An unstable atom that undergoes radioactive decay in order to achieve stability is known as a radionuclide. The radiation these atoms emit can sometimes be used in medical imaging and therapy. Agents approved for such uses in humans that incorporate radioactive molecules are referred to as radiopharmaceuticals. Radiopharmaceuticals can portray physiology, biochemistry, or pathology in the body without causing any significant physiological effect. They are also referred to as “radiotracers” because they are given in subpharmacological doses that “trace” a particular physiological or pathological process in the body. This chapter presents general principles regarding clinically important radionuclides and radiopharmaceuticals, their production, radiolabeling, and quality assurance. Some terms related to radioactive imaging and therapy agents are defined in Box 4.1 .
Radionuclide: Unstable isotope of an element that transitions to greater stability through radioactive decay.
Radiopharmaceutical: FDA-approved radioactive/radiolabeled agent (i.e., drug) for imaging or therapy.
Activity: The rate of decay; expressed as curies (3.7 × 10 10 decays per second) or in metric units as becquerels (1 decay per second, 1 mCi = 37 MBq).
Half-life (or <SPAN role=presentation tabIndex=0 id=MathJax-Element-1-Frame class=MathJax style="POSITION: relative" data-mathml='T12′>T12T12
T 1 2
): The time required for half the radioactive atoms in a sample to have decayed.
Equilibrium: Steady state or constant relationship that develops between a contained radioactive parent/daughter pair when the parent has a longer half-life than the daughter. Used for radionuclide production in a generator.
Carrier-free: Radiopharmaceutical free of contamination by other isotopes (stable or radioactive) of the same element. This is not to be confused with carrier molecule.
Carrier molecule: A chosen substance radiolabeled to allow evaluation or treatment of a particular physiologic parameter or cellular function, allowing or improving properties such as localization, accumulation, and/or background clearance.
Specific activity: The concentration of the radionuclide per unit volume or weight (i.e., in mCi/mg). High specific activity is optimal.
Radiative abundance: Also known as radiation yield; the likelihood that the decay of radioactive substance will result in desired emissions.
FDA, U.S. Food and Drug Administration.
Production of Radionuclides
Naturally occurring radionuclides are often heavy, toxic elements (e.g., uranium, actinium, thorium, radium, and radon) with very long half-lives (>1000 years). Most of these radioactive elements have no role in nuclear medicine, and radionuclides for clinical use are commonly produced artificially. Table 4.1 provides the physical properties of single-photon-emitting radionuclides used in medical imaging with a gamma camera. Dual-photon positron-emitting agents are listed in Table 4.2, and Table 4.3 notes several important radionuclides for therapy purposes. Appendix 2 contains a periodic table of the elements for reference.
Radionuclide | Principal Mode of Decay | Physical Half-Life | Principal Photon Energy in keV (% abundance) | Production Method |
