Fig. 22.1
Image fused 4D-PET/CT of patient with pancreatic tumor for radiotherapy planning. Standard planning target volume (STD-PTV) is correlated with 4D-CT image (red outlines) and 4D-planning target volume (4D-PTV) with 4D-PET image (yellow outlines). The use of information obtained from 4D studies allows the definition of target volumes as 4D-PTV reduced compared to those obtained with STD-PTV
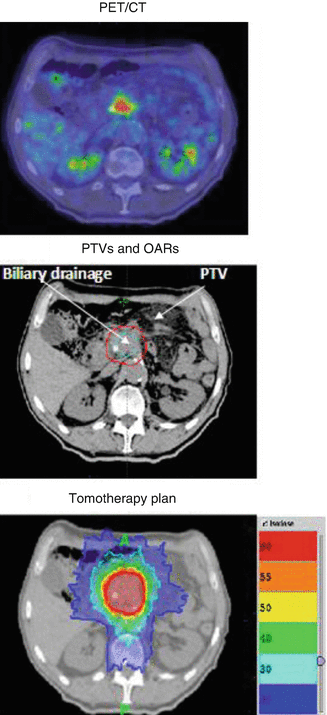
Fig. 22.2
Patient with pancreatic adenocarcinoma (histological grade: T4N0) previously treated with chemotherapy (5-FU). Helical tomotherapy planned on planning target volume (PTV) based on PET/CT image with the dose of 60 Gy, 2 Gy/f
18F-fluorodeoxyglucose (FDG) is the most commonly used and the only oncologic PET tracer approved by the Food and Drug Administration (FDA) for routine clinical use. More than 90 % of oncologic PET imaging is performed by FDG-PET due to the increased metabolism of glucose by tumor cells in most cancers. In addition to diagnosis, staging, restaging, and monitoring response to cancer treatment, FDG-PET can be useful for selection or delineation of RT target volumes [9]. However, FDG is not a tumor-specific substance; its accumulation in benign lesions, such as inflammation, may cause false-positive results which lead to low tumor specificity.
In addition to glucose, amino acids and choline have also shown increased tumor consumption and tumor specificity. Radiolabeled radiotracers, such as 11C-methionine (MET) and 11C/18F-labelled choline (CHO), may in fact have significant potential for tumor detection in organ sites with an undesirable FDG-PET background such as with brain tumor or in tumors with low intrinsic FDG uptake, such as prostate cancer (PCa). Also, 11C-acetate (ACE) acts as a probe of tissue metabolism through entry into catabolic or anabolic metabolic pathways as mediated by acetyl-coenzyme A, and it is also a useful PET tracer for various cancer types, such as lung cancer (LC), hepatocellular carcinoma, renal cancer (RC), PCa, and astrocytomas, which makes it a potential substitute for FDG in target volume selection and delineation in RT planning [10, 12–14].
In RT treatment, it is reported that there is a strong correlation between locoregional control (LRC) and overall survival (OS) [15–18]. A straightforward way to improve local tumor control, as proved by large bodies of evidence, is to increase the radiation dose further [19, 20]. Current treatment prescriptions are already close to patient tolerance making it nearly impossible to raise the dose prescription of the whole target tumor. New biological imaging methodologies such as PET can be used to draw a three-dimensional (3D) map of radiobiological relevant parameters as its inherent potential to trace the real target volume – volume consisting of tumor cells that requires a therapeutic dose to control the disease. The RT prescription usually takes larger target region to some primary dose and then boosts the GTV (including high-uptake regions) to a higher dose. The primary and boost doses are typically planned for spatially homogeneous delivery to their respective target regions. There has been also increasing clinical interest in delivering a nonhomogeneous dose to the target based on FDG-PET uptake, with the aim of improving LRC [21].
An increased precision of target subvolume localization by using PET/CT information plus a therapeutic dose delivered by dose painting may have an additional effect to improve LRC and further reduce the potential of tumor recurrence.
Metabolic PET can provide information that impact RT planning in several ways, by detecting a tumor that is missed by CT or MRI, by detecting additional tumor regions outside the tumor volume as defined by CT or MRI, and by showing subregions with increased or altered metabolism within the GTV that could be preferentially targeted and treated with escalated radiation doses.
22.1.1 Lung Cancer
LC is the leading cause of cancer death in both men and women. Eighty percent of LC is non-small-cell lung cancer (NSCLC). While difficult to treat, the best opportunity to cure NSCLC is by radical surgical resection in the early stages of disease. RT is a key treatment modality in the curative treatment of patients with LC (Figs. 22.3 and 22.4). Recent progress in combined modality treatments incorporating chemotherapy (CH) and radiation, with or without surgery, as well as technical advances in radiation delivery, has led to significant improvements in treatment outcomes. Accurate evaluation of lymph node (LN) metastases is crucial for planning curative RT.
