During the last three decades, cardiovascular magnetic resonance (CMR) has developed into an important diagnostic clinical tool in cardiology. Not only the anatomy of the heart but also its function, metabolism, and perfusion, as well as the coronary arteries, can be evaluated with CMR. There are advantages of CMR over other diagnostic imaging methods: first, CMR does not use ionizing radiation; second, the radiofrequency (RF) radiation penetrates bony and air-filled structures without attenuation; third, CMR gives additional diagnostic information about tissue characteristics; and, finally, CMR provides three-dimensional (3D) images or images of arbitrarily oriented slices.
However, when performing CMR, particular precautions must be taken. Because CMR operates with high static and gradient magnetic fields, special safety regulations must be taken into account and certain contraindications must be considered. This chapter reviews the safety, electrocardiographic (ECG) setup, patient monitoring, and contraindications to CMR; in particular, the issue of devices (pacemakers, implantable cardioverter defibrillators [ICDs]) as well as coronary stents and prosthetic heart valves, is addressed.
Safety of Cardiovascular Magnetic Resonance
General Issues
CMR generally takes longer than other diagnostic modalities (although the time is significantly shortened with real-time imaging ), and the confined space in which the patient is placed is rather narrow, which some patients find uncomfortable. During CMR, communication with the patient may be difficult because of interfering noise from the gradient coils. On the other hand, CMR is entirely noninvasive. Overall, the safety issues during CMR that may pose potential safety concerns can be summarized as follows :
- 1.
Biologic effects of the static magnetic field
- 2.
Ferromagnetic attractive effects of the static magnetic field on certain devices
- 3.
Potential effects on the relatively slow time-varying magnetic field gradients
- 4.
Effects of the rapidly varying RF magnetic fields, including RF power deposition concerns
- 5.
Auditory considerations of noise from the gradients
- 6.
Safety considerations concerning superconducting magnet systems
- 7.
Psychological effects
- 8.
Possible effects of the intravenous use of magnetic resonance (MR) contrast agents
- 9.
Patient safety during stress conditions
Biologic Effects
Concerning the biologic effects of a static magnetic field, many structures in animals and humans are affected by magnetic fields. Many potential biologic effects and different magnitudes of magnetic fields have been examined, including the effect of the field on cardiac contractility and function. Gulch and colleagues concluded that static magnetic fields used in CMR do not constitute any hazard in terms of cardiac contractility. These magnetic fields do not increase ventricular vulnerability, as assessed by the repetitive response threshold and the ventricular fibrillation threshold. In one of the investigations, however, cardiac cycle length was shown to be altered. Two studies reported that lymphocytes from blood samples obtained in humans exposed to a 1.5 tesla (T) field demonstrated DNA double-strand breaks and changes consistent with mild inflammation. However, these studies have significant limitations and four recent reports have found no such DNA damage. Another area of concern is CMR during pregnancy, for example, teratogenicity (cellular effects, for example on differentiation, have been demonstrated in animals), damage to the fetal inner ear due to noise exposure and tissue heating effects, although none have been documented to cause harm. A recent study of CMR in pregnancy (including first trimester) found CMR to be safe. Furthermore, 7 T scanners are becoming more common (although currently very rarely used for CMR), and have been associated with transient dizziness upon movement of the subject into the scanner (postulated to be due to induced currents affecting vestibular hair cells in the inner ear), as well as a metallic taste in the mouth in up to 11% of patients. Numerous biologic effects on other systems have been investigated extensively, and it may be concluded that no deleterious biologic effects from static magnetic fields used in CMR have yet been established.
Ferromagnetism
The physical effect of the static magnetic field consists of a potential health hazard from the attractive effect on ferromagnetic objects. Ferromagnetic objects can be defined as those in which a strong intrinsic magnetic field can be induced when they are exposed to an external magnetic field. The existence of different kinds of scanners with different shielding makes the discussion about this topic even more crucial. When dealing with a static magnetic field, two types of physical concerns exist.
