- •
The distinction between “simple” and “complex” congenital heart disease is not well defined. In this chapter, simple congenital heart disease describes uncomplicated anatomic defects or shunt lesions that are not associated with other cardiovascular anomalies.
- •
This chapter describes cardiovascular magnetic resonance (CMR) findings in the following congenital cardiac anomalies:
- •
Interatrial communications
- •
Ventricular septal defect
- •
Patent ductus arteriosus
- •
Partially anomalous pulmonary venous connection
- •
Coarctation of the aorta and bicommissural aortic valve
- •
- •
CMR is usually used in concert with other imaging modalities for assessment of cardiovascular anatomy and function, measurements of blood flow, tissue characterization, and for evaluation of myocardial perfusion and viability.
- •
CMR is particularly helpful in adolescent and adult patients with congenital heart disease because it overcomes many of the limitations associated with echocardiography (e.g., restricted acoustic windows), cardiac catheterization (e.g., invasive, expensive, radiation exposure), computed tomography (e.g., radiation exposure, predominantly static anatomic information), and nuclear scintigraphy (e.g., low spatial resolution, lack of anatomic information, radiation exposure).
INTERATRIAL COMMUNCATIONS
Types of Interatrial Communications
Types of interatrial communications are shown in Figure 14-1 . Patent foramen ovale (PFO) is the most common type of interatrial communication. Anatomically, it is located between normally formed septum primum and septum secundum. The second most common type of interatrial communication is secundum atrial septal defect, followed by primum atrial septal defect.
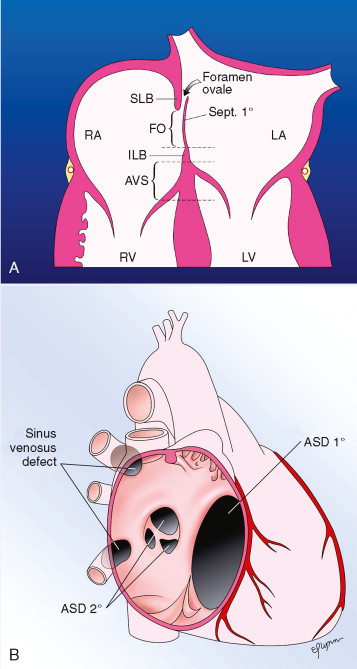
Secundum atrial septal defect ( Figures 14-2 and 14-3 ) is a defect within the fossa ovalis, usually caused by a single or multiple defects within septum primum. Septum secundum is usually well formed. Most secundum atrial septal defects (ASDs) are not confluent with the vena cavae, right pulmonary veins, coronary sinus, or the atrioventricular (AV) valves. Primum atrial septal defect ( Figures 14-4 to 14-6 ) is an endocardial cushion (AV canal) defect with an interatrial communication located between the anterior-inferior margin of the fossa ovalis and the AV valves. It is considered a form of partial AV canal defect with two separate AV valve annuli and no ventricular septal defect of the AV canal type. Sinus venosus defect is a communication between one or more of the right pulmonary veins and the cardiac end of the superior vena cava (SVC) and/or the posterior wall of the right atrium. Sinus venosus defect ( Figures 14-7 to 14-9 ) comprises approximately 4% to 11% of interatrial communications. Although anatomically not a true atrial septal defect, sinus venosus defect results in an interatrial communication and is hemodynamically similar to an atrial septal defect.