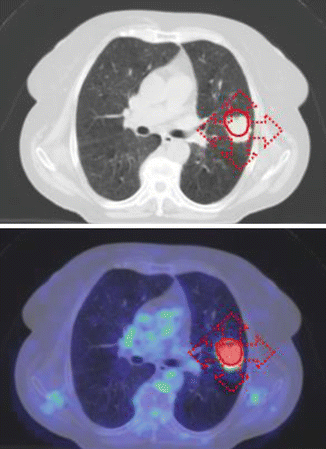
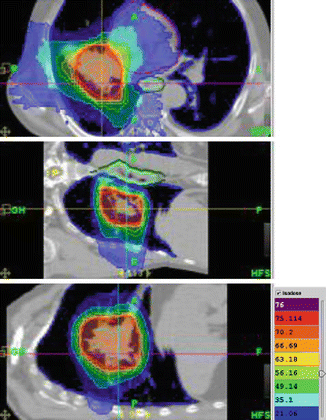
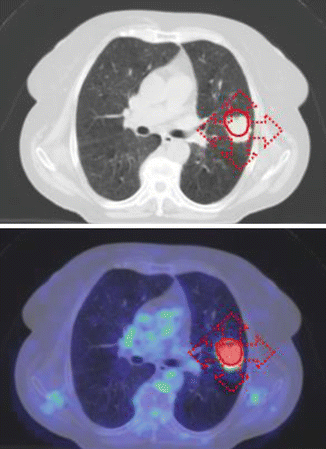
Fig. 22.3
PET/CT images contoured with organ motion in patient with lung cancer for radiotherapy treatment planning
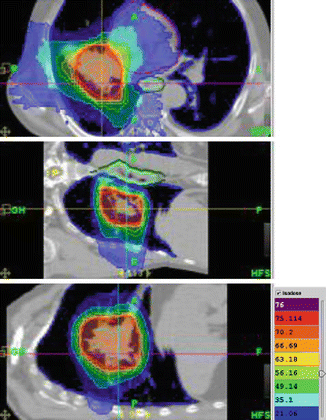
Fig. 22.4
Tomotherapy treatment planned in an 88-year-old man with lung cancer. The plan has 39 fractions defined for a planned delivery of 70.2 Gy. This dose was given on area in red line and is defined as planned target volume
FDG-PET has been widely used in evaluation of patients with LC. The overall sensitivity, specificity, and accuracy of FDG-PET for detection of LC are very high for primary, residual, and recurrent disease. FDG-PET scan is more sensitive than CT in detection of LN involvement and distant metastases in NSCLC [9, 22]. Changes in the target volumes of over 20 % and stage alteration of 20–50 % have been consistently observed when FDG-PET was incorporated in target volume delineation (TVD). Most prominent changes are often associated with the presence of atelectasis in the treated areas, or the identification of additional LN disease, which may be difficultly visualized on CT [23–29]. In addition, incorporation of FDG-PET in the delineation of regional LN disease may lead to a decrease in the LN-GTV. This has been demonstrated in patients with N2-N3 disease by van Der Wel et al., who showed a PET-related decrease of the LN-GTV from 13.7 ± 3.8 to 9.9 ± 4.0 cm3 (p = 0.011) [29]. It led to significant decrease in radiation dose to the esophagus (V55 decreased from 30.6 ± 3.2 to 21.9 ± 3.8 %, p = 0.004) and the normal lungs (V20 decreased from 24.9 ± 2.3 to 22.3 ± 2.2 %, p = 0.012). As a result, dose escalation from 56.0 ± 5.4 to 71.0 ± 13.7 Gy (p = 0.038) became feasible, which led to improved tumor control probability (TCP), that is, the probability that a given dose of radiation will provide tumor control or eradication considering the specific biological cells of the tumor, from 14.2 ± 5.6 to 22.8 ± 7.1 % (p = 0.026) without accounting for geometric misses, and improved TCP from 12.5 to 18.3 % when it is accounted.
These findings further demonstrate the advantage of incorporating FDG-PET information in TVD especially for stage III NSCLC, which makes dose escalation possible. The theoretical dose escalation level was addressed in many planning studies. Different dose-painting strategies to shape the radiation dose according to the functional image information have been proposed recently: dose escalation and dose redistribution. Dose escalation applies an additional dose to the functional subvolumes of the target, whereas dose redistribution consists of increasing the dose to the radioresistant areas while reducing the dose to the rest of the tumor in a way to keep the mean dose constant.
There are two strategies for the realization of dose escalation: dose painting by contours (DPBC) and dose painting by numbers (DPBN). DPBN intends to increase the additional dose gradually, according to the local PET voxel intensities, while a homogeneous dose of BTV which contoured by a threshold created in PET image is given in DPBC. For both DPBC and DPBN plans, strict planning constraints set for the organ at risk (OAR) should be complied. In addition, as the feasibility of technique is largely proved [30–36], both are therefore considered clinically acceptable and alternatives for boosting subvolumes.
MacManus et al. reported that metabolic response correlated well with RT outcome. Complete metabolic response (CMR) predicted good RT outcome: 1-year survival for CMR and non-CMR patients was 93 % and 47 %, respectively, and 2-year survival was 62 % and 30 %, respectively [37]. Additionally, PET/CT scans have been shown to detect distant metastases that are missed by conventional scans, which is of obvious benefit to patients who may require additional treatment [38].
Respiratory motion can have a significant impact on static oncological PET/CT imaging where standardized uptake values (SUV) and/or volume measurements are important. The impact is highly dependent upon motion amplitude, lesion location and size, attenuation map, and respiratory pattern. To overcome the motion effect, motion compensation techniques may be necessary in clinical practice to improve the tumor quantification for determining the response to therapy or for RT treatment planning. PET/CT imaging of the lung and abdomen region is generally affected by patient respiratory motion, which can lead to underestimation of maximum SUV (SUVmax) of a region of interest (ROI), overestimation of tumor volume, and mismatched PET and CT images that yield attenuation correction (AC) errors, registration errors, and tumor mislocalization [39]. It has been reported that various respiratory motion correction methods can cause tumor SUVmax to increase as much as 159 % and tumor volume to decrease as much as 43 % compared to those of the conventional measurement in patient studies [40, 41].
Currently hypoxia is deeply evaluated with the use of different PET tracers such as 18F-fluoromisonidazole (FMISO), 18F-fluoroazomycinarabinoside (FAZA), and copper-64 diacetyl-bis (N4-methylthiosemicarbazone) (ATSM). A crucial advantage of PET imaging for studying tumor hypoxia is its capacity to provide in vivo 3D images of the distribution of positron emitting tracers that selectively bind to hypoxic tissue in a safe and noninvasive manner [42]. Postema et al. published results using FAZA in NSCLC patients reporting an incidence of baseline hypoxia in 7 of 13 patients (54 %) [43].
22.1.2 Head and Neck Cancers
Oncologic imaging plays an important role in head and neck cancers (HNC) in detection, staging, restaging, and therapy response assessment of these tumors. Accurate staging at the time of diagnosis is critical for selecting the appropriate treatment strategy. Unfortunately, at the time of initial diagnosis, more than 50 % of patients already present with regional LN metastases or even distant metastases. In this population, RT plays a critical role when surgery is contraindicated. However, radical treatment is associated with increased toxicity, particularly when concomitant CH or altered fractionation strategies are used. A meta-analysis of both combined chemoradiation (CRT) and altered fractionation confirmed significant LRC and survival benefits overall but were unable to demonstrate a survival benefit in the >70 age group [44].