First, there are concerns about forces exerted on ferromagnetic objects within, on, or distant from the patient. These forces result in rotational (torque) or translational (attractive) motion of the object. Within the human body, a ferromagnetic metallic structure may be sufficiently attracted, or have a sufficient amount of torque exerted, to create a hazardous situation. These factors should be carefully considered before subjecting a patient with a ferromagnetic implant or material to CMR, particularly if the device is located in a potentially dangerous area of the body, where movement or dislodgment of the device could injure the patient. Another potentially injurious effect is known as the projectile, or missile, effect. This refers to the fact that ferromagnetic objects have the potential to gain sufficient speed during attraction to the magnet that the accumulated kinetic energy could be injurious or even lethal if the object were to strike a patient. Numerous studies have been performed to assess the ferromagnetic qualities of various metallic implants and materials. The results indicate that patients with certain metallic implants or prostheses that are nonferromagnetic or are minimally deflected by static magnetic fields can safely undergo CMR. The literature on this topic has been extensively reviewed and compiled. However, there are common misconceptions about what types of objects are ferromagnetic. The most important misconception is that stainless steel is ferromagnetic, when it is not. Patients with stainless steel implants can therefore be imaged safely, except for a small number of well-described exceptions, as discussed later. The implant will interfere locally with the images; for example, signal loss occurs around metallic prosthetic valves ( Fig. 10.1 ) and sternal wires ( Fig. 10.2 ) after bypass surgery, but this does not make the imaging hazardous. Nonstainless steel, which may be ferromagnetic, is not used for human implants, but is commonly used for oxygen cylinders, for example. Finally, batteries are typically attracted to the magnet, and this is one of the problems of imaging pacemakers.
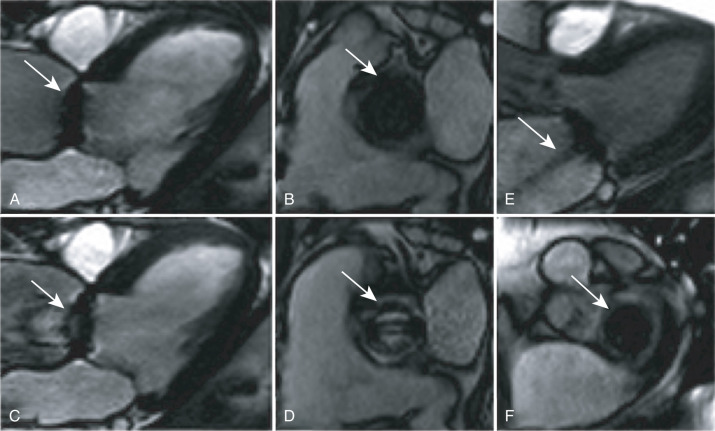
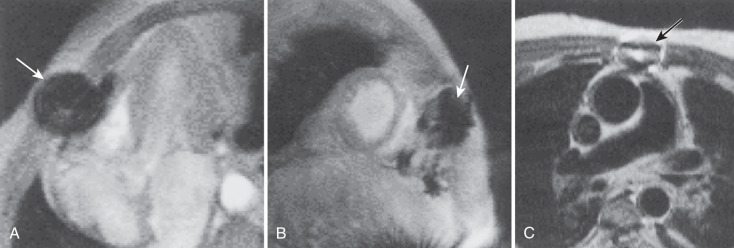
The second type of physical concern deals with magnetically sensitive equipment, the functioning of which may be adversely affected by the magnetic field. The most common of these is the cardiac pacemaker. Most pacemakers include a reed relay switch whereby the sensing mechanism can be bypassed and pacing in the asynchronous mode can occur. This switch is activated when a magnet of sufficient strength is held over the pacemaker. In addition, the function of cardiac pacemakers may be influenced by field strengths as low as 17 gauss. In practice, reed switch closure can be expected in all pacemakers placed in the bore of the scanner. Pacemaker and ICD function is considered again later in this chapter.