---|---|---|---|---|
Mo-99 | β – | 2.8 d | 740 (12), 780 (4) | Reactor |
Tc-99m | Isomeric transition | 6 hr | 140 (89) | Generator (Mo-99) |
I-131 | β – | 8 d | 364 (81) | Reactor |
I-123 | EC | 13.2 hr | 159 (83) | Cyclotron |
Ga-67 | EC | 78.3 hr | 93 (37), 185 (20), 300 (17), 395 (5) | Cyclotron |
Tl-201 | EC | 73.1 hr | 69-83 (Hg x-rays), 135 (2.5), 167 (10) | Cyclotron |
In-111 | EC | 2.8 d | 171 (90), 245 (94) | Cyclotron |
Xe-133 | β – | 5.2 d | 81 (37) | Reactor |
Co-57 | EC | 272 d | 122 (86) | Cyclotron |
Cs-137 | β – | 30.17 yrs | 662 | Reactor |
Radionuclide | Physical Half-Life (min) | Positron Energy E max (MeV) | E mean (MeV) | Maximum Range in Soft Tissue (mm) | Mean Range in Soft Tissue (mm) |
---|---|---|---|---|---|
C-11 | 20.4 m | 0.96 | 0.39 | 4.2 | 1.2 |
N-13 | 10 m | 1.2 | 0.49 | 5.5 | 1.8 |
O-15 a | 2 m | 1.73 | 0.73 | 8.4 | 3.0 |
F-18 | 110 m | 0.63 | 0.25 | 2.4 | 0.6 |
Ga-68 | 67.8 m | 1.90 | 0.84 | 10.3 | 2.9 |
Rb-82 | 1.3 m | 3.38 | 1.56 | 8.6 | 5.9 |
Zr-89 a | 78.4 d | 0.902 | 0.40 | 3.8 | 1.3 |
Cu-64 a | 12.7 hr | 0.653 | 0.28 | 2.5 | 0.7 |
Beta Minus Emitters | |||||||
---|---|---|---|---|---|---|---|
Radionuclide | Half-Life | E max (MeV) | E ave (MeV) | Maximum Particle Range (mm) | Mean Particle Range (mm) | Gamma (γ) Photons Suitable for Imaging | Examples of Uses |
Iodine-131 (I-131) | 8.01 days | 0.606 | 0.81 | 2.4 | 0.4 | 364 keV (81%) | Thyroid cancer, hyperthyroidism |
Yttrium-90 (Y-90) | 64.1 | 2.28 | 0.94 | 11.3 | 3.6 | No γ; Bremsstrahlung radiation | CD20 Antibodies: lymphoma Microspheres: Colon cancer hepatic metastases Hepatocellular cancer |
Lutetium-177 (Lu-177) | 6.7 d | 0.50 | 0.14 | 1.7 | 0.28 | 208 (11%) | Neuroendocrine tumor |
Samarium-153 (Sm-153) | 46.3 hr | 0.81 | 0.22 | 3.1 | 0.7 | 103 (29%) | Bone metastases |
Rhenium-186 (Re-186) | 3.7 d | 1.07 | 0.33 | 3.6 | 1.2 | 137 keV (9%) | Bone metastases |
Strontium-89 (Sr-89) | 50.5 days | 1.496 | 8.0 | 2.4 | 910 (0.01%) | Bone metastases | |
Phosphorus-32 (P-32) | 14.3 days | 1.71 | 0.70 | 7.9 | 2.6 | None | Bone metastases, a intraperitoneal ovarian cancer metastases, pleuroperitoneal fistulas |
Alpha Emitters | |||||
---|---|---|---|---|---|
Agent | Half-Life | Decay | E α (MeV) | Principal Gamma (keV) and % Abundance | Uses |
Radium-223 (Ra-223) | 11.4 days | α multistep daughters also decay <SPAN role=presentation tabIndex=0 id=MathJax-Element-2-Frame class=MathJax style="POSITION: relative" data-mathml='(αβ−)’>(??−)(αβ−) ( α β − ) | 5–7.5 | 82, 154, 270 (γ total 1.1%) | Prostate metastases |
Actinium-225 (Ac-225) | 10.0 days | α | 5.9 | 99 (5.8%) | Experimental applications |
Bismuth-213 (Bi-213) | 45.6 min | α/β – | 6.0 | 440 (27.3%) | Experimental applications |
Lead-212 (Pb-212) | 10.64 hours β – | Bi-212 daughter <SPAN role=presentation tabIndex=0 id=MathJax-Element-3-Frame class=MathJax style="POSITION: relative" data-mathml='αβ↑−’>??↑−αβ↑− α β ↑ − | 6.1 | 238.6 (43.1%) | Experimental applications |
Astatine-211 (At-211) | 7.2 hr | α | 6.0 | 500–900 keV (≤ 1%) 77–92 keV x-rays from Po-211 | Experimental applications |
a Some applications are not approved by the U.S. Food and Drug Administration.