FDG-PET can provide important complementary information for RT planning in HNC (Fig. 22.5), and its role has been reviewed in several publications [45–48]. Although there are some contradictory results [49, 50], many clinical studies have shown that the uptake of FDG, usually measured as the SUVmax, is a significant predictor of prognosis for many tumor sites, including HNC [51–53]. Moreover, high FDG uptake is correlated with increased local failure and shorter survival [54–56]. The precise mechanism causing tumors with higher SUV values to present a poorer treatment response is unknown. Tumors with a high SUV may:
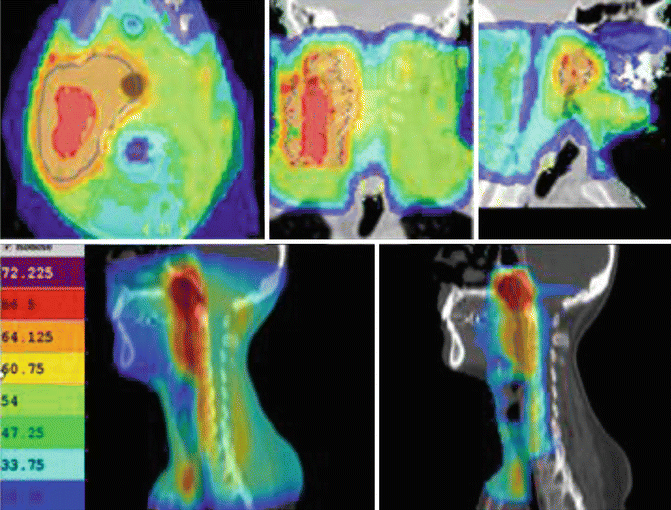
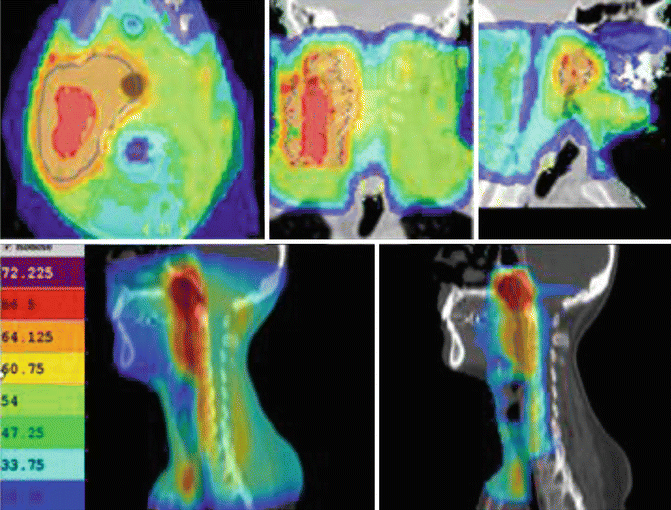
Fig. 22.5
Radiotherapy treatment planning in a patient affected by head and neck cancer. Helical tomotherapy radiation treatment was delivered with the simultaneous integrated boost approach at different dose escalated to metabolic sub volume. A boost – 69 Gy – was given on FDG-PET-positive volume (red area); clinical target volume and clinical negative neck received 66 Gy (orange area) and 54 Gy (green area), respectively
or some combination of these options.
The vast majority of recurrences seem to be the result of insufficient dose delivered to portions of selected target volumes. Therefore, dose painting becomes a compelling strategy if a spatial variation in LRC can be established. Based on clinical outcomes, the FDG-avid region in a tumor is recognized as a possible target for dose escalation. Several planning and optimization studies have assessed the feasibility of dose boosting on a subvolume of a tumor, based on a FDG-PET image, using IMRT [36, 61]. Some clinical data suggest that to reach equal response rates, FDG-avid tumors are likely to require at least 10–30 % more dose than FDG non-avid tumors. However, due to the confounding effect of tumor volume/T-stage and other prognostic factors, tests of the estimated dose-modifying effect, using high-quality prospective clinical trial data, are needed.
Changes in the tumor and surrounding normal tissues during RT for HNC support treatment adaptations [62–64]. Adaptive radiotherapy (ART) appears to be superior over nonadaptive treatment: it increases minimum doses in the target structures and decreases the cumulative maximum dose in dose-painted areas. It also readjusts the dose-painting patterns. However, there is a disagreement over benefits of ART [65, 66] that originates from variations in timing and frequency of adaptive procedures, methods of plan adjustments to anatomical and biological changes, and methods of dose accumulation.
FDG-PET/CT can play various roles in the treatment of HNC including staging, restaging, and defining BTV for high-dose RT boost. Potentially, the GTV can be changed on the basis of PET information, facilitating sparing of nearby normal tissues and allowing dose escalation to relatively small subvolumes. In addition, biological imaging using FDG-PET may identify areas of tumor spread not recognized by morphological imaging, potentially improving the accuracy of GTV definition [67, 68].
22.1.3 Esophageal Cancers
The incidence of esophageal cancer ranks in the top ten worldwide with a relatively high mortality rate. Esophageal cancers consist primarily of two subtypes, adenocarcinoma (comprising 50–80 % of all esophageal cancer cases) and squamous cell carcinoma (comprising most of the remaining cases).
RT is one of the primary treatments for esophageal cancer. Combined CH and RT has proven superior to either therapy alone as primary treatment for esophageal cancer. Esophageal IMRT is associated with significantly better OS, LRC, and noncancer-related death compared with 3D conformal RT [69]. There are no prospective data yet, but retrospective series suggest that treatment is well tolerated and may allow delivery of a higher tumor dose, of significant interest for the treatment of medically inoperable elderly patients [70].
FDG-PET is not generally used for the initial diagnosis of esophageal cancer due to significant FDG uptake in inflammatory esophagitis, Barrett’s esophagus, and other benign diseases. FDG-PET plays also a limited role in the evaluation of regional LN disease in patients with esophageal cancer but has a high sensitivity for evaluation of local recurrence and a high sensitivity and specificity for detection of distant disease occurring outside the initial surgical field [71, 72].
Accurate delineation of GTV is a prerequisite for a successful treatment of this cancer with RT. In esophageal cancer, the determination of cephalic and caudal tumor extent by CT is rather uncertain. Drudi et al. found that the lengths of esophageal carcinoma measured by CT scan and esophagogram corresponded to the lengths of surgical specimens in only 32 % and 59 % of cases, respectively. Therefore, methods to increase the accuracy of TVD are needed. Several studies have reported that PET/CT could improve primary tumor and LN detection [73]. Furthermore, clinicopathological studies in surgically treated patients showed that CT scanning is reasonably good in showing radial tumor extent but poor in assessing longitudinal extent and that CT is often inaccurate when it is used to estimate the extent of LN involvement. PET is better than CT in showing tumor longitudinal extent. PET may be the only way to estimate the lower tumor border when an endoscope cannot be passed through a stenosed esophagus. Therefore, integrated PET/CT imaging aids the estimation of more accurate tumor volume and the distinction of precise anatomical barriers.
