Noninvasive imaging of the vascular renal system is a common request in diagnostic radiology. Typical indications include suspected renovascular hypertension, vasculitis, neoplasm, vascular malformation, and structural diseases of the kidney. Profound knowledge of the renal anatomy, including vascular supply and variants, is mandatory for radiologists and allows for optimized protocolling and interpretation of imaging studies. Besides renal ultrasound, computed tomography and MR imaging are commonly requested cross-sectional studies for renal and renal vascular imaging. This article discusses basic renal vascular anatomy, common imaging findings, and current and potential future imaging protocols for various renovascular pathologic conditions.
Key points
- •
Computed tomographic angiography and magnetic resonance angiography are appropriate imaging modalities to investigate renal vascular pathologic conditions.
- •
Technical advances in computed tomography and magnetic resonance have led to improved image quality for evaluation of suspected renal vascular pathologic conditions.
- •
Knowledge of imaging appearances of different renal vascular pathologic conditions is critical for accurate diagnosis.
Introduction
Noninvasive imaging of the vascular renal system is a common request in diagnostic radiology. Typical indications include suspected renovascular hypertension, vasculitis, neoplasm, vascular malformation as well as structural diseases of the kidney. Profound knowledge of the renal anatomy, including vascular supply and variants, is mandatory for radiologists and allows for optimized protocolling and interpretation of imaging studies. Aside from renal ultrasound, computed tomography (CT) and magnetic resonance (MR) imaging are commonly requested cross-sectional studies for renal as well as renal vascular imaging. The aim of this article is to discuss basic renal vascular anatomy, common imaging findings as well as current and potential future imaging protocols for various renovascular pathologic conditions.
Renal artery anatomy and variants
Right and left renal arteries typically originate from the aorta at the level of the second lumbar vertebral body (L2). The origin of the right renal artery is typically slightly higher than the origin of the left renal artery. The right renal artery is longer than the left renal artery and courses inferiorly posterior to the inferior vena cava (IVC) and the right renal vein before reaching the right renal hilum. The left renal artery is more horizontally oriented and courses posterior to the left renal vein , ( Fig. 1 A). The main renal arteries typically are divided into 5 segmental arteries: apical, superior, middle, inferior, and posterior segmental arteries. The segmental arteries further divide into lobar branches. The junction between the anterior two-thirds and posterior one-third of the kidney, known as the Brödel bloodless line of incision, is a relatively avascular plane separating the posterior arterial segment from the other arterial segments. Awareness of this plane can help avoid bleeding complications in percutaneous nephrostomy. , Before reaching the renal hilum, the renal arteries give rise to a few additional branches, such as the ureteric artery, the inferior adrenal artery, and the capsular artery. As part of the evaluation of pretransplant donor patients, it is important to describe the branching pattern of the first 2 cm of the renal arteries in order to prevent complications related to the vascular anastomosis of the transplant kidney. ,
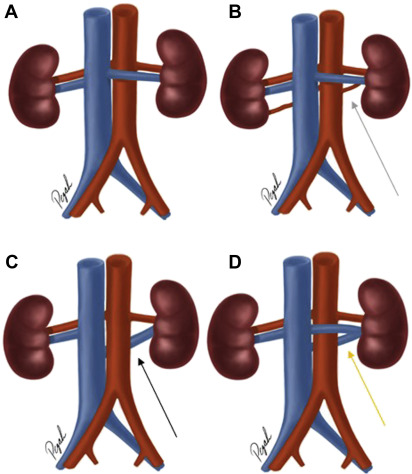
Accessory renal arteries ( Fig. 1 B) are present in 30% of the individuals and can be found bilaterally in about 10% of the individuals. Accessory renal arteries are much more common in individuals with renal fusion or positional anomalies of the kidneys, including horseshoe kidney or crossed fused renal ectopia. , Accessory renal arteries usually arise more inferior to the main renal arteries. However, they can arise anywhere between T11 and L4 vertebral bodies. Accessory renal arteries cross anterior to the ureter and can cause obstruction of the ureter.
Aberrant renal arteries (also known as polar renal arteries), in contrast to accessory renal arteries that enter the kidney through the renal hilum, enter the kidney through the renal capsule. Assessment of the exact anatomy of the renal arteries is important before renal donation surgery. The presence of more than 4 renal arteries is considered a relative contraindication for living renal donor surgery.
