SECTION XIV PHYSICS SAFETY
Essentials 1
Case
Radionuclide Y (atomic number Z) decays by β2 decay, resulting in the production of a metastable daughter state. The metastable daughter nucleus then transforms to the ground state by isomeric transition. A sample containing both the parent and daughter states is examined. Which of the following is likely to be present in the sample?
A. A β2 particle at a single discrete energy
B. A range of high-energy γ-rays emitted by radioisotope Y
C. A daughter state with Z + 1 protons
D. X-rays with a range of continuous energies emitted by the daughter state
E. Only one daughter state at a single energy level
Answers and Explanations
C. Correct! In β2 decay, a neutron is converted to a proton, and therefore, the Z (atomic number) increases by one; thus, the daughter is a different element than the parent (“transmutation”). The mass number (A) does not change. Therefore, the parent and daughter are isobars. An example is 133Xe’s β2 decay to 133Cs.
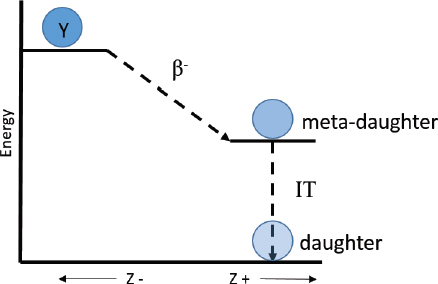
Other choices and discussion
A. The decay of the parent radioisotope will result in the emission of a family of β2 particles of varying continuous energies up to Eβ max. Typically, the average β2 particle energy, Eβ, will be approximately one-third of Eβ max.
B. In β2 decay, the parent emits a beta-particle (β2) and an anti-neutrino () as it decays to the daughter state.
D. In isomeric transition, the metastable daughter nucleus will emit characteristic, discrete gamma rays (γ-rays) rather than a family of γ-rays with continuous energies. Frequently, a metastable nucleus will emit both γ-rays and conversion electrons; the frequency of each type of emission (e/γ ratio) is characteristic for each isotope. Like the γ-rays, these conversion electrons will have discrete energies (based on their position in the electron cloud—i.e., the shell from which they were ejected).
E. As noted in A, the decay of the parent will result in the emission of numerous β2 particles at varying energies, resulting in several daughter states with varying energies.
Suggested Readings
Patton JA. Introduction to nuclear physics. Radiographics 1998;18:995–1007 Saha GB. Physics and radiobiology of nuclear medicine. 4th ed. New York: Springer; 2014Top Tips
β2 decay → neutron converted to proton → Z + 1 → parent and daughter are isobars (same mass number, different atomic number).
β2 decay → parent emits a beta-particle (β2) and an anti-neutrino ().
The average β2 particle energy, Eβ, will be approximately one-third of Eβ max.
Essentials 2
Case
Which of the following characteristics of alpha (α) decay limits the use of alpha emitters for diagnostic applications?
A. α-particles interact minimally with solid materials, such as those found in radiation detectors.
B. α-particles are typically of very low energy.
C. α-decay most often occurs in light (low mass number) elements that are not easily incorporated into radiopharmaceuticals.
D. α-particles have a short range in tissue.
E. α-decay produces daughter nuclides that cannot be chemically separated from the parent.
Answers and Explanations
D. Correct! As heavy particles, alpha (α) particles undergo numerous interactions with surrounding matter, and as such, continuously lose small amounts of energy causing them to slow down as they travel only a relatively short distance—typically < 100 µm in solids (β1 particles from 82Rb, in contrast, have a mean range of ~2.5 mm).
Other choices and discussion
A. Alpha (α) particles have two protons (Z = 2) and two neutrons (A = 4), and are essentially a helium nucleus. They have a high linear energy transfer and deposit substantial energy over a very short range via their interactions with surrounding matter. Given this high local deposition of energy, α-emitting radiopharmaceuticals have been viewed as promising for targeted irradiation of tumor cells.
B. Alpha (α) particles are typically of very high energy, oftentimes more than 3 to 5 MeV.
C. Alpha (α) decay, like fission, typically occurs in heavy isotopes such as uranium (238U, where Z = 92) and radium (223Ra, where Z = 88). Difficulty in producing α-emitting radiopharmaceuticals has been mainly related to the radiolytic effects of clinically useful α-particle doses which produce challenges to traditional radiochemistry methods.