In a prospective trial of PET for RT planning in esophageal cancer, Leong et al. [74] showed that PET has a significant impact on GTV by identifying unsuspected LN involvement. Moureau-Zabotto et al. [75] showed that the addition of PET information to CT-based RT planning altered GTV values in 19 of 34 patients (56 %); GTV was reduced in 12 and increased in 7 (21 %). Han et al. [76] reported that an SUV cutoff of 2.5 provided an accurate estimation of GTV length. Vali et al. [77] reported that the use of a threshold of approximately 2.5 may be considered the best strategy for the delineation of GTV in esophageal cancer.
22.1.4 Breast Cancer
Breast cancer is the most common cancer in female patients and carries a significant risk for recurrence and metastases. Breast cancer is the second leading cause of death in women. For localized cancers, the 5-year survival rate is more than 96 %, but survival decreases dramatically with regional or distant metastatic disease. Strategies in managing breast cancer include various combinations of surgery, RT, and CH regimens. RT is mainly applied in three areas:
- (i)
adjuvant RT after breast surgery
- (ii)
RT for isolated recurrence after surgery
- (iii)
RT for metastatic disease
Breast cancer has several known characteristics that determine aggressiveness and can be used as therapeutic targets as well as a means for monitoring responsiveness to therapy. Published reports are limited in assessing the utility of FDG-PET in breast cancer RT planning [72]. At present, FDG-PET has not shown significant utility in RT planning after breast-conserving surgery. Whole breast irradiation after breast-conserving surgery is one of the standard treatments for the patients with early-stage breast cancer [80, 81]. After the whole breast irradiation, additional boost to the tumor bed has demonstrated considerable improvement in local control rate [82, 83]. In the modern era, CT-based planning has been used to individualize treatment and tailor RT treatment fields to encompass the breast and draining lymphatic while minimizing dose to the heart, lungs, and spinal cord. 3D planning has also enabled more accurate demarcation of the lumpectomy cavity at depth rather than relying solely on the surgical scar at the skin’s surface.
Preoperative PET/CT may provide the more reliable diagnostic tool to localize the tumor bed in breast cancer RT (Fig. 22.6). Other studies reported that the FDG-PET/CT can improve the delineation of lumpectomy cavity for partial breast irradiation. The PET/CT-derived planning target volumes (PTVs) are larger than the CT-derived PTVs and are not well-covered dosimetrically by CT-based treatment plans. A PET/CT-based treatment plan covers both PET/CT and CT PTVs with only a modest increase in the dose to the normal tissue, being able to influence treatment planning for partial breast irradiation [84]. While the importance of RT technique in preventing locoregional recurrence has been previously established in other malignancies such as cervical and HNC [85, 86], no studies have systematically examined the importance of RT field design with modern imaging modalities and treatment planning in preventing locoregional recurrence in breast cancer patients.
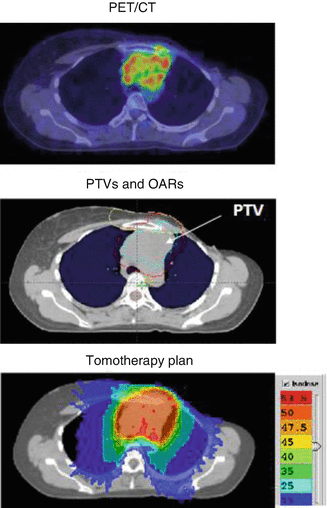
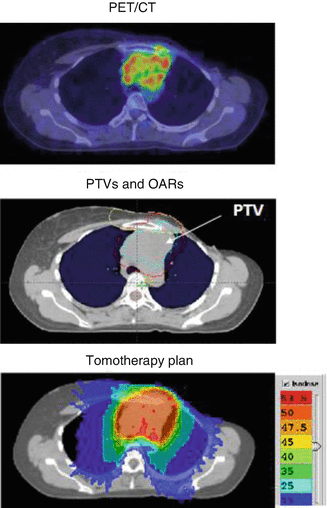
Fig. 22.6
A 50-year-old woman with metastatic breast cancer previously treated with hormone therapy and chemotherapy. Patient performed radiotherapy on left breast, supraclavicular fossa, internal mammary lymph node (1991), and chest wall (2003). At follow-up control patient presents recurrence in mediastinal lymph node. Helical tomotherapy was planned on planning target volume (PTV) based on PET/CT image with a dose of 60 Gy, 2 Gy/fraction
In patients with clinical stages II and III, PET/CT was documented to be superior to conventional imaging modalities for the detection of extra-axillary regional metastatic LN (infraclavicular, supraclavicular, and internal mammary LNs) and distant metastases [87–92]. The role of FDG-PET is especially prominent in the management of oligometastatic disease; a state of limited metastatic spread of disease is potentially curable with local therapy. This is an area in which RT is emerging as an important treatment modality [93].
Novel tracers, such as CHO, 18F-fluoroestradiol (FES), 18F-fluorothymidine (FLT), and progesterone-receptor in breast cancer, have the potential to add meaningful biomarkers to clinical imaging and treatment trials, to study tumor molecular physiology with the potential to improve diagnosis, treatment selection, drug development, and pharmacodynamic monitoring of the effects of treatment. These tracers are likely to move closer to clinical use and to become a useful tool for personalized treatment [94].
22.1.5 Lymphomas
Lymphomas often respond well to therapy, and survival rates are generally 90 % or higher when diseases are detected at early stages, making it one of the most curable forms of cancer [95]. The primary therapeutic modalities are CH and RT, as surgery does not often play a role in the management of this disease. Accurate imaging is crucial to treatment strategy, and FDG-PET has become a useful imaging modality in the staging and treatment evaluation algorithm for lymphoma by providing unique metabolic information.