Renal vein anatomy and variations
A single vein typically provides drainage of deoxygenated blood from the kidneys into the IVC bilaterally. However, right and left renal veins are not symmetric, and there are clinically significant variations of the renal vein anatomy.
The right renal vein is typically located anteriorly to the right renal artery at the hilum of the right kidney. It typically courses in an anterior-superior direction toward the lateral aspect of the IVC. Unlike the left renal vein, the right renal vein typically has less anatomic variations and is less likely to have venous tributaries along its course. About 30% of individuals demonstrate more than 1 branch of the right renal vein. In about 6% of individuals, the right adrenal gland vein drains into the right renal vein, which reflects the normal anatomy on the left side. Retroperitoneal veins, such as lumbar veins, drain into the right renal vein in about 3% of individuals. ,
The left renal vein is longer (about 8.5 cm) than the right renal vein (about 2.5 cm) because it passes anterior to the aorta on its transverse course from the left renal hilum to the medial aspect of the IVC. The typical venous tributaries joining the left renal vein before its insertion into the IVC include (1) the left adrenal gland vein, (2) a branch of the inferior phrenic vein that drains into the superior aspect of the left renal vein, (3) the left gonadal vein that drains into the inferior aspect of the left renal vein, and (4) additional tributaries, in about 75% of individuals, to the posterior aspect of the left renal vein from lumbar and hemiazygos veins. A circumaortic left renal vein ( Fig. 1 C) is the most common anatomic variant of the left renal vein. It is characterized by 2 veins arising from the main left renal vein trunk, with 1 renal vein coursing anterior to the aorta and the other renal vein coursing obliquely inferiorly between the aorta and vertebral bodies; eventually both veins drain into the medial aspect of the IVC. This anomaly can infrequently cause hematuria, proteinuria, or massive bleeding if inadvertently injured during surgery. Another less common renal vein variant is the retroaortic left renal vein (about 3%) ( Fig. 1 D). Here, the left renal vein courses posterior to the aorta before it drains into the IVC, usually at a lower level than typical for a normal left renal vein insertion. Much less likely, the retroaortic left renal vein drains into the left common iliac vein. The presence of a retroaortic left renal vein has also been associated with proteinuria and hematuria. ,
Imaging protocols
Computed Tomography Imaging
CT is a commonly used imaging modality for the noninvasive assessment of renal vascular anatomy and pathologic condition. The advantages of CT imaging include high-spatial resolution, fast image acquisition, and the availability of volumetric data sets for image processing, such as 3-dimensional maximum intensity projection (3D MIP) as well as multiplanar and curved planar reformations. MIP images are useful for the 3D depiction of small vascular pathologic conditions, such as aneurysms or pseudoaneurysms of the renal arteries and the analysis of the spatial relationship relative to adjacent renal vasculature branches. Curved planar reformations can be beneficial for the assessment of stenotic disease, for example, in tortuous renal arteries. Exposure to ionizing radiation may be a concern in younger patients, who may need to undergo repeated imaging studies. Contrast-associated acute kidney injury following the administration of iodinated contrast agent can occur as a complication particularly in patients with compromised renal function.
A typical imaging protocol for renal vascular imaging using state-of-the-art equipment can be characterized by the following parameters: 120-kVp tube potential (variable depending on patient size with a spectrum ranging from 70 to 150 kV depending on scanner type based on Care kV with a reference setting of 120 kV), CareDose with reference mAs of 200, collimation of 192 × 0.625 mm, pitch of 0.6, and a rotation time of 0.5 second. Field-of-view in z-direction from 2 cm above the level of the kidneys to the level of the aortic bifurcation in the arterial phase and through the entire pelvis including bladder on the venous phase. Patients receive 100 to 150 mL of iodinated contrast material at 4 mL/s injection rate. For arterial phase imaging, bolus tracking with region of interest placed in the aorta approximately 2 cm above the renal arteries with a threshold of 150 HU. Venous phase acquisition with 80-second scanning delay after injection.
The emergence of dual-energy CT technology has provided many opportunities in improving identification and characterization of different pathologic conditions as well as the mitigation of traditional CT imaging artifacts, including beam hardening. Dual-energy technology can provide benefits for renovascular imaging. Using virtual monoenergetic images (at lower peak kilovolt) retrospectively reconstructed from dual-energy datasets, contrast enhancement of the abdominal vasculature can be improved compared with conventional polyenergetic images. Therefore, the use of dual-energy technology offers the potential of contrast dose reduction without compromising image quality, a feature particularly beneficial for patients with poor renal function. ,
Renal Magnetic Resonance Angiography
MR angiography provides a powerful alternative to CT angiography in the evaluation of renal vasculature. Capable of achieving spatial resolution close to that acquired with CT, MR offers the additional benefit of imaging without the use of ionizing radiation.