E. Daughter nuclides produced by α-decay have an atomic number of Z – 2 and a mass number of A – 4 as compared to the parent radionuclide. As such, the daughter is typically chemically distinct from the parent, and at low activities, can be separated by standard methods such as resin-based elution.
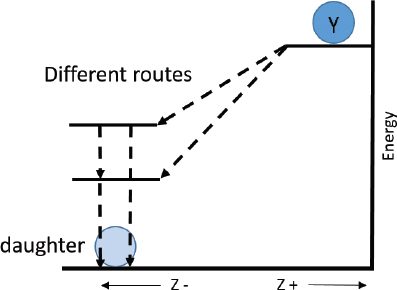
Suggested Readings
Cherry SR, Sorenson JA, Phelps ME. Physics in Nuclear Medicine. 4th ed. Philadelphia: Saunders; 2012 De Kruijff RM, Wolterbeek HT, Denkova AG. A critical review of alpha radionuclide therapy—how to deal with recoiling daughters? Pharmaceuticals 2015;8:321–336 Huclier-Markai S, Alliot C, Varmenot N, et al. Alpha-emitters for immune-therapy: a review from chemistry to clinics. Curr Top Med Chem 2012;12:2642–2654Top Tips
Alpha (α) particles have two protons (Z = 2) and two neutrons (A = 4), and are essentially a helium nucleus. Daughter nuclides produced by α-decay have an atomic number of Z – 2 and a mass number of A – 4 as compared to the parent radionuclide.
Given their ability to deliver high doses of radiation to a small area (high linear energy transfer) when appropriately targeted, α-emitting radiopharmaceuticals show great promise for radiotherapeutic applications and have achieved recent success on a limited basis.
While the α emissions of the parent may be favorable due to the short path length, and therefore limited “crossfire” radiation, the production of excited daughter states that may add to the radiation dose/damage has remained a concern and will be of particular interest in the future.
Essentials 3
Case
A charged particle is found to have a high linear energy transfer (LET) in a tissue. What would you conclude about the particle?
A. The particle produces a high specific ionization (SI) in the tissue.
B. The particle must be a neutron.
C. The particle produces mainly single-strand deoxyribonucleic acid (DNA) breaks in the tissue.
D. The particle likely has a very high velocity as it travels through the tissue.
E. The path of the particle in the tissue is long and crooked.
Answers and Explanations
A. Correct! LET refers to the amount of energy deposited in matter (e.g., in tissue) per unit of length along the path of an incident radiation. SI is defined as the average number of ionization events per unit of radiation path length. SI is related to LET by the following relationship: LET = SI/W, where W is the average energy expended in the production of an ionization. Thus, as a radiation moves along its path, deposited energy will produce ionizations, and as such, a particle that produces many ionizations (high SI) would be expected to have a high LET.
Other choices and discussion
B. Neutrons (n), alpha (α) particles, and protons may have high LETs and are considered to be heavy particles with mass energies greater several orders of magnitude greater than an electron (a light particle). Electrons (including β-particles) are small particles with low LET. Electromagnetic radiations, though not particulate in nature, also exhibit low LET.
C. High LET radiation produces frequent double-strand DNA breaks that are difficult for the cell or tissue to repair effectively. Low LET radiation produces fewer double-strand DNA breaks and is associated with less radiation-induced cell death.
D. A particle with a high LET, by definition, deposits a high amount of energy into the local tissues. This deposition is a property of the tissues (higher density tissues will provide more atoms available for interaction in a given mass of tissue than would less dense tissues) and of the particle or incident radiation. A particle traveling at a high velocity through a tissue would be expected to spend only a small fraction of time in the vicinity of any given atom, and therefore would have a low likelihood of interacting and causing an ionization of that atom. In contrast, a particle traveling at a low velocity through a tissue would have a higher likelihood of interacting with and depositing energy resulting in an ionization.
E. Particles that deposit energy locally lose speed (energy) as they do so. Typically, these particles travel a short distance along a fairly straight path until they have lost all of their energy to the surrounding tissues. Small particles, such as β-particles, are more likely to interact with multiple atoms in the tissue, having a long and tortuous path as each interaction causes the loss of a small amount of energy and deflects the electron from its incident path angle.
Suggested Readings
Cherry SR, Sorenson JA, Phelps ME. Physics in Nuclear medicine. 4th ed. Philadelphia: Saunders; 2012 Kassis AI, Adelstein SJ. Radiobiologic principles in radionuclide therapy. J Nucl Med 2005;46:4S–12S Saha GB. Physics and Radiobiology of Nuclear Medicine. 4th ed. New York: Springer; 2014Top Tips
For a given amount of kinetic energy, alpha (α) particles may ionize hundreds of times more atoms in the local tissue than electrons (including β-particles) with the same kinetic energy.