Most of the existing evidence in the literature on the contribution of PET/CT in lymphoma staging comes from studies performed in Hodgkin’s lymphoma (HL). PET/CT proved to be more sensitive and specific than other conventional modalities, including CT, for the evaluation of extent of LN and extra-LN disease. Stage variation occurs in nearly 25 % of patients, mostly to upstage disease, leading to a change in treatment strategy in nearly 10–15 % of them [96]. The role of a contrast-enhanced CT (CECT) performed simultaneously in the same diagnostic session with the PET scan is still a subject of debate. PET/CECT may be useful in patients with abdominal and pelvic involvement for delineating LN from adjacent bowel loops and vasculature [97].
Prognostic value of PET/CT in baseline staging also has been reported in follicular lymphoma (FL), which is the most common indolent (slow-growing) form of non-Hodgkin’s lymphoma (NHL) (grade 3 more specifically). Le Dortz et al. found that a score value ≥2, whatever the Follicular Lymphoma International Prognostic Index (FLIPI) score, was the only predictive factor in multivariate analysis on treatment outcome.
In conclusion, PET/CT is strongly recommended before treatment onset for patients in routine staging workup in most lymphoma subset with the exception of marginal extra-LN lymphoma, cutaneous lymphoma, and small lymphocytic lymphoma [98]. In HL, FDG-PET enables an early evaluation of the metabolic changes occurring during the induction treatment as early as after the first [99], the second [100–103], and the third [104] cycle of CH (interim PET). In this regard, PET/CT yielded highly promising results as a surrogate of chemosensitivity for predicting tumor response and progression-free survival (PFS), with a sensitivity and specificity of 43–100 % and 67–100 %, respectively [105], even superseding the prognostic role of the International Prognostic Score [106].
In April 2009 at a workshop on interim PET in lymphoma, simple and reproducible rules have been proposed for interim PET visual interpretation [107, 108], and these criteria have been recently retrospectively validated [109]. Briefly, the adopted rules include the following statements:
- (i)
visual assessment is preferred, but SUV determination can be used in some cases
- (ii)
interim-PET interpretation should always be made by comparing the single foci of FDG uptake to those recorded in the baseline study
- (iii)
intensity of FDG uptake should be graded according to a five-point scale in which two reference organs, the mediastinal blood pool structures and liver, are used to define different grades of FDG uptake
Two recent meta-analysis, reviewing 34 studies comprehensive of 882 HL and 604 diffuse large B-cell lymphoma (DLBCL-NHL) patients, showed that FDG-PET performed after treatment is highly predictive of treatment outcome in terms of PFS and OS both in HL and DLBCL patients with and without residual masses, with a higher sensitivity for HL and a higher specificity for DLBCL [110, 111]. Despite the good response to therapy, a residual mass can be demonstrated by radiological means in up to 80 % of HL and up to 40 % of NHL patients after completion of treatment [112, 113], even if only less than half of these masses will harbor residual disease. In advanced-stage HL, before the advent of functional imaging for treatment response evaluation, involved field RT was proposed for LN lesions or residual masses, as an integral part of a combination CH for HL, in particular ABVD (doxorubicin, bleomycin, vinblastine, and dacarbazine) treatment [114].
More recently, PET/CT performed at the end of treatment for lymphoma proved effective in discriminating residual active disease from fibrotic masses, with a sensitivity of 43–100 % and a specificity of 67–100 % [111]. Not surprisingly, the negative predictive value (NPV) of the end-treatment PET depends on the efficacy of the administered CH, as high as 94 % after an aggressive regimen, such as bleomycin, etoposide, doxorubicin, cyclophosphamide, vincristine, procarbazine, and prednisone (BEACOPP) escalated in advanced-stage HL or as low as 75 % after the low-intensity regimen vincristine, epidoxorubicin, bleomycin, cyclophosphamide, etoposide, prednisone (VEBEP) [115]. Another relevant role played by post-CH PET scan is to guide RT both in HL and DLBCL. Accurate TVD has become an essential component of radiation field design, owing to treatment strategies that now incorporate reduced radiation field sizes and targeted conformal therapies [116, 117]. In particular, radiation using involved node RT (INRT) or involved site RT (ISRT) restricts RT to the initially involved LNs with a margin, thus requiring more precision in identifying involved disease. FDG-PET information for defining target volumes for RT planning has also drawn great interest and has significant value in monitoring response to therapy and predicting outcomes for patients with lymphoma. Pretreatment PET is required for the application of ISRT or INRT principles to early-stage HL and NHL [116, 118, 119], even if it is unfortunately often logistically difficult to acquire a PET study in pre-CH phase. ISRT similarly restricts RT to the initially involved LN but allows for a larger margin to account for uncertainties [116].
Multiple studies have demonstrated that target volume alterations can occur when PET is obtained at the time of simulation for HL. Few studies, however, have specifically examined PET for radiation planning in a predominantly NHL population including other hematologic malignancies. The implications on TVD may differ for HL and NHL. Most studies examining the use of PET for radiation planning in HL have fused pretreatment PET scans obtained in the treatment position to posttreatment CT simulation scans because PET is often negative at the completion of CH. In NHL, patients are often referred for definitive therapy; in that case, they are likely to have positive PET at time of radiation. Although sensitivity and specificity of PET is well defined in HL, its utility is more controversial for low-grade NHL [120–122].
Consistent data from multiple studies have established the role of post-therapy FDG-PET imaging for the prediction of recurrent HL and DLBCL [123]. An NPV and a positive predictive value (PPV) of 80 and 100 % were reported, respectively, for FDG-PET in the identification of residual aggressive NHL after completion of first-line CH [124, 125]. More recently, it was emphasized that end-of-treatment PET was highly predictive of PFS and OS, regardless of interim PET result in early-stage HL and performed better than interim PET in the low-risk HL population [101, 126]. The revised IWG response criteria incorporated FDG-PET to accurately assess end-therapy persistent masses in both NHL and HL. Filippi et al. investigate the role of RT in patients affected with primary mediastinal B-cell lymphoma (PMBCL) with residual FDG-PET-positive disease after rituximab CH, and their result indicates that approximately 50 % of PMBCL patients show residual disease at FDG-PET scan after CRT. RT is able to convert to CR approximately 85 % of these patients, but those with a Deauville score of 5 (10 %) appear at high risk of progression and death, and they might be candidates for intensified programs [127].