Both 1.5-T and 3-T systems can be used to achieve high-resolution MR angiograms (MRA). In general, the voxel size should be kept to ≤1 mm 3 to optimize spatial resolution. Isotropic voxels are ideal because they enable multiplanar postprocessing and the generation of 3D volumetric renderings.
When adjusting MR acquisition parameters, it is important to be mindful of changes that affect the signal-to-noise ratio (SNR). The acquired voxel size is directly proportional to SNR. As a result, decreasing the voxel size, in isolation, without changing other parameters, will decrease the SNR. Time to acquisition and field strength similarly have proportional effects on the SNR. Because signal is proportional to the square of field strength, whereas noise increases linearly, 3-T MR systems have a theoretic improvement in SNR of up to 2-fold when compared with 1.5-T systems. This gain in SNR at 3-T MR can in turn be used to improve spatial resolution and/or data acquisition speed. Therefore, MR angiography at 3 T is the preferred option for renal vascular imaging, as available.
In addition to the utilization of stronger field strengths, MRA can be improved with the utilization of parallel imaging. Parallel imaging relies on the inherent differences in signal detected by multiple coils, as a result of their position, to obtain spatial information about the object being imaged. The use of spatial information from the coils decreases the number of phase-encoding steps needed, and in turn, decreases the time of image acquisition. Improving acquisition speed decreases the length of breath-holding required and minimizes motion artifact.
An additional consideration in contrast-enhanced renal MRA is the type of contrast used. A benefit in the selection of MR renal angiography as opposed to CT is the ability to avoid iodinated contrast. Many of the patients requiring renal angiography have impaired renal function. Described by the American College of Radiology as a “real, albeit rare, entity,” contrast-induced nephropathy (CIN) is the sudden deterioration in renal function that is caused by the intravascular administration of iodinated contrast medium. CIN is a causative diagnosis and a subgroup of postcontrast acute kidney injury. The preponderance of evidence in numerous studies has shown gadolinium contrast agents not to be a cause of CIN when administered at recommended standard doses. Although the potential avoidance of CIN makes contrast-enhanced MR angiography with gadolinium-based contrast agents (GBCA) an attractive diagnostic option, caution must be used in patients with severe, end-stage disease (chronic kidney disease [CKD] 5, growth factor receptor [GFR] <15 mL/min/1.73 m 2 ) and severe CKD (CKD 4, GFR 15–29 mL/min/1.73 m 2 ) in whom there is a 1% to 7% risk of developing nephrogenic systemic fibrosis (NSF) after exposure to a class 1 GBCA. If deemed necessary, use of gadolinium-based contrast in patients with impaired renal function should be isolated to group II agents for which the risk of NSF is low or nonexistent.
After the recognition of the small, albeit real risk of NSF with use of GBCA in patients with advanced CKD, interest has developed in the utilization of noncontrast MR angiographic techniques, such as electrocardiogram-gated Fourier fast spin echo and balanced steady-state free precession imaging. Studies have shown that these noncontrast techniques can be a useful first step in evaluating the renal vasculature, serving as a means of triaging patients to a contrast-enhanced examination, as needed. ,
Similar to contrast-enhanced computed tomography angiograms (CTA), when using GBCA contrast-enhanced MRA, the contrast injection should be optimized for the renal arteries. One method of ensuring proper timing is to use a small volume of contrast, for example, 1 mL, as a test bolus, to time the arrival of contrast at the level of the renal arteries. The calculation of time to arrival and execution of optimal imaging timing on a subsequent full-dose contrast administration can be complicated by variations in breath-holding and is prone to human error. For these reasons, the use of time-resolved imaging is commonly used as an alternative with imaging initiated at the same time as the contrast administration. In addition to ensuring optimal bolus timing, time-resolved imaging can be performed, which provides the added benefit of real-time visualization of blood flow, and can prove useful in the detection of arterial stenoses, arteriovenous fistulas, or malformations. The use of a non-CBCA, such as ferumoxytol, may serve as an attractive alternative in patients with severe and end-stage renal disease. Ferumoxytol is based on ultrasmall paramagnetic iron oxide particles. The compound was initially investigated as an MR contrast agent, but ultimately was approved by the Food and Drug Administration (FDA) for the intravenous treatment of anemia in patients with end-stage renal disease. , Ferumoxytol benefits from both excellent T1-shortening properties and a long intravascular half-life of 14 to 21 hours, allowing for high-resolution steady-state angiography at doses as low as 1 mg/kg up to a total of 510 mg.