Both particulate and electromagnetic incident radiations transfer energy to tissues and can result in tissue ionizations.
Essentials 4
Case
An incident radiation (γ-ray) interacts with an atom as shown below. The labeled electron is _______________ and thus, the atom will be _______________.
A. a secondary electron; in an excited state
B. an Auger electron; in an excited state
C. a photoelectron; in an ionized state
D. a β2 particle; more stable than the original atom
E. a β1 particle; an isotope of the original atom
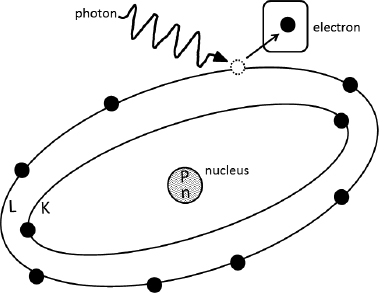
Answers and Explanations
C. Correct! Ionization of an atom has occurred when an orbital electron has been removed or separated from the atom, leaving the atom in an unstable ionized state. The electron that is lost from an atom as a result of a collision with a photon is called a photoelectron. (Strictly speaking, the photoelectron is a specific type of secondary electron, as it is separated from the atom by the incident [primary] radiation, which in this case is a photon.)
Other choices and discussion
A. A secondary electron lost by interaction or collision with an incident radiation is called a secondary electron, to differentiate it from the incident (primary) radiation; the electron in this example is a secondary electron. By definition, excitation of an atom occurs when an electron is raised to a higher energy state within its orbital shell; the electron is not lost from the atom in excitation event.
B. An Auger electron is emitted when an electron in a higher orbital shell transitions to fill a vacancy left by an electron being removed from a lower orbital shell (typically due to the process of internal conversion). When the excess energy of the transition is then transferred to an orbital shell electron, that electron is emitted; this is emitted electron is the Auger electron. Note that, as an alternative to emission of an Auger electron, the excess transition energy may instead result in the release of a characteristic X-ray. By definition, excitation of an atom occurs when an electron is raised to a higher energy state (from a lower energy shell to an upper energy shell); the electron is not lost from the atom in excitation event.
D. A β2 particle is a form of particulate radiation, not electromagnetic radiation (i.e., it is not a photon). As shown in the diagram, the atom has lost an electron, leaving it with a net positive charge in an ionized state. Ionized states are not more stable than their nonionized form (ground state).
E. A β1 particle is a form of particulate radiation, not electromagnetic radiation (i.e., it is not a photon). In the example shown, no nuclear changes have occurred (the number of protons and neutrons in the nucleus has not changed), thus this atom has simply been ionized. By definition, isotopes are nuclides with the same proton number (Z) but different mass numbers. Examples of clinically relevant isotopes are 68Ga and 67Ga, which both have Z = 31, but have different numbers of neutrons and therefore different mass numbers.
Suggested Readings
Cherry SR, Sorenson JA, Phelps ME. Physics in Nuclear Medicine. 4th ed. Philadelphia: Saunders; 2012 Saha GB. Physics and Radiobiology of Nuclear Medicine. 4th ed. New York: Springer; 2014Top Tips
Photoelectrons and other secondary electrons are ejected from an atom by its collision with an incident radiation.
Other types of emitted orbital electrons (e.g., Auger) typically result from an ionized atom transitioning to its ground state rather than the direct interaction with an incident radiation.
Essentials 5
Case
Neutral stable phosphorus has an atomic number of 15 (Z = 15) and a mass number of 31 (A = 31). Based on the Bohr model of the atom, what is the principal quantum number of the energy shell in which the outermost electrons are likely to be found?
A. 0
B. 1
C. 2
D. 3
E. 4
Answers and Explanations
D. Correct! Each electron shell is designated a principal quantum number (n), beginning at 1 for the K-shell. Thus, the L-shell has a principal quantum number of 2, the M-shell has a quantum number of 3, etc. Each shell can house a maximum of 2n 2 electrons, and electrons will fill the lowest shell first. Once the K- and L-shells contain the maximum number of electrons, electrons will then fill the M-shell, which has a quantum number of 3 and can contain a maximum of [2 × (3)2] = 18 electrons. Thus, in a stable atom with 15 protons and 15 electrons, the outermost electrons are expected to fill into the 3p orbital, where the principal quantum number of 3 represents the M-shell.