22.1.6 Myelomas
Multiple myeloma (MM) is a hematologic neoplasm characterized by bone marrow invasion of a neoplastic clone of plasma cells, presence of bone lytic lesions, and extramedullary organ invasion in later stages of the disease. The disease course is heterogeneous with a patient survival ranging from a few months to more than a decade. In this perspective, a still unfilled need exists for reliable prognostic tool identification in order to predict the individual disease outcome in a single-patient basis. As in other solid and hematologic cancers, one of the most important prognostic factors is tumor dissemination (or stage) at baseline; the most frequently involved organ is the bone and bone marrow [128, 129].
The MM staging system was introduced in 1975 by Durie and Salmon to allow patient stratification with different tumor bulk by calculating the measurable theoretical myeloma cell mass. MM is yet incurable, and local therapies are frequently used to treat MM-induced lesions in the palliative setting and solitary osseous or extra-osseous plasmacytomas in the definitive setting. RT is used to treat bone pain, to manage areas at risk for pathologic fracture, and to sterilize residual disease after surgery. MM is highly responsive to RT owing to its radiosensitivity, with clinical response rates as high as 97 % [130–132]. However, the emergence in the last decade of new imaging techniques, such as FDG-PET/CT and MRI, has provided new insights on tumor spread at disease onset and gradually revolutionized staging system developing a more integrating clinical and imaging approach, the Durie/Salmon PLUS myeloma staging.
Sensitivity of FDG-PET/CT proved very high for detection of extraskeletal lesions, while MRI outperforms FDG-PET/CT for detecting bone and bone marrow invasion by disease, especially for spine and pelvic localizations. PET/CT detected bone lesions mainly on the basis of their increased metabolic activity instead of any anatomical alteration. Moreover, it provided an accurate identification of tumor viability that is useful for treatment planning and monitoring. FDG-PET/CT proved a powerful prognosticator in MM: metabolic target volume measurement at baseline and longitudinal studies aimed at assessing the role of minimal residual disease detection by FDG-PET/CT are the most innovative aspect of functional imaging in this disease [133].
Solitary plasmacytoma (SP) is potentially curable malignant neoplasms of plasma cell lineage, often accompanied by a serum paraprotein (PP) or by urinary free light chain (Bence-Jones protein) excretion. They comprise approximately 10 % of all plasma cell tumors, occurring as either solitary bone plasmacytoma (SBP) or as extramedullary plasmacytoma (EP) [134, 135]. Although RT can result in local control rates in excess of 80 % in both SPB and EP [136, 137], MM will ultimately develop in 50–70 % [138–141] and <50 % [135, 140, 142, 143] of patients with SBP and EP, respectively. The majority of SBP will progress to MM within 3–4 years [144]. Prognostic factors for progression of SBP to MM include older age [145], increasing tumor size [146], and persistence of PP after RT [147].
The frequent progression of SP to MM after definitive RT suggests that the staging workup has been insufficiently sensitive to detect occult systemic disease at presentation. FDG-PET/CT can play a useful role in the evaluation of patients with SP who are candidates for definitive RT or who have been treated with RT (Fig. 22.7). PET influenced the initial clinical management in 35 % of patients who underwent staging PET scans as part of their workup before definitive RT. There is some indication in the literature that PET may be useful for response assessment in MM, but there is little information on response assessment on SP [148]. Persistence of FDG uptake for some months after RT in an irradiated SP does not necessarily indicate a bad prognosis or by itself constitute an indication for further treatment. In the absence of new lesions or a rising PP level, a period of observation may be the most appropriate course of action. PET can reliably image known deposits of malignant plasma cell disorders and can image new sites of disease that are not apparent using other imaging modalities such as CT or MRI [149].
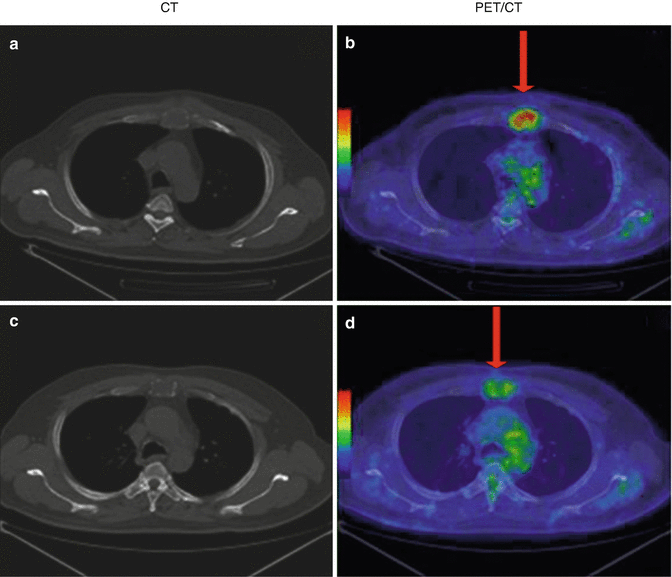
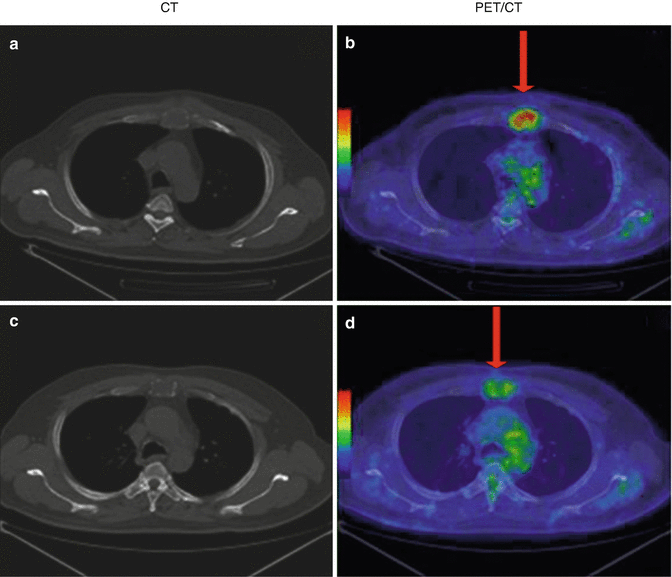
Fig. 22.7
Patient with sternal solitary bone plasmacytoma undergone to radiotherapy treatment (30 Gy/10 fractions). The comparison between CT and PET/CT before (a, b) and after (c, d) radiotherapy treatment showed minimal residual uptake (red arrow) on the treated lesion
Schirrmeister et al. [150, 151] reported that PET influenced patient management in 4 (27 %) of 15 cases of SP, by changing diagnosis or by accurately restaging disease. In patients with SP and negative PP levels, biochemical studies may not be useful for monitoring disease status after local RT. In a rise of PP after therapy, imaging may distinguish local from distant disease progression. PET/CT images can be directly imported into planning systems for contouring at the RT workstation, facilitating 3D BTV determination. PET can provide clear images of the extent of soft tissue extension of SP, even when the tumor edge is isodense with surrounding normal tissue on CT, and may be the best modality for planning treatment of a boosting dose of RT to the GTV after an initial wider field treatment. Most series report the use of doses in the 40–50 Gy range.
