Giovanna Ferraioli Department of Clinical, Surgical, Diagnostic and Pediatric Sciences, University of Pavia, Pavia, Italy Liver fibrosis is the common pathway of several chronic liver diseases. Assessment of the severity of liver fibrosis is crucial for determining the prognosis of patients and for evaluating the need for treatment. Elastography techniques are able to non‐invasively evaluate differences in the elastic properties of soft tissues by measuring the tissue behaviour when a mechanical stress is applied. Elasticity is an intrinsic biomechanical property of every tissue, including the liver. Due to fibrosis, the liver becomes stiffer than normal, and there is a close relationship between liver stiffness and fibrosis stage. Several studies and meta‐analysis have shown the important role played by shear wave elastography (SWE) techniques in the staging of liver fibrosis, and such techniques are increasingly replacing liver biopsy in clinical practice. Indeed, guidelines have accepted that, in several clinical scenarios, SWE can be used instead of liver biopsy for assessing the severity of liver fibrosis. Elastography techniques show the biomechanical properties of the tissue: under a stress, stiffer tissue shows less longitudinal displacement and higher speed of transverse displacement. B‐mode ultrasound, in contrast, shows the acoustic properties of tissue. Thus, B‐mode ultrasound and elastography give different and complementary information about the status of the liver. The term SWE includes all the techniques based on the assessment of the shear wave speed (SWS) [1]. The shear wave displacement is generated (i) by an external mechanical stress, as in vibration‐controlled transient elastography (VCTE) – which is performed with a dedicated system, the FibroScan® (Echosens, Paris, France) – or in magnetic resonance elastography (MRE); or (ii) by using the push‐pulse of a focused ultrasound beam, as in the acoustic radiation force impulse (ARFI)‐based techniques integrated into standard ultrasound systems. The shear waves travel at a speed between 1 and 10 m/s depending on the tissue stiffness: in stiffer tissue they propagate faster [2, 3]. SWE relies on the generation of shear waves determined by the displacement of tissue induced by external pressure (VCTE) or by the force of a focused ultrasound beam (ARFI‐based techniques). VCTE is performed with a dedicated device, the FibroScan. Vibrations of mild amplitude and low frequency (50 Hz) are transmitted by the tip of the transducer through the liver, inducing an elastic shear wave that propagates through the underlying tissue. The FibroScan is not a real‐time ultrasound device; however, A‐mode and time‐motion mode are used to locate the area of liver parenchyma to perform the measurement and to monitor the SWS propagation (Figure 9.1.1). Pulse‐echo ultrasound acquisitions are used to follow the propagation of the shear wave and to measure its velocity [4]. Software for the quantification of liver steatosis, the controlled attenuation parameter (CAP), has been developed and is available in the new generation of FibroScan devices. The CAP is based on the properties of ultrasound signals acquired by FibroScan using the hypothesis that fat affects ultrasound propagation. The CAP is evaluated using the same radio‐frequency (RF) data and region of interest (ROI) that are used for liver stiffness measurement (LSM). Figure 9.1.1 Transient elastography readings (FibroScan 502 Touch®, Echosens, Paris, France). On the monitor, all the measurements are displayed. The system automatically filters out invalid measurements and their number, if any, is shown on the top right. The median value of the measurements (in kPa) is reported together with the interquartile/median (IQR/M) ratio. The system also quantifies the attenuation of the ultrasound beam, in dB/m, using a proprietary algorithm (controlled attenuation parameter, CAP). The median CAP value, together with the IQR, is shown. Above the readings and