Medical isotope production involves one of four methods: nuclear fission or neutron activation in a nuclear reactor, charged-particle bombardment in a particle accelerator (i.e., a cyclotron), or decay of a radioactive parent forming the desired agent in a radionuclide generator ( Fig. 4.1 ). Production methods are outlined in Table 4.4 . The various reactions involved in production can be annotated in equation form, noting the type of reaction, any particle involved in the transformation, as well as the initial isotope and final product, as presented in the examples listed in Box 4.2 .
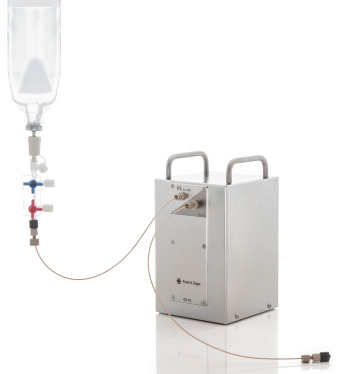
Characteristic | Cyclotron | Nuclear Reactor | Radionuclide Generator | ||
---|---|---|---|---|---|
Fission | Neutron Activation | ||||
Bombarding particle | Proton, deuteron, alpha, tritium | Neutron | Neutron | Production by decay | |
Product | Proton excess | Neutron excess | Neutron excess | Proton or neutron excess | |
Decay mode | β + Electron capture | β – | β – | Varies | |
Carrier-free | Yes | Yes | No | Yes | |
High specific activity | Yes | Yes | No (difficult to separate chemically) | Yes | |
Cost | High a | Low | Low | Tc-99m Low Ga-68 High | |
Common medical radioisotopes produced | β – | Mo-99 I-131 Xe-133 Cs-137 | P-32 Sr-89 Sm-153 Mo-99 I-131 b | ||