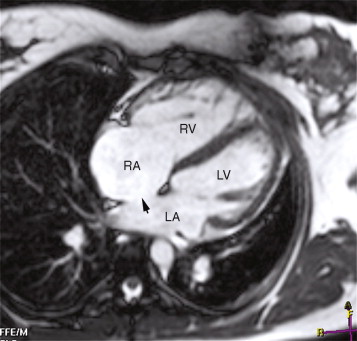
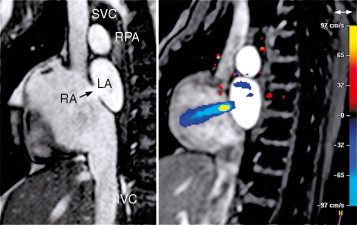
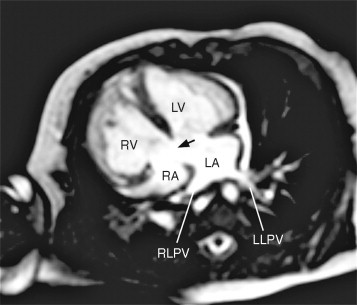
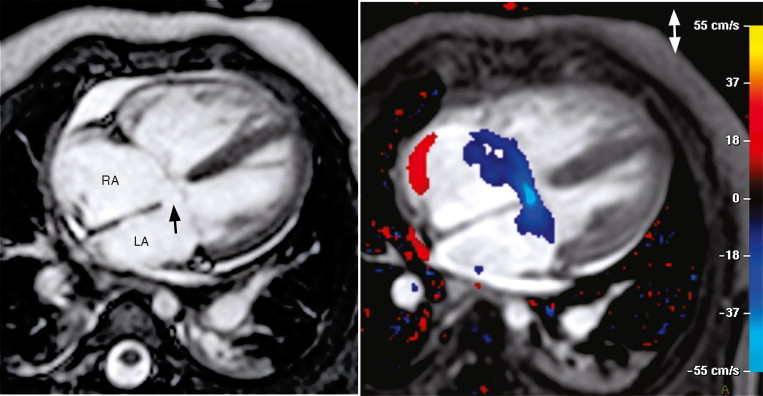
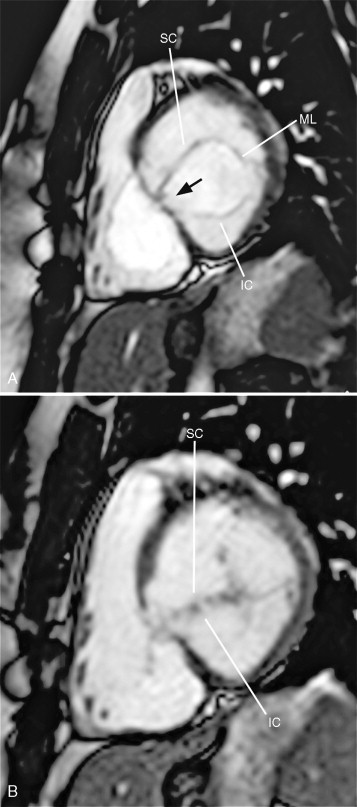
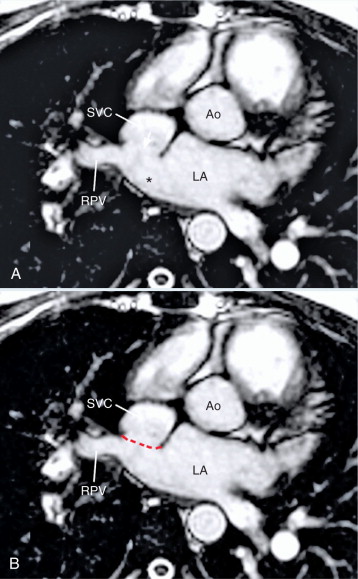
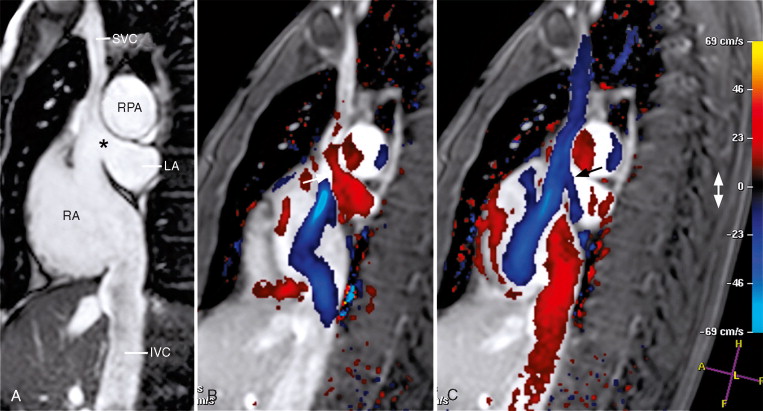
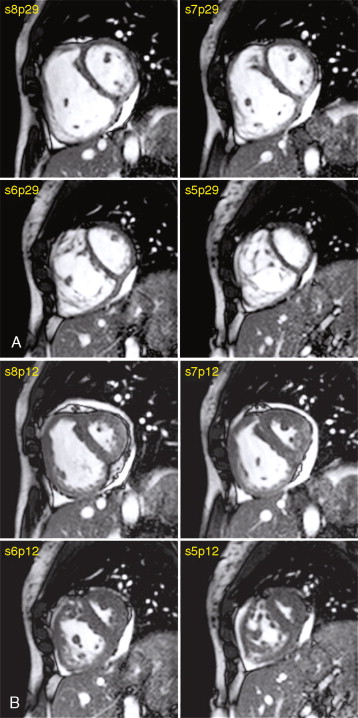
Measurement of Pulmonary-to-Systemic Flow Ratio in Patients with Interatrial Communications
In the absence of associated cardiovascular anomalies and with normal pulmonary vascular resistance, interatrial communications result in a left-to-right shunt proximal to the AV valves. The volume of the left-to-right shunt depends on the size of the defect and the relative compliance of the right and left ventricles. Most patients are asymptomatic during childhood and adolescence. Some patients with unrepaired interatrial communications will develop complications during adult life, including pulmonary vascular disease, atrial arrhythmias, heart failure, and paradoxical emboli. Indications for closure of interatrial communications in children include symptoms (e.g., exercise intolerance, dyspnea, slow weight gain), left-to-right shunt greater than 1.5 to 2.0 associated with right ventricular volume overload, and paradoxical emboli. Although a patent foramen ovale or a small secundum atrial septal defect may decrease in size or even close spontaneously during infancy and early childhood, larger secundum atrial septal defects can increase in size during adulthood. Primum atrial septal defects and sinus venosus defects almost never decrease in size over time. CMR allows evaluation of the location and size of the interatrial communication as well as quantitative assessment of the hemodynamic burden from the defect(s), such as pulmonary-to-systemic flow ratio, right ventricular size and function, etc. ( Figures 14-10 and 14-11 ).