from left to right: TM‐mode, A‐mode, and shear wave propagation speed reported in a distance‐time graph. In ARFI‐based techniques, a dynamic stress is applied with short‐duration acoustic forces (pushing pulses). It causes localised displacements deep within tissue; the shear waves that are generated are tracked and their speed, thus the elasticity of the tissue, is estimated. The ultrasound beams designed to create a measurable displacement with acoustic radiation force have longer pulses (50–1000 μs) able to generate micron‐level tissue displacements [2, 3]. The shear waves are transverse waves, with a motion perpendicular to the direction of the force that has generated them; that is, perpendicular to the direction of the ultrasound beam. In soft tissue, shear waves propagate at a speed 1000 times slower than the speed of sound and they attenuate very fast. The speed of the shear wave can be measured in a small ROI, as in point shear wave elastography (pSWE). B‐mode ultrasound is used to select the area of liver parenchyma to perform the measurement (Figures 9.1.2–9.1.6). With 2D‐SWE, quantitative images of the SWS (or stiffness) in a large ROI are obtained by placing the ARFI focus (push) at multiple sequential locations and, at each, detecting the shear wave arrival time at multiple lateral locations [2, 3]. An image, which is a colour‐coded map of the SWS, is built. A measurement box is then positioned inside the ROI to obtain the values of shear wave speed/stiffness (Figures 9.1.7–9.1.12). Figure 9.1.2 Point shear wave elastography (ElastPQ®, EPIQ Elite ultrasound system, Philips Healthcare, Eindhoven, Netherlands). The region of interest (the yellow rectangle) has a fixed size. The mean value of the push‐track sequences is given together with the standard deviation (SD). An SD ≤30% of the mean value indicates an acquisition of good quality. When the signal‐to‐noise ratio of an acquisition is very low, the mean value is not shown. SuperSonic Imagine (Hologic, USA) has a proprietary radiation force technique termed supersonic shear imaging. Ultrasound plane‐waves, which enable an ultrafast frame rate (typically faster than 1000 frames per second), are transmitted. The tissue displacement is generated at all positions along the acoustic axis almost simultaneously. This produces a shear wave in the shape of a cone, known as a Mach cone, which decays less rapidly with distance than that from a single push‐pulse [5]. ARFI‐based techniques are all implemented in ultrasound systems, either pSWE or 2D‐SWE. The manufacturers usually have registered names for their elastography products. SWS is displayed in metres/second (m/s). Assuming that the tissue has a very simple behaviour (i.e. it is linear, isotropic, and homogeneous) and is incompressible, the velocity of shear wave propagation can be converted to tissue stiffness through the Young’s modulus, E = 3 (ρ × vS2), where E is the Young’s modulus, ρ is the density of tissue, and vS is the SWS [2, 3]. The Young’s modulus is a measure of stiffness, and it indicates how difficult it is to deform a material by stretching or compression (E = uniaxial stress/uniaxial strain). The unit of measurement is the kilopascal (kPa); that is, the unit of pressure. It should be underlined that speed may also be converted to the shear modulus G, which measures the ability of a material to resist transverse deformations: shear stress/shear strain. The shear modulus has the same unit of measure as the Young’s modulus, kPa, but it is three times smaller: G = ρ × vS2
9.1
Shear Wave Elastography for Liver Disease: Part 1
Introduction to Liver Elastography and Different Elastographic Techniques
Physical Principles of Shear Wave Elastography
Vibration‐Controlled Transient Elastography
Acoustic Radiation Force Impulse–Based Shear Wave Elastography
Units of Measure
Stay updated, free articles. Join our Telegram channel

Full access? Get Clinical Tree
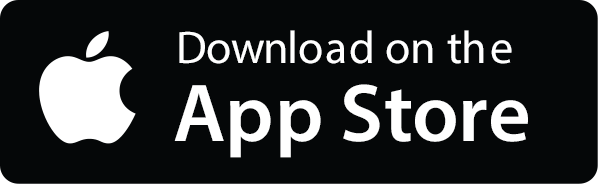
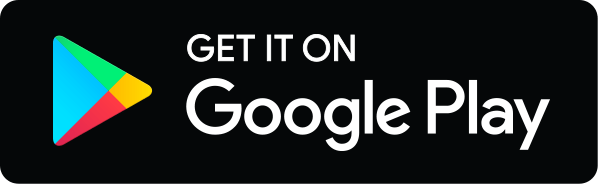