Although time-resolved imaging with ferumoxytol is technically feasible, a rapid bolus injection is not advised per FDA recommendations as outlined in the package insert of the compound. The long half-life and resultant steady-state contrast in the blood pool obviates bolus timing in most cases. As with CT angiography, analysis of MR angiographic images is best performed using a 3D postprocessing suite with multiplanar and curved planar reformatting capabilities. 3D volumetric renderings are a powerful visual tool for demonstrating vascular pathologic condition, particularly for the nonradiologist. When high-resolution, isotropic images are acquired, renal MRA can also be 3D printed for surgical planning.
Renal vascular pathologic condition
Renal Artery Stenosis
Renal artery stenosis is the leading cause of secondary hypertension. It is found in 1% to 5% of all patients who suffer from hypertension. The most common cause of renal artery stenosis is atherosclerosis, with the highest prevalence in older male patients. Typically, the proximal 2 cm of the renal artery are affected. This results in a decrease in renal perfusion and consequently an increase in systemic blood pressure owing to activation of renin-angiotensin cascade. Bilateral renal artery stenosis is seen in 30% of affected patients. Other less likely causes of renal artery stenosis include fibromuscular dysplasia (FMD), dissection, embolic disease, vasculitis, or external compression, which are discussed separately. ,
Defining a clinically relevant cutoff for the degree of luminal narrowing is controversial. However, a stenosis exceeding 50% of diameter reduction is typically considered significant, although symptoms may not occur until the stenosis exceeds 70%. It is noteworthy that in a metaanalysis of 947 patients from 113 centers with renal artery stenosis owing to atherosclerosis, there was no difference between medical therapy and renal stenting. Therefore, investigating the presence, cause, and degree of stenosis appears particularly relevant in patients with failure of medical management of hypertension, new-onset hypertension, in young patients and patients with progressive renal insufficiency. , ,
Renal Doppler ultrasound is frequently used as a first-line imaging modality for the evaluation of renal artery stenosis. However, the diagnostic accuracy of renal Doppler ultrasound is limited for several reasons, including the experience of the technologist or radiologist performing the study, patient’s body habitus, or presence of overlying bowel gas. Typically, a stenosis is considered significant if the peak systolic velocity (PSV) exceeds 200 cm/s or if the renal artery PSV/aorta PSV ratio exceeds 3.5:1.
CTA is an excellent choice for the evaluation of the anatomy of the renal arteries with the possibility of providing multiplanar reconstructions ( Fig. 2 ). CTA is a rapid noninvasive technique with higher resolution compared with MRA. The sensitivity and specificity of CTA for the diagnosis of renal artery stenosis exceeding 50% have been reported to be in the range of 88% and 96% and 77% and 98%, respectively. , Because of its high negative predictive value, a CTA interpreted as normal essentially excludes significant stenotic disease of the renal arteries. A decrease in cortical enhancement, poststenotic dilation, and kidney atrophy are considered secondary findings indicative of significant renal artery stenosis.
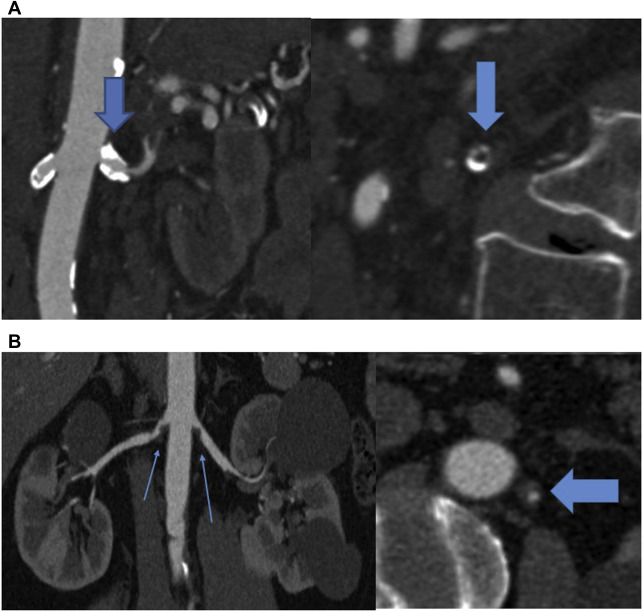
Gadolinium-enhanced 3D MRA of the renal arteries or steady-state free precession and arterial spin labeling methods are MR imaging techniques available for the evaluation of renal artery stenosis. Sensitivities and specificities for the diagnosis of renal artery stenosis for MRA have been reported to range between 88% and 100% and 71% and 100%, respectively. Other methods currently under investigation are blood-oxygen level–dependent MR imaging to study kidney oxygenation. Advanced MRA techniques have been proposed for artifact-free in-stent lumen visualization. Generally, in patients with an endothelial growth factor receptor less than 30, gadolinium should not be administered because of the risk of NSF.