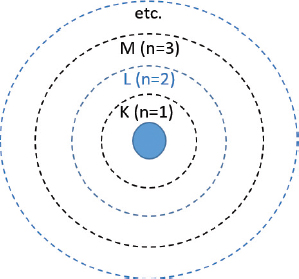
Other choices and discussion
A. There is no electron shell with a principal quantum number of 0.
B. In a stable atom with 15 protons, there will be 15 electrons within the electron cloud. These electrons will first fill the lowest shell—the K-shell, which holds a maximum of 2 [2 × (1)2] electrons, thus leaving 13 electrons within the higher-level shells. Thus, the outermost electrons will not be in the K (quantum number of 1) shell.
C. Once the K-shell contains the maximum number of electrons, the electrons will then fill the L-shell (which has quantum number of 2, and can contain a maximum of [2 × (2)2] = 8 electrons). In a stable atom with 15 protons and 15 electrons, there will, therefore, be 15 − (2 + 8) electrons left to reside within a higher-level shell.
E. The N-shell (with a quantum number of 4 and a maximum number of contained electrons of [2 × (4)2] = 32) will only be filled once the M-shell contains its maximum number of electrons. Thus, for a stable atom with 15 protons and 15 electrons, we would not expect any electrons in the N-shell as they would have all been accommodated by the K-, L-, and M-shells.
Suggested Readings
Saha GB. Physics and Radiobiology of Nuclear Medicine. 4th ed. New York: Springer; 2014Top Tips
Each electron shell is designated a principal quantum number (n), beginning at 1 for the K-shell.
Each electron shell can house a maximum of 2n 2 electrons, and electrons will fill the lowest shell first.
Essentials 6
Case
In transporting a 200 mCi sample of radionuclide Q-labeled pharmaceutical in a capped plastic syringe to the imaging suite, the investigator falls and drops the sample—spilling most of it on the hallway floor. Based on the activity and primary emission (210 keV γ-rays) of the radionuclide, this is deemed to be a major radioactive spill. Unlike a minor spill, clean-up and precautions for a major spill necessarily include:
A. Notification of personnel and other persons in the area that a spill has occurred and restricting access to the spill area.
B. Covering the spill with absorbent paper, when applicable, to prevent spread of the material or contamination of other surfaces.
C. Survey of the spill area and areas of potential contamination with a survey meter or other appropriate detector.
D. Immediate notification of the radiation safety officer (RSO).
E. Decontamination of affected individuals.
Answers and Explanations
D. Correct! The distinction between major spills and minor spills depends largely on the amount and type of radionuclide (internal radiation hazard) involved. In addition, major spills are often considered to be those spills that are not easily contained or containable by standard measures or interventions. In this case, the spill is deemed to be a major spill, and therefore precautions for a major spill should be followed. In addition to the other precautions listed, which should be followed for all spills whether they are minor or major, major spills require the immediate reporting of the incident to the local RSO. The RSO may make recommendations regarding clean-up and modifications to standard procedures to prevent or lessen the impact of future spills. For minor spills, the RSO may not need to be notified—particularly not immediately—unless there were unusual circumstances surrounding the spill.
Other choices and discussion
A. Following any spill of radioactive material in a nuclear medicine or radiology department, persons in the area surrounding the spill should be notified of the spill and prevented from entering the area. In this example, physicians, nurses, technologists, patients, and others might be prevented from utilizing the section of the hallway where the spill has occurred until proper decontamination has occurred and surveys indicate that the area poses no hazard.
B. As most radiopharmaceuticals are administered intravenously and in liquid form, covering the spill with absorbent paper is often a good first step in minimizing its impact. This can serve not only as a visual alert that something has been spilled, whether on the floor or on a countertop, but can be used to remove the spilled material from the surface. If the spilled material is in powder or gas form, efforts to limit its spread might include the closing of nearby or adjacent doors. For spills on the floor, careful attention should be paid to surveying, and if needed, decontaminating the shoes of personnel involved in the spill and spill clean-up, to prevent inadvertent contamination of other areas.
C. In assessing the degree of spread or potential contamination following any radioactive spill, a survey meter or other device should be used to determine how large the spill area is and the extent to which any radioactive material has been transferred to other surfaces. For example, nearby door handles, floors, and equipment may be surveyed to ensure that material has not been transferred. Identification of affected (contaminated) personnel or other persons is of first priority, and survey meters may be used to detect spilled material on the clothes or skin of those who may have been exposed.