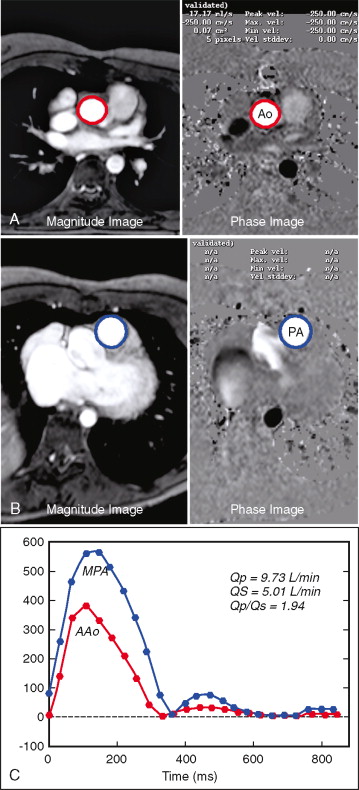
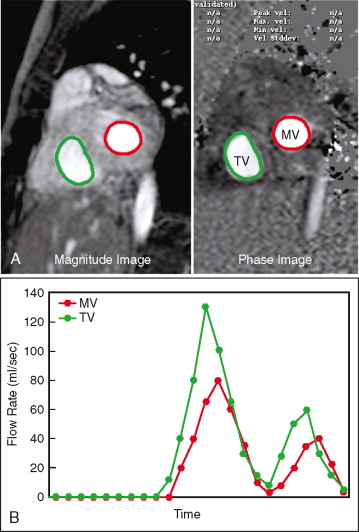
VENTRICULAR SEPTAL DEFECTS
Different types of ventricular septal defects (VSDs) are shown in Figure 14-12 .
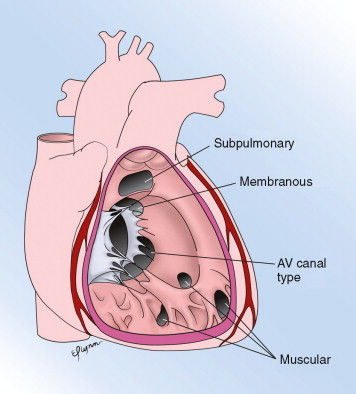
Conoventricular septal defect ( Figure 14-13 ) results from malalignment between the conal (outlet) septum superiorly and the muscular ventricular septum inferiorly. A conoventricular septal defect may be membranous if its posterior-inferior border is confluent with the tricuspid valve or may be muscular if the inferior limb of septal band forms its inferior-posterior border.