Effect of Rapidly Switched Magnetic Fields
CMR exposes the patient to rapid variations of magnetic fields by the transient application of magnetic gradients during imaging. The effect of rapidly switched magnetic fields may be the induction of currents within the body or any other electrical conductor, according to Faraday’s law. The current is dependent on the time rate of change of the magnetic field (d B /d t ), the cross-sectional area of the conducting tissue loop, and the conductivity of the tissue. Biologic effects of induced currents can be caused either by power deposition by the induced currents (thermal effects) or by direct effects of the current (nonthermal effects). Thermal effects as a result of switching gradients are not believed to be clinically significant. Possible nonthermal effects include stimulation of nerve or muscle cells. The threshold currents for nerve stimulation and ventricular fibrillation are known to be much higher than the estimated current densities induced under clinical CMR conditions. The echo planar imaging method, however, involves more rapidly changing magnetic field gradients, and peripheral muscle stimulation in humans has been reported. Such considerations have become more important as new technology has allowed the introduction of commercially available ultrafast gradient switching systems, and guidelines for maximum magnetic field variation are under development.
Radiofrequency Time-Varying Field
The transmitted RF time-varying field induces electrical currents within the tissue of the patient. The majority of this power is transformed into heat within the patient’s tissue as a result of ohmic heating. The time-varying magnetic gradients have the potential to cause either thermal or nonthermal biologic effects. The distinction between these two is a matter of frequency, waveform shape, and magnitude. The discussion about nonthermal effects from RF magnetic fields is controversial because of questions about the relationship between chronic exposure to electromagnetic fields over many years and the causation of cancer or developmental abnormalities. The most recent evidence suggests that proximity to power lines is not injurious. Clearly, acute exposure of a patient to short-term RF fields for a diagnostic CMR examination is different from chronic exposure. The induced currents from RF magnetic fields are unable to cause nerve excitations. One of the difficulties faced by medicine is proving that a procedure is not injurious because of anecdotal case reports of adverse events and publication bias toward nonneutral reports. This issue is also faced by such a well-established technology as ultrasound, where safety concerns have been raised over acoustic exposure.
In contrast to the insignificant thermal effects caused by switched gradients, however, thermal effects as a result of RF pulses are of significant concern. The main biologic effects induced by RF fields are therefore related to the thermogenic qualities of the RF field. A general point of discussion is the appropriate safety regulations for levels of magnetic field strength in CMR imaging. Application of the fundamental law of electrostimulation is well established, both on theoretical and experimental grounds. Application of this law, in combination with Maxwell’s law, yields an equation called the fundamental law of magnetostimulation, which has the hyperbolic form of a strength-duration curve and allows an estimation of the lowest possible value of the magnetic flux density capable of stimulating nerves and muscles. Calculations have shown that the threshold for heart excitation is more than 200 times higher than for nerve and muscle stimulation, depending on pulse duration. However, in clinical practice, some precautions are necessary. First and most importantly, the specific absorption rate of the imaging sequence being operated is monitored by the scanner software and must be kept below limits set by such bodies as the US Food and Drug Administration (FDA). Second, circumstances that could enhance the possibility of heating injury should be avoided. This includes ensuring the prevention of loops that could act as aerials within the scanner and enhance the heating effect locally. Therefore, patients should not be allowed to cross their legs (loop via the pelvis) or clasp their hands (loop via the shoulder and upper chest). The simple use of pillows prevents such problems. Other possible loops include the ECG leads, which should always be run out of the scanner parallel to the main field, and not looped across the chest. Finally, pacemaker and ICD leads make excellent aerials. The pacemaker lead can heat significantly during CMR and become a potential hazard (discussed later). Another consideration in patients after cardiac surgery is the effect of retained epicardial pacemaker leads. These leads can be left in place after surgery, and they may therefore act as an antenna during CMR. Studies have suggested that such short retained epicardial wires do not pose a significant problem.
Finally, the use of ECG electrodes, which are essential for cardiac gating, must be considered. Metallic ECG electrodes may cause burns during CMR, but this risk can be reduced with the use of carbon fiber electrodes, and these have now become standard.
Auditory Considerations
During CMR, the gradient coils and adjacent conductors produce a repetitive sound because they act essentially as loudspeakers, with current being driven through them while they are in a magnetic field. Auditory considerations should therefore be taken into account when imaging a patient. The amplitude of this noise depends on factors such as the physical configuration of the magnet, the pulse sequence type, timing specifications of the pulse sequence, and the amount of current passing through these coils. In general, the amplitude of the generated noise from the clinical CMR scanners remains between 65 and 95 dB. However, there have been reported instances of temporary hearing impairment as a result of CMR. Magnet-safe headphones or wax earplugs are readily available and have been shown to prevent hearing loss, and these are in common use. Systems combining sound attenuation with the facility to play music of the patient’s choice are also available. Research on the reduction of noise in MR scanners is ongoing, and the use of antinoise is one area of interest.