β + | F-18 C-11 N-13 O-15 Zr-89 | Ga-68 Rb-82 | |||
Electron capture | Tl-201 I-123 Ga-67 In-111 | I-125 Cr-51 | |||
Isomeric transition | Tc-99m Kr-81m |
a Historically higher than reactor production; F-18 for fluorodeoxyglucose (FDG) now economical.
b From 130 Te (n, γ) 131 Te <SPAN role=presentation tabIndex=0 id=MathJax-Element-4-Frame class=MathJax style="POSITION: relative" data-mathml='I(βI–)→’>I(?I−−)−→−−−−−I(βI–)→
I ( β I – – ) →
131 I.
Common abbreviations: p, proton; n, neutron; d, deuteron; α , alpha; γ , gamma; f, fission; β – , beta minus; EC, electron capture.
Equation shorthand format:
Target atom (irradiating particle, emission) radionuclide product
Examples:
Cyclotron Production
Fluorine-18:18O(p,n)18F
Gallium-67:68Zn(p,2n)67GaIodine-123:124Te(p,2n)123I
66Zn(d,−n)67Ga121Sb(α,−2n)
66Zn(d,−n)67Ga121Sb(α,−2n)
122Te(d,n)123I
Indirectproduction:124Xe(p,n)123Cs(→⊥(β↑+)(↓↑123)Xe(→⊥(β↑+)(↓↑123)I(carrier-free)
Reactor Production
Fissionreaction(n,f):235U+n01→236U→M4299o+SS0135n+2n01OR235U(n,f)99Mo
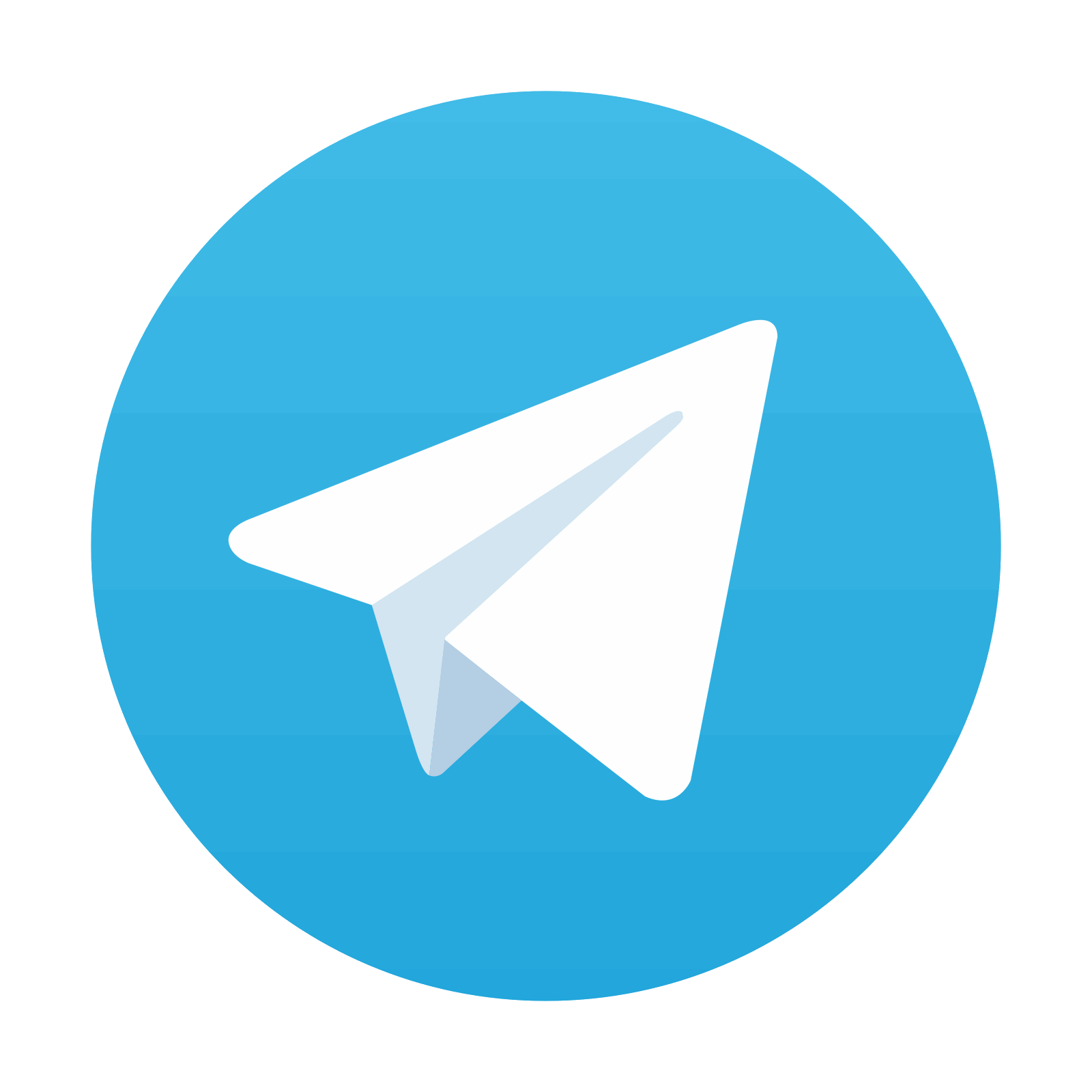
Stay updated, free articles. Join our Telegram channel

Full access? Get Clinical Tree
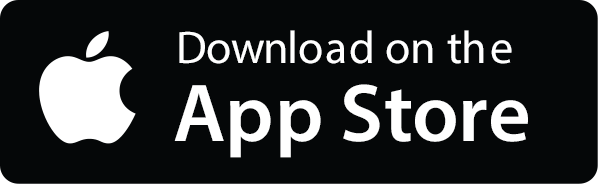
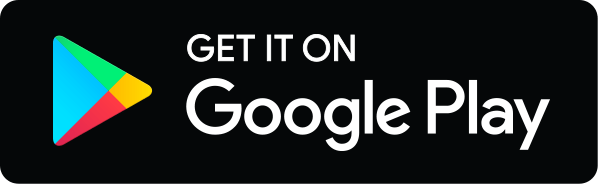