The radiation target volume for SP typically includes the whole affected bone and any soft tissue extension. It is common practice to treat a smaller volume around the GTV with a boosting dose after an initial dose has been given to a wider field. There is evidence for a dose-response effect for local control. Frassica et al. [138] reported a series in which 11 % of SPB cases developed local recurrences following treatment with a median dose of 39.75 Gy, but no cases of local failure occurred if ≥45 Gy was used. Mendenhall et al. [152], in a review of 81 cases, reported a 94 % local control rate with ≥40 Gy vs. a 69 % control rate with doses <40 Gy.
22.1.7 Brain Tumors
Brain tumors include all tumors that arise inside the cranium, especially gliomas, which are inherently serious and life-threatening because of their invasive and infiltrative character in the limited space of the intracranial cavity. Surgery, CH, and RT are the main treatment modalities for gliomas. RT as a primary treatment modality is mainly reserved for the inoperable cases; however, it is often employed in an adjuvant setting, particularly if there is residual tumor left behind after surgical resection.
RT continues to play an important role in the treatment of brain tumors. As an initial step in RT planning, patients undergo a CECT scan in the treatment position. MRI has been used to improve the accuracy of outlining tumors owing to the superiority of MR in identifying intracranial anatomic structures.
Standard planning for RT relies on both gadolinium-enhanced T1-weighted (T1-Gad) and T2-weighted fluid-attenuated inversion recovery sequences to image the contrast-enhancing portion of the tumor and peritumoral edema, respectively. By introducing MR fusion to CT planning, MR increased the clinical target volume (CTV) compared with that seen on CT and felt that the integration of MR data with CT information was of significant practical value in treatment planning. In the RT of high-grade gliomas, the target volume is identified using postoperative CT and MRI with intravenous contrast. The area of T1-Gad MRI is often used to define the extent of GTV radiologically. However, several pathologic studies have shown that the contrast enhancement may not represent the outer tumor border because infiltrating glioma cells can be identified well beyond the area of enhancement [153]. Gadolinium contrast enhancement is also a nonspecific sign of blood-brain barrier disruptions and cannot accurately differentiate nonspecific postsurgical changes from residual tumor [154].
Normal brain cells consume large amounts of glucose due to their high metabolic demand; therefore, FDG-PET alone is not suitable for brain tumor evaluation. Multimodality images including MRI or CT and FDG-PET are essential for accurate evaluation of brain tumors. However, due to increased proliferation and protein synthesis, brain tumors also have increased amino acid uptake and upregulated amino acid transporters. Radiolabeled amino acid and its analogs are a class of tumor imaging agents that may be more suitable for tumors with high FDG background imaging.
PET imaging with MET, FLT, and CHO is a good option for functional imaging in brain tumor RT planning. MET, 18F-fluoro-ethyl-tyrosine (FET), and 18F-3,4-dihydroxy-6-18F-fluoro-l-phenylalanine (FDOPA) have been comparatively studied and produce very similar quality images for brain tumors [155], except that there is normal physiologic uptake of FDOPA in the basal ganglia. Uptake of MET and FET does not accumulate in normal anatomic structures of the brain. In a prospective study of the treatment of primary glioblastomas, poor coverage of MET uptake (which was not used for TVD) was associated with an increased risk of noncentral recurrence [156]. However, a prospective study of patients treated using FET-PET target volumes showed the observed pattern of failure to be predominantly central [157].
Uptake of FET has been used to delineate radiation boost volumes. In a phase II trial of dose escalation for glioblastomas, a simultaneous integrated boost of 72 Gy in 30 fractions delivered to an FET-PET-defined PTV, concurrent with 60 Gy in 30 fractions delivered to a traditional MRI defined PTV, did not lead to a survival benefit [158]. In a retrospective series relating the results of MRI and FET-PET to the outcome of biopsy, Pauleit et al. found a higher sensitivity and specificity of combined MRI and FET-PET for biopsy selection as compared to MRI only [159]. Data previously published by Niyazi et al., showed that targets delineated by means of FET uptake were 30 % larger than those solely based on MRI [160]. Piroth et al. conducted a phase II trial investigating the possible benefit of FET adapted local dose escalation [161]. In their cohort (n = 22), the FET-PET volume was almost twice as large as the Gad-enhanced MRI volume. Both groups used fixed LN thresholds of 1.5 and 1.6, respectively. Moreover, Grosu et al. published data of improved but enlarged target volumes for both meningioma and glioma when integrating MET-PET into the planning procedure [162].
Creating the PTV without integrating FET-PET would have resulted in missing a mean volume proportion of 17 % of the FET-positive tissue. However, to date, there is no evidence from prospective randomized and controlled trials that inclusion of this volume would convert into a clinical benefit. If all FET-positive lesions are considered to be tumor tissue not visible in MRI, it is essential to include these regions into the target volume. Knowing that most glioma recurrences are located within or at the margin of the initial RT field, this finding may emphasize the importance of conducting FET-PET/CT prior to RT. Currently, a randomized controlled trial evaluating MRI-based contouring in comparison to FET-PET for recurrent glioma is being planned under the auspices of the German Neuro-Oncology Group.