Superconducting System Issues
Most superconducting CMR scanner systems use liquid helium. The helium maintains the magnet coils in their superconducting state. Helium achieves the gaseous state at approximately −269°C (4 K). If for any reason the temperature within the cryostat rises, or in a system quench, the helium will enter the gaseous state. This means a marked increase in volume and thereby pressure within the cryostat. A pressure-sensitive valve is designed to give way to the gaseous helium, which is always vented outside the CMR scanner room. However, it is possible that some helium gas is released into the imaging room should the system not work perfectly. Asphyxia and frostbite are potential hazards if a patient is exposed to helium vapor for a prolonged time, although there are no reports of such an occurrence in the medical community. For older scanners that still use a buffer of liquid nitrogen within the system (boils at 77 K), an oxygen monitor is recommended in the scanner room. Cryogenic dewars should be stored away from the scanner and in well-ventilated areas.
Psychological Effects
Claustrophobia or other psychological problems may be encountered in up to 10% of patients undergoing CMR, although on average, the incidence is closer to 2% to 4%, and this can be reduced further to a small number of intractably anxious patients by the use of explanation, reassurance, and where necessary, light sedation with, for example, 2 to 5 mg intravenous (IV) diazepam. The development of shorter magnets and open designs are promising, although 39% of patients experienced some level of claustrophobia in a short-bore scanner and 26% in an open-bore scanner in a direct comparison.
Such problems are related to a variety of factors, including the restrictive dimensions of the scanner, the duration of the examination, the noise, and the ambient conditions within the magnet bore. Fortunately, adverse psychological effects with CMR are usually transient. In a study reported by Weinreb and colleagues, based on the experience of 450 patients undergoing CMR and computed tomography (CT) examinations, it was clearly shown that patients often prefer the CMR study, although CMR took longer. Furthermore, the patient is placed into a confined space and there are difficulties in communicating with the patient during CMR scanning because of the noise from the gradient coils and the necessity of eliminating all extraneous RF sources from the examination room. To a certain extent, this can be avoided when the patient assumes a prone position in the scanner, facilitating communication with the outside surroundings. Simple maneuvers, such as using mirrors, also help in allowing the patient a clear view of the scanning room. Allowing the anxious patient to visit the scanner before the appointment gives the patient an opportunity to become familiar with the facility and staff.
Safety Considerations Associated With CMR Contrast Agents
The safety profile of the contrast agents containing gadolinium currently on the market is extremely good. Their safety profile is well documented. The median lethal dose of gadopentetate dimeglumine (Gd-DTPA) is roughly 10 mmol/kg, which is 100 times the diagnostic dose and exemplifies the wide safety margin that these contrast agents enjoy. Patient tolerance is also high, and the prevalence of adverse reactions is approximately 2%. Among the reactions related to the IV administration of gadolinium contrast agents are headache, nausea, vomiting, local burning or cool sensation, and hives. There have been reported incidents of anaphylactoid reactions associated with IV injection, although the frequency of this appears to be approximately 1 per 100,000 doses. The safety margins with these agents appear to be considerably better than with x-ray contrast agents, although issues of nephrogenic systemic fibrosis are a concern in patients with severely impaired renal function, and there are differences in safety between the commercially available gadolinium contrast agents favoring the tighter-bound chelates (see Chapter 3 ). Evidence of cerebral deposition (especially globus pallidus and dentate nucleus) of gadolinium-based contrast agents has emerged from animal and human studies, although the mechanism(s) and clinical implications are currently unknown. The risk of cerebral deposition appears related to the number of administrations of gadolinium-based contrast. The use of gadolinium in pregnancy depends on the risk-benefit ratio, as in general contrast agents are best avoided. Gadolinium can be used and safety appears good, but with a possible small increase in adverse outcomes, the interpretation of which is limited by small numbers. For CMR, these agents are used to increase contrast between blood and soft tissue for cine imaging for functional studies or angiography, to enhance cardiac tumors and cysts, to assess myocardial perfusion, and to examine for myocardial infiltration. In summary, FDA-approved gadolinium complexes can be safely used in patients with cardiac disorders.