E. As suggested above, the identification and decontamination of affected persons is the first priority after any radioactive spill. The Nuclear Regulatory Commission requires that all radioactive materials licensees have procedures in place for the handling of radioactive spills.
Suggested Readings
Baldwin JA, Bag AK, White SL, Palot-Manzil FF, et al. All you need to know as an authorized user. Am J Roentgenol 2015;205:251–258 Siegel JA. Nuclear Regulatory Commission Regulation of Nuclear Medicine: Guide for Diagnostic Nuclear Medicine. Reston, VA: Society of Nuclear Medicine; 2001Top Tips
Classification of a spill as either major or minor depends on the radionuclide involved and the amount of spilled activity. For example, minor spills of radionuclides labeled with 99mTc are those where the spilled activity is < 100 mCi. Conversely, spills of ≥ 10 mCi of radionuclides labeled with 67Ga or 111In are considered major spills, and spills of only 1 mCi of 131I-labeled radionuclides are considered major spills.
Major spills require the immediate reporting of the incident to the local RSO.
Essentials 7
Case
In the United States, members of the general public receive the highest annual dose of radiation (per capita) from which of the following sources?
A. Diagnostic X-rays
B. Cosmic radiation from the sun
C. Ingested isotopes including potassium-40
D. Thorium-232 and radium-226 within terrestrial material
E. Inhalation of radon in the air
Answers and Explanations
A. Correct! Based on data from the United Nations (United Nations Scientific Committee on the Effects of Atomic Radiation) 2008, diagnostic X-rays resulting from medical procedures such as computed tomography, radiography, fluoroscopy, and dental radiographs contribute approximately 2.4 mSv to the annual effective radiation dose to the general public. This data is consistent with, though with very slight differences, data on background and medical sources of radiation as published in the 2009 National Council on Radiation Protection and Measurement Report 160. You may refer to the NIS section for additional discussion.
Other choices and discussion
B. Cosmic rays deliver approximately 0.3 mSv of effective radiation dose annually, with the amount varying somewhat with altitude.
C. Ingestion of foods and water results in the internal deposition of compounds that emit radioisotopes such as 40K. These internal compounds expose members of the general public to about 0.3 to 0.4 mSv effective dose per year.
D. Terrestrial radiation sources expose the general public to approximately 0.4 to 0.5 mSv of radiation dose annually.
E. Inhaled or airborne radon is the most abundant source of natural background radiation, accounting for approximately 1.2 to 2 mSv effective dose per year.
Suggested Readings
Mettler FA, Bhargavan M, Faulkner K, et al. Radiologic and nuclear medicine studies in the United States and worldwide: frequency, radiation dose, and comparison with other radiation sources—1950-2007. Radiol 2009;253:520–531 National Council on Radiation Protection. NCRP Report No. 160. (2009). Ionizing radiation exposure of the population of the United States. http://ncrponline.org/publications/reports/ncrpreport-160/ United Nations Scientific Committee on the Effects of Atomic Radiation. 2006 Report to the General Assembly. New York, NY: United Nations; 2008 United Nations Scientific Committee on the Effects of Atomic Radiation. Sources and effects of ionizing radiation. Medical radiation exposures, annex B. 2008 Report to the General Assembly with Scientific Annexes. New York, NY: United Nations; 2010Top Tips
Estimated annual per capita effective dose in the United States is approximately 5.6 mSv.
*Among diagnostic imaging procedures, computed tomography accounts for almost half of effective radiation doses to the general public, at 1.3 to 1.5 mSv annually. This is followed by nuclear medicine, with an estimated radiation dose of 0.6 to 0.8 mSv per year.
Whereas, previously, annual radiation dose from natural background radiation exceeded manmade sources, with the increased utilization of imaging for diagnostic and therapeutic applications, medical sources of radiation are overall approximately equal to background radiation doses.
Essentials 8
Case
A 62-year-old man undergoes thromboembolization of a cerebral arteriovenous malformation. He receives a cumulative radiation dose of 500 cGy from the procedure. Which of the following most accurately characterizes tissue reactions (formerly referred to as “deterministic effects”) resulting from the interventional procedure?