Renal Artery Entrapment
In young patients with hypertension, a potential underlying cause may be the compression of the proximal portion of any of a renal artery by the psoas musculature or a crus of the diaphragm resulting in a narrowing of the affected renal artery. This entity is typically seen in less than 5% of patients with hypertension. This finding is more likely to involve the left renal artery. The modality of choice to evaluate the relevant anatomy, including course, degree, and length of renal artery compression, is CT angiography. The initial treatment of choice is stenting of the affected artery. However, the success of the procedure may be challenged because of stent failure as a consequence of mechanical forces generated by repetitive muscle contractions, and surgical repair may need to be considered. ,
Renal Artery Dissection
Renal artery dissections can occur as isolated or as part of aortic dissections extending into the renal arteries. Associated clinical symptoms may occur in the form of deteriorated renal function and increase in blood pressure or acute onset of flank pain. Isolated cases are mainly complications of endovascular interventions or sequela of blunt abdominal traumas. Rarely, spontaneous dissections that mainly involve the distal portions of the main renal arteries can occur in patients with FMD, history of cocaine abuse, collagen vascular syndromes such as Ehlers-Danlos or Marfan syndrome, or in the context of cystic medial necrosis. ,
Contrast-enhanced CTA is the modality of choice in the evaluation of a suspected dissection ( Fig. 3 ) to demonstrate the presence and extension of an intimal flap; however, despite the superior spatial resolution of CTA, small flaps and thrombotic changes of small-caliber vessels may be difficult to visualize. Secondary signs of renal artery dissections include luminal narrowing, abrupt terminating of the vessel, also referred to as vessel cutoff sign, or renal ischemia. , If the patient is clinically stable, observation and anticoagulation are recommended treatment options. In unstable patients, the treatment of choice is endovascular stent repair. ,
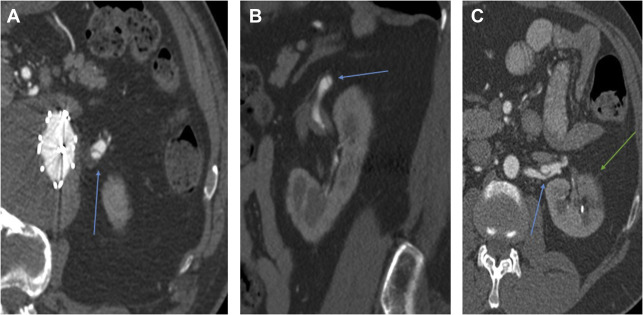
Renal Artery Aneurysm and Pseudoaneurysm
Renal artery aneurysms (RAA) ( Fig. 4 ) develop following a weakening of the renal arterial wall with consequent expansion of the arterial lumen owing to increased intraluminal pressure. RAAs are rare, with an estimated prevalence of 0.1%. They are found mostly in patients with systemic arterial hypertension. , RAAs tend to be asymptomatic and therefore typically diagnosed incidentally on cross-sectional imaging of the abdomen. When symptomatic, they can be associated with pain, hematuria, and renal infarction. Complications include rupture, thrombosis, and embolism. , RAAs mostly occur in the main right or left renal artery, most commonly at the site of the renal artery bifurcation. Additional aneurysms originating from visceral arteries can be seen in up to 6.5% of patients with RAAs. On gray-scale ultrasound imaging, noncalcified RAAs appear anechoic, whereas calcified aneurysms may demonstrate increased echogenicity. Doppler sonography can be used to assess the perfusion pattern of RAAs. CT angiography is considered the modality of choice because it allows for the comprehensive assessment of aneurysms in abdomen and pelvis.
