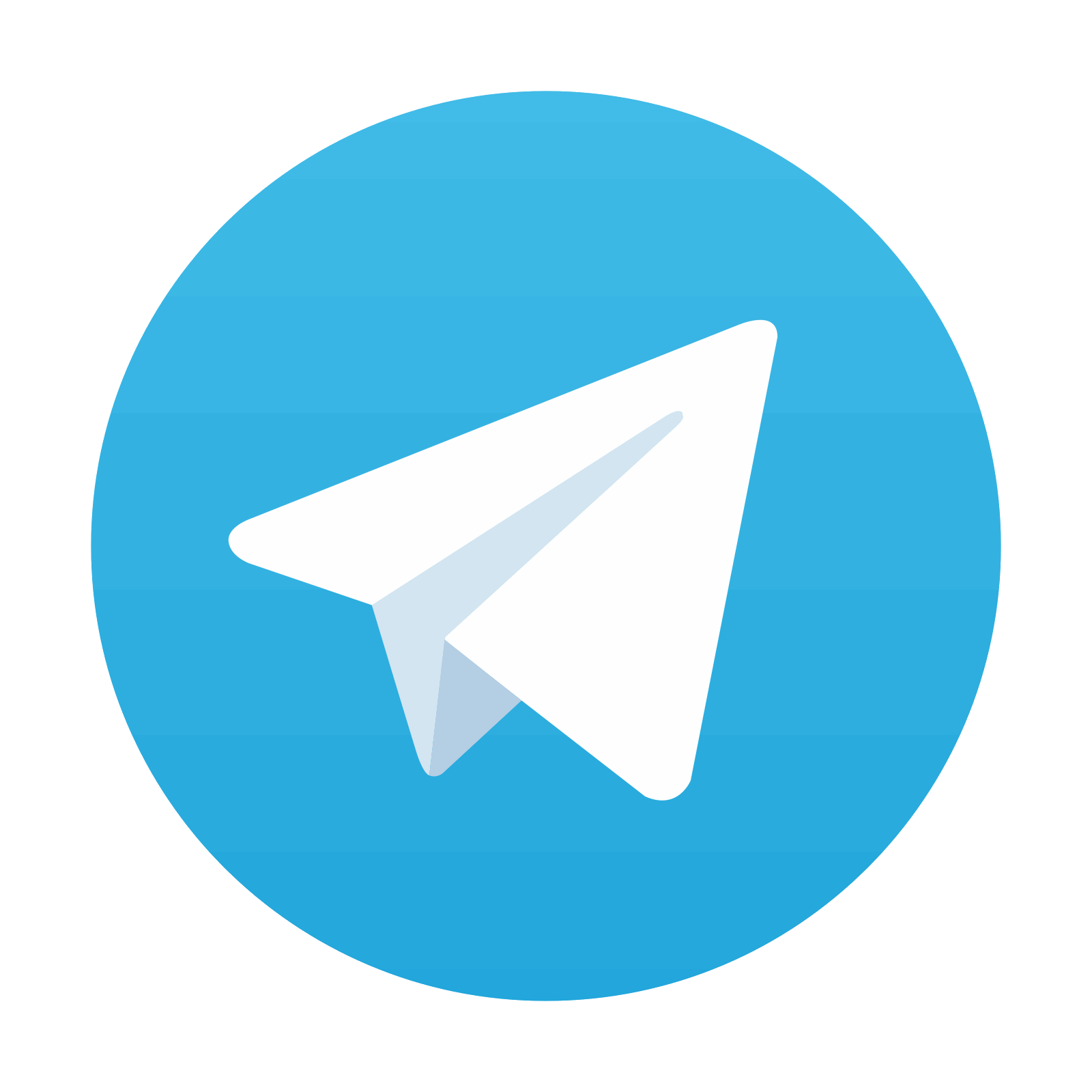
Stay updated, free articles. Join our Telegram channel

Full access? Get Clinical Tree
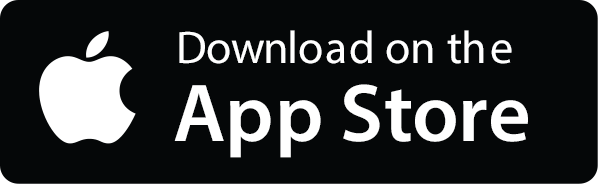
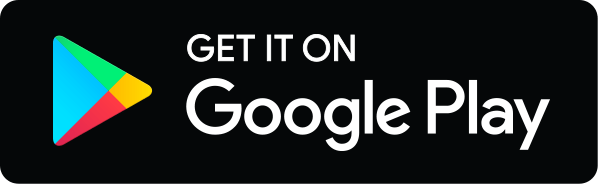