A. As depicted in curve A, the severity of tissue reactions increases with high radiation dose.
B. As depicted in curve A, the likelihood of tissue reactions is not modifiable.
C. As depicted in curve B, severe tissue reactions occur only at late stages or time points.
D. As depicted in curve B, the likelihood of tissue reactions is nonzero above a threshold dose.
E. As depicted in curve C, the likelihood of tissue reactions is expected to be constant at any dose.
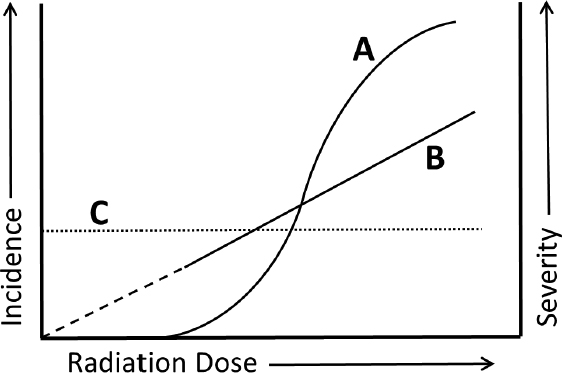
Answers and Explanations
A. Correct! “Tissue reactions,” formerly referred to as “non-stochastic” and then “deterministic” effects of radiation, are classically distinguished from radiation stochastic effects by the presence of a threshold dose (which may be quite low and variable depending on the tissue type), above which the severity of effects increases and below which there is no detectable effect.
Other choices and discussion
B. There is accumulating evidence that the likelihood (incidence) of tissue reactions, especially those reactions typically occurring late after the exposure, may be modified by substances such as antioxidants, anti-inflammatory drugs, and others.
C. Tissue reactions include damage occurring soon after the radiation exposure (“early”)—such as skin erythema, which may be seen within several hours—and those seen at remote time points after the exposure (“late”)—such as cataracts, sterility, and radiation-related circulatory disease, which may become evident years after the exposure.
D. Curve B depicts the “linear-no-threshold” model of stochastic radiation effects, not “tissue reactions.” This model holds that there is no threshold dose, but rather suggests that the occurrence (incidence) of effects increases linearly as a function of radiation dose. Stochastic effects include cancer and other radiation-induced heritable effects such as mental retardation.
E. Curve C depicts effects that are independent of radiation dose.
Suggested Readings
Hamada N, Fujimichi Y. Classification of radiation effects for dose limitation purposes: history, current situation and future prospects. J Radiat Res 2014;55:629–640 Saha GB. Physics and Radiobiology of Nuclear Medicine. 4th ed. New York: Springer; 2014 Stewart FA, Akleyev AV, Hauer-Jensen M, et al. ICRP Statement on Tissue Reactions/Early and Late Effects of Radiation in Normal Tissues and Organs—Threshold Doses for Tissue Reactions in a Radiation Protection Context. ICRP Publication 118. Ann ICRP 2012;41(1/2)Top Tips
The current terminology, “tissue reactions,” was formerly referred to as deterministic or nonstochastic effects.
There is a threshold dose for tissue reactions, and severity of tissue reactions increases with high radiation dose.
Essentials 9
Case
A nuclear reactor has been installed in a rural area of Alabama and is undergoing initial quality assurance testing before inspection by the Nuclear Regulatory Commission. The reactor is to be used predominately for the production of medical grade 131-iodine (131I) by fission of 235-uranium (235U). During testing, a reactor core overheats and partially melts, ultimately resulting in release of 131I into the air; no significnat quantities of other parent or daughter products are released. During the investigation of the accident, it is determined that, while there is no groundwater or soil contamination in the areas surrounding the reactor, there are high levels of 131I within air samples from the neighboring areas. Which of the following interventions would be most effective at minimizing total radiation dose to members of the public?
A. Re-cooling of the reactor core
B. Use of hand and body radiation detectors
C. Use of lead vests or lead thyroid shields
D. Distribution of ultraviolet-blocking eye protection
E. Distribution of potassium iodide pills
Answers and Explanations
E. Correct! In addition to its beta emissions with a maximum energy of ~606 keV, 131I emits high-energy photons (γ-rays) with a primary energy of 364 keV. Emitted beta particles typically travel several meters in air while high-energy photons. However, in this case, with aerosolized 131I in the air, the radioactive emissions are of concern both at the site of the accident and in the surrounding area, where 131I particles may result in both external and internal (due to inhalation) radiation exposures. Inhaled 131I may accumulate in the thyroid gland if unblocked. Therefore, the adminstration of a nonradioactive blocking agent such as potassium iodide or Lugol solution (aqueous solution of potassium iodide plus iodien) can be important in minimizing dose to the thyroid gland from beta radiation and gamma radiation dose from the source (thyroid) to other tissues (target).