Multiple new CMR contrast agents are being developed and investigated. These are mainly gadolinium complexes, sometimes with novel binding molecules for special actions, but in addition, iron-based compounds are being developed. Some of these agents (e.g., gadofosveset) bind to albumin and are retained in the vascular system and do not leak into the extravascular space. The adverse-effect profile of gadofosveset appears similar to other gadolinium-based agents, although a prolongation of the corrected QT interval (without any dysrhythmias) has been reported. These agents may have clinical utility for angiography, possibly in the coronaries, and for functional imaging. Hyperpolarized CMR—executed with tracers such as 1- 13 C pyruvate—has only recently been introduced to humans and safety data are limited. Molecular imaging employs “probes,” which bind to molecular targets either actively or passively by accumulation, and trace various anatomical and functional processes, such as thrombus formation, angiogenesis, atherosclerotic plaque, and fibrosis development. Most of these techniques have only been tested in animals, and safety data in humans are scarce.
Patient Safety During Stress Conditions
A concern with stress CMR scans has been the ability to handle emergency situations. Patient monitoring during stress conditions is a critical issue because myocardial ischemia can be provoked in patients with coronary artery disease. Commercial equipment is available for noninvasive monitoring of blood pressure, heart rate, oxygen saturation, and other vital parameters in CMR scanners. The most crucial difference compared with conventional exercise testing outside a magnetic field is the lack of a diagnostic ECG, in particular, at high levels of stress, precluding the proper assessment of stress-induced ST-segment changes. This holds for both conventional exercise using a specially adapted bicycle ergometer and pharmacologically induced stress. Under these circumstances, only heart rate can be monitored reliably. When performing pharmacologic stress CMR (e.g., with dipyridamole, adenosine, regadenoson, or dobutamine), an experienced physician should be present during the examination, and appropriate treatments for complications should be in direct proximity. Dipyridamole (half-life 30 minutes), adenosine (half-life 10 seconds) and regadenoson (half-life 3 minutes) are vasodilators. These agents have similar side effects, such as hypotension, arrhythmias, and bradycardia. During adenosine infusion, atrioventricular (AV) heart block may develop in a small percentage of patients (0.7%–2.8%), although this is usually asymptomatic and self-limited. When patients are symptomatic, the short physical half-life of adenosine means that heart rhythm and symptoms can be restored very quickly by halting the infusion. As a suitable antagonist to dipyridamole, adenosine, and regadenoson, aminophylline may be given slowly at an initial dose of 50 mg IV up to a maximum dose of 250 mg if necessary. In the case of persisting advanced heart block, 0.5 mg atropine IV should be administered up to a total dose of 3 mg. Dipyridamole and adenosine should not be administered to patients with asthma, whereas regadenoson is safe in patients with asthma and chronic obstructive pulmonary disease, because of its more selective action on A 2A receptors. Adenosine, and dipyridamole indirectly (by inhibiting the degradation of adenosine), acts on A 1 , A 2A , A 2B , and A 3 receptors. A 2A receptors mediate coronary and peripheral vasodilation, whereas A 1 , A 2A , A 2B , and A 3 receptors cause bronchoconstriction and AV block. A further advantage of regadenoson is the fact that it can be administered as a bolus, therefore not requiring a CMR-compatible infusion pump. Dobutamine (half-life 2 minutes) is a beta-agonist leading to an increase in cardiac inotropy (contractility) and chronotropy (heart rate). Common side effects are palpitations, and less commonly, arrhythmias, such as supraventricular tachycardia and (nonsustained) ventricular tachycardia. Dobutamine can be safely administered to patients with asthma. The actions of dobutamine can be counteracted by IV administration of a short-acting beta-blocking agent, such as esmolol. In the case of cardiac arrest or ventricular fibrillation, the recommendations should be followed according to published guidelines, such as those proposed by the European Resuscitation Council. In every CMR facility, an alarm system and a written flow chart should be visually available, with the necessary instructions in case of emergency. It is necessary to be able to remove the patient from the examination room quickly (preferably within 20 seconds) to an area where emergency treatment can be performed safely, away from the hazards of the magnetic field. A nonferromagnetic stretcher stored in the scanner room or a detachable scanner table is ideal. A cardiac arrest trolley must be maintained in close proximity to the scanner room, and all staff should undergo regular training in cardiopulmonary resuscitation techniques. Regular checks should be made of both the resuscitation equipment and the alarm system.