Biologically based treatment planning with FDOPA-PET may also be possible because there is a correlation between FDOPA uptake, cell density, and cell proliferation in newly diagnosed tumors [163]. FDOPA provides equivalent visual and quantitative SUV information to MET but offers a significantly longer physical half-life of 18F (109 min) compared with 11C (20 min). This is significant, as 11C tracers can be used only at institutions with an on-site cyclotron, limiting their use to essentially large academic centers and not generally applicable in practice. Normal uptake of FDOPA in the basal ganglia can present challenges when trying to distinguish between normal brain and adjacent tumor, which is absent with FET-PET uptake. Pafundi et al. reported that FDOPA-PET may more accurately identify regions of higher-grade/higher-density disease in patients with astrocytoma and will have utility in guiding stereotactic biopsy and RT targeting [164]. Future studies will investigate the role of FDOPA-PET with relative cerebral volume in perfusion MRI for targeting the most aggressive disease components and to determine whether biologically based treatment planning with FDOPA-PET/CT can improve outcomes for high-grade gliomas.
Finally, the amino acid PET tracer alpha-[11C]methyl-L-tryptophan (AMT) is transported in tumor tissue by the L-type amino acid transporter but is not incorporated into proteins [165]. Instead, AMT is utilized by the kynurenine immunomodulatory pathway [166, 167]. Under pathologic conditions, induction of this pathway’s rate-limiting enzyme, indoleamine 2,3-dioxygenase, leads to increased metabolism of tryptophan and thus AMT accumulation via the kynurenine pathway [168, 169]. This pathway plays an important role in brain tumor pathogenesis [170]. Christensen et al., found that retrospectively created GTVs based on preoperative AMT-PET, either alone or in conjunction with postoperative MRI findings, demonstrating significant discordance with the standard clinical high-risk GTV generated via T1-Gad findings alone. Importantly, there was no significant difference in volumetric size of the GTVs determined by AMT-PET relative to those determined by MRI alone. Despite this, the investigational AMT volume offered superior coverage of the volume of initial progression when compared to the standard MRI-based GTV. Ideally, increased accuracy of GTV definition would allow for smaller empiric safety margins for microscopic spread that determines the CTV.
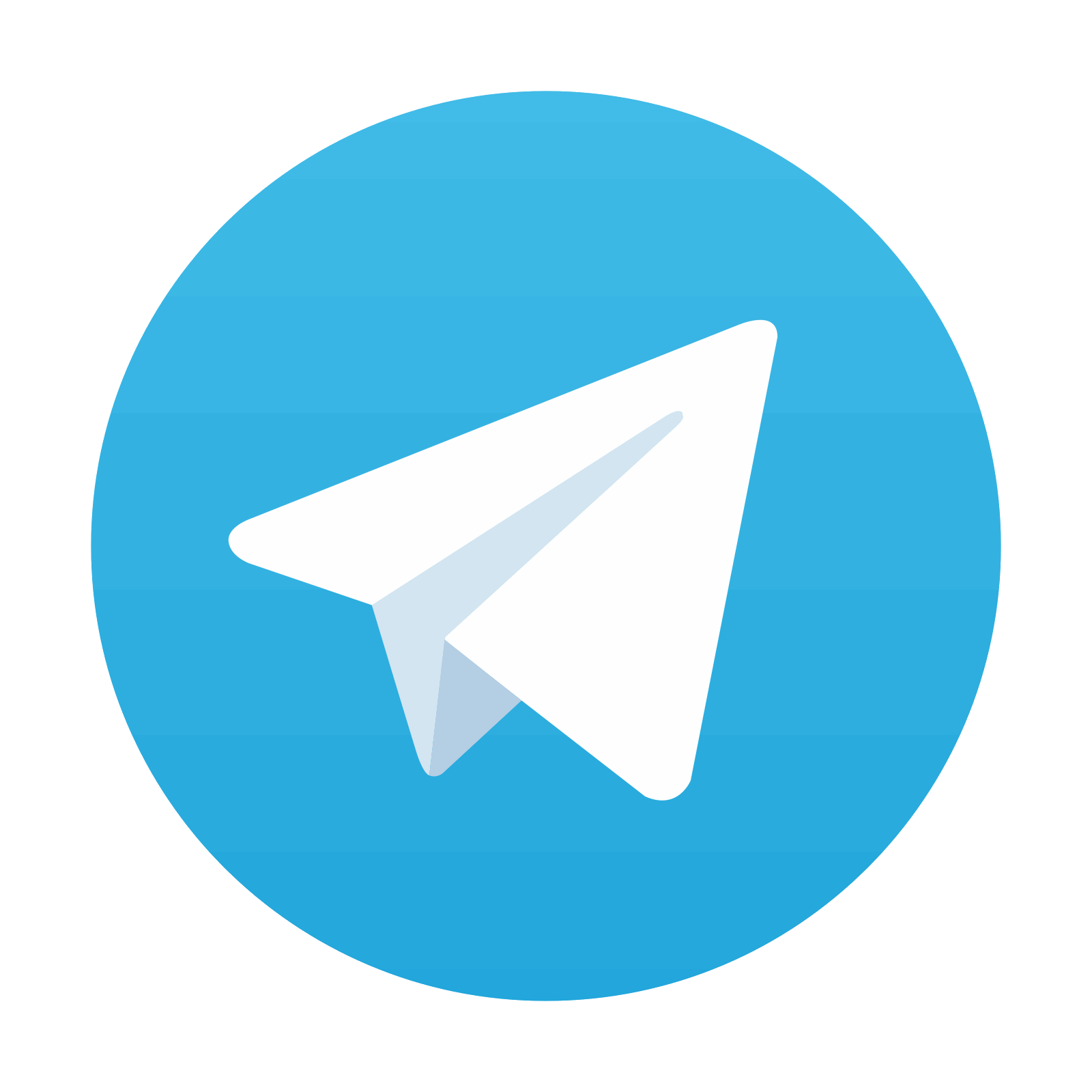
Stay updated, free articles. Join our Telegram channel

Full access? Get Clinical Tree
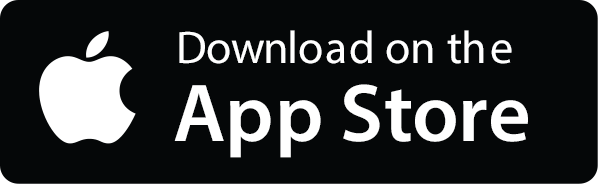
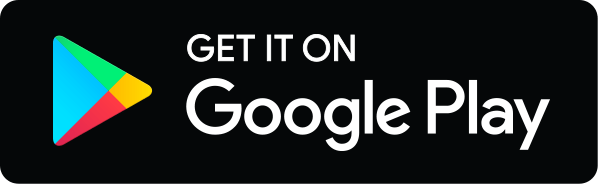