Other choices and discussion
A. Re-cooling of the reactor core may be of little benefit as the core has already partially melted. In addition, because radiation release into the environment has already occured, re-cooling will be ineffective in minimizing the dose from the released 131I.
B. For radiation workers or those with occupational exposures to ionizing radiation, body and hand detectors are effective means of monitoring exposure. In the setting of a radiation accident, these detectors may be to assist in determining the total exposure to workers, but would not be helpful for minimizing dose.
C. 131I is a beta-emitter used most often in the treatment of hyperthyroidism and well-differentiated thyroid carcinoma. While lead vests may limit absorbed radiation from low-energy photons, the 131I half-value layer (HVL) of Pb is 3mm and the tenth-value layer is 11 mm. The thickness of typical lead aprons is 0.25 to 0.375 mm. Thus, these protective garments would be expected to block little of the primary photons emitted from 131I. In addition, it is important to note that high-Z materials such as lead produce penetrating Bremsstrahlung radiations (X-rays) when exposed to beta particles.
D. 131I does not produce ultraviolet radiations. Therefore, ultraviolet-blocking eyewear would be of no substantial benefit.
Suggested Readings
Sugarman SL, Goans RE, Garrett AS, et al. The Medical Aspects of Radiation Incidents. Knoxville, TN: Oak Ridge Institute for Science and Education (US); 2013. https://orise.orau.gov/files/reacts/medical-aspects-of-radiation-incidents.pdf Yamamoto LG. Risks and management of radiation exposure. Ped Emerg Care 2013;29:1016–1026Top Tips
131I is both a beta and gamma ray emitter.
131I accumulates in the thyroid gland if unblocked.
The 131I half-value layer of Pb is 3 mm and the tenth-value layer is 11 mm.
Essentials 10
Case
A single photon emission computed tomography camera is undergoing performance testing with a Jaszczak phantom. The nuclear medicine technologist fills the chamber surrounding the solid spheres and rods with a solution of dilute 99m-technetium pertechnetate (Na-TcO4). The filled phantom is used for:
A. Daily uniformity calculation
B. Daily energy peaking
C. Quarterly linearity testing
D. Quarterly system spatial resolution testing
E. Annual system performance testing
Answers and Explanations
E. Correct! The fillable Jasczak phantom is used for assessment of the imaging system overall and may be used for both single photon emission computed tomography and positron emission tomography systems, depending on the radiotracer used to fill the chamber. When filled, the phantom may be used to test system resolution, contrast, and uniformity. An overall system check should be performed at least annually, if not quarterly.
Other choices and discussion
A. Gamma camera uniformity should be tested with either a point source at a specified distance from the camera head (intrinsic uniformity) or with a sheet flood source (typically 57-Cobalt) placed directly on the collimator (extrinsic uniformity). Uniformity should be evaluated daily.
B. Energy peaking should be performed daily. The energy spectrum of the radioisotope to be imaged, most often 99m-technetium, should be analyzed to ensure that the photopeak of interest coincides with the preset energy window.
C. Linearity testing is done best with a four-quadrant bar phantom placed atop the collimator, with a point source at a specified distance from the camera head. Checks for linearity should be performed montly or quarterly.
D. Spatial resolution should be checked monthtly or quarterly, and much like uniformity, may be evaluated as a function of the intrinsic camera performance or the extrinsic camera performance. As with linearity, it is typically checked using a four-quadrant bar phantom, with the user identifying the number of quadrants in which the bar separations can be discerned.