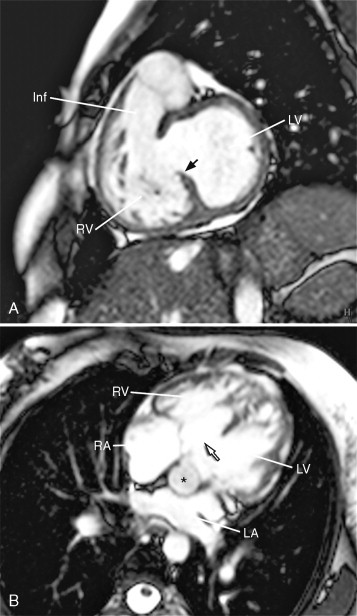
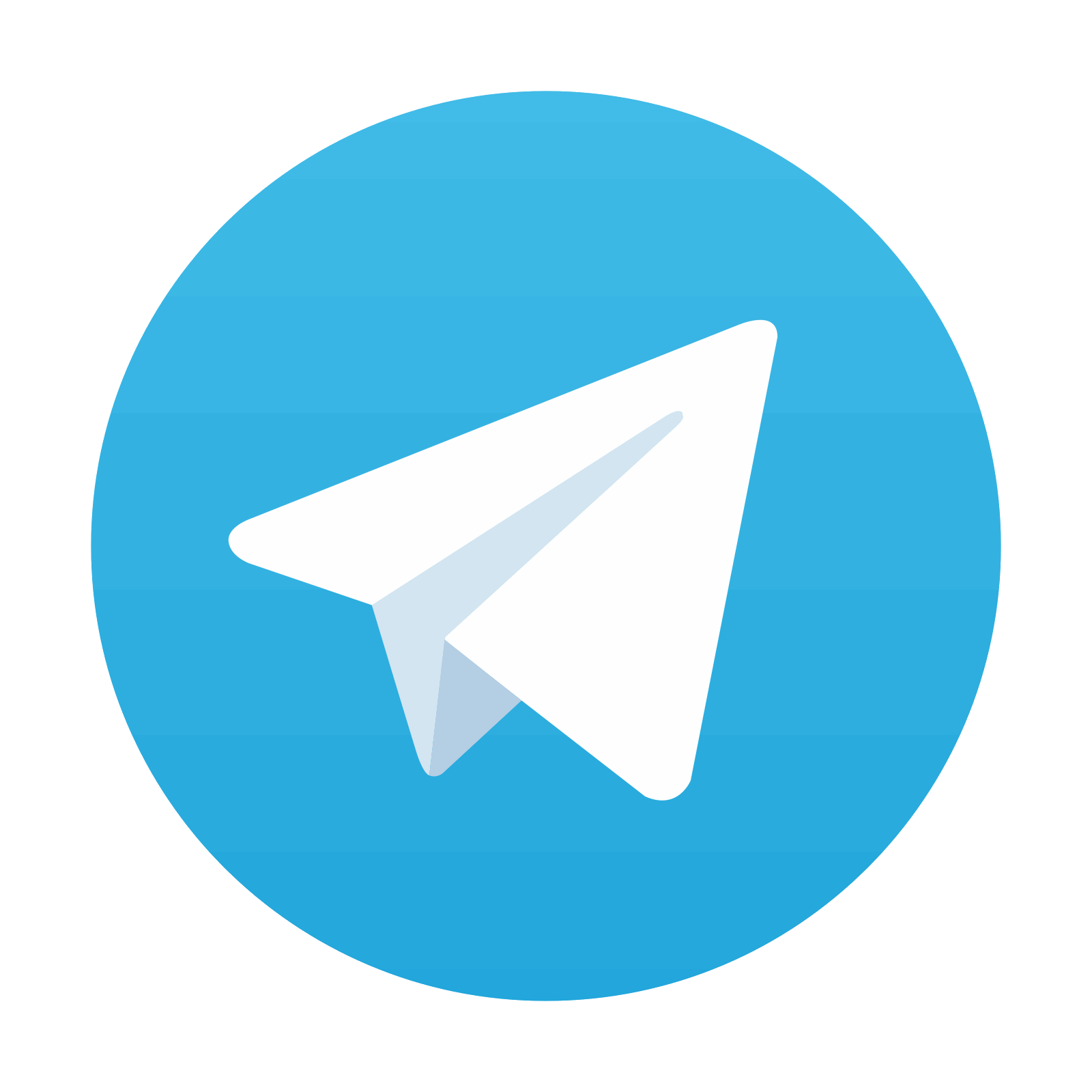
Stay updated, free articles. Join our Telegram channel

Full access? Get Clinical Tree
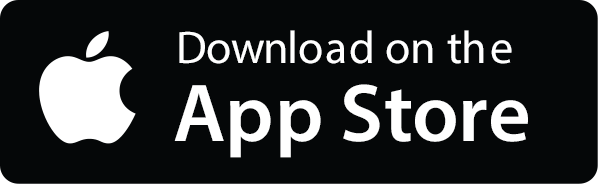
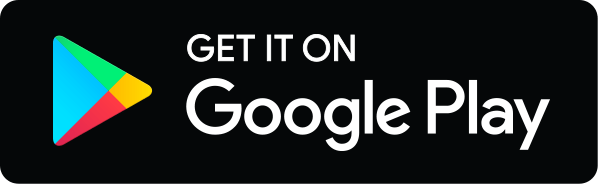