Suggested Readings
Intersocietal Accreditation Commission. IAC Standards and Guidelines for Nuclear/PET Accreditation. Ellicott City, MD: Intersocietal Accreditation Commission; 2012 Zanzonico P. Routine quality control of clinical nuclear medicine instrumentation: a brief review. J Nucl Med 2008;49:1114–1131Top Tips
Uniformity, energy peaking → daily
Linearity testing → monthly or quarterly
Spatial resolution → monthly or quarterly
Overall system check → quarterly or annually
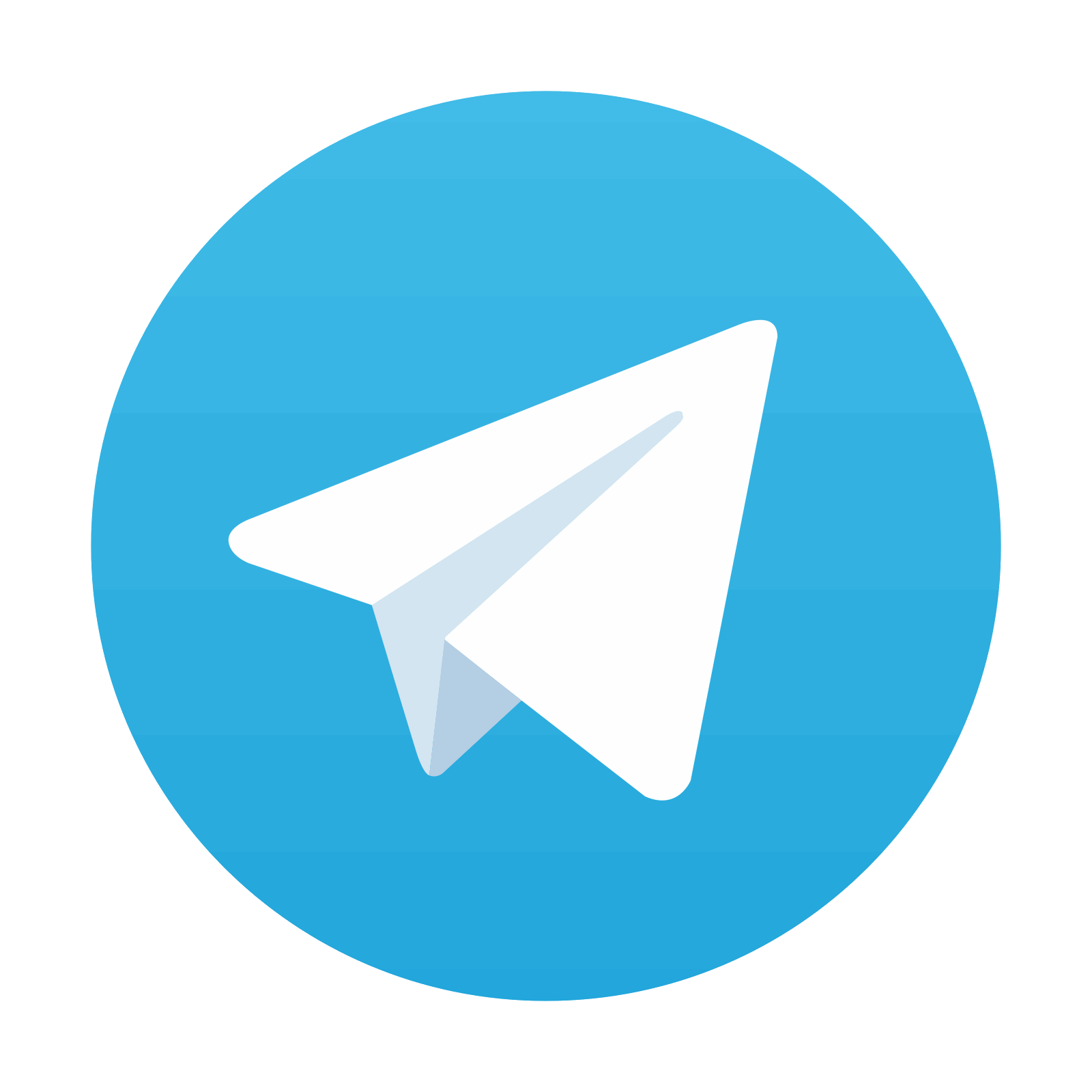
Stay updated, free articles. Join our Telegram channel

Full access? Get Clinical Tree
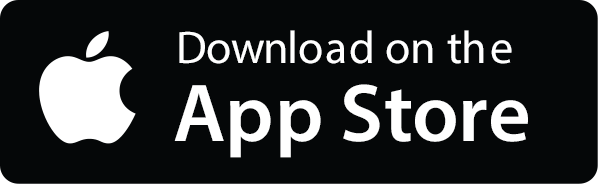
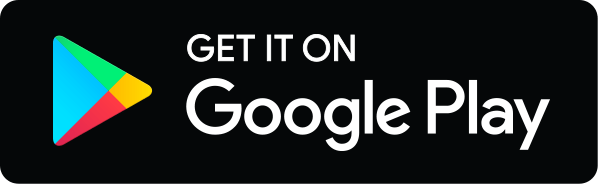