Patient Monitoring and Electrocardiographic Setup
Patient monitoring during CMR poses problems that will not be familiar to users of other technologies, such as echocardiography. Ferrous metal, which is present in most monitoring equipment, can distort the magnetic field, and such an item has the potential to become a projectile. In addition, monitoring wires that are attached to the patient, leaving the scanner, and passing to another room may act as an antenna for stray RF signals. Electrical equipment in the scanner room also can act as a source of RF noise. All of these disturbances may result in image degradation. Therefore, specific solutions to these problems have been designed.
Commercially available CMR-compatible monitoring equipment, including that used to measure ECG, blood pressure, and chest wall movements, as well as for general anesthesia, has been tested in several studies. Satisfactory monitoring can be obtained and images obtained during its use can be evaluated adequately. For some monitoring, simple solutions work, such as that reported by Roth and associates, who measured arterial blood pressure outside the CMR scanner by lengthening the rubber tubing connected to a blood pressure cuff. The newest monitoring equipment eliminates the need for wires and tubes to leave the scanning room by using a microwave transmitter communicating with a slave display unit in the operating room.
The CMR procedure depends on a high-quality ECG signal for routine imaging, and each manufacturer has developed its own solution to the problems posed. Fiberoptic transmission of ECG signals for gating is now common, and this significantly reduces RF pulse artifacts in the ECG. Felblinger and coworkers showed that this type of system could yield signals almost free from interference, during both conventional and high gradient activity sequences, such as during echo planar imaging. From this signal, the authors also developed a method for respiration monitoring during MR sequences. Third-party ECG solutions are also now being incorporated into the latest generation of scanners, and these come with specific ECG recommendations for lead placement. Carbon fiber electrodes are required to eliminate the risk of burning that has been reported with standard metallic ECG electrodes. Typical lead placement is the result of compromise. A better signal results from widely spaced electrodes, but this results in more artifact from the gradients. In general, therefore, the leads are kept relatively close together, and on the left side, which reduces the magnetohydrodynamic effect (the effect of systolic aortic flow causing surface potentials on the ECG that distort the ST segment; Fig. 10.3 ). A typical lead placement that is commonly adopted is shown in Fig. 10.4 . Some centers have found ECG gating using electrodes on the back to be successful, but this is not widely used. The ECG leads should not be allowed to form loops, which could present a burning hazard, and they should be braided together and brought out of the magnet while aligned parallel to the bore to reduce electrical interference. Keeping the electrical cables short is helpful, and fiberoptic conversion modules are therefore often very close to the patient’s chest. Switching between the ECG traces sometimes allows flexibility to reduce gating errors from tall T-waves or electrical interference. One thing is certain, however, and that is that time spent ensuring that the ECG is stable and working correctly at the start of the scan is time very well spent.
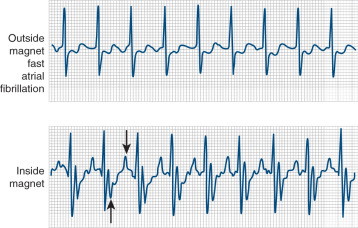
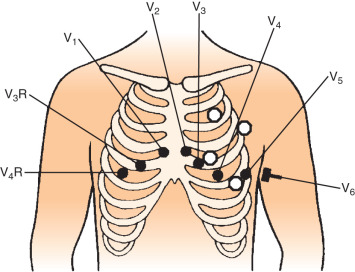
An alternative technique to routine surface ECG recording has been described by Fischer and colleagues using vectorcardiography, and has been widely implemented. The system examines the 3D orientation of the ECG signal and uses the calculated vector of the QRS complex as a filter mechanism to ignore electrical signals, which are of similar timing and similar magnitude in the cardiac cycle, but a different vector. The system as reported identified the QRS complex correctly in 100% of cases, with 0.2% false-positive findings. In a subsequent study in normal individuals and patients with supraventricular extrasystoles, the same authors showed that vector cardiography-based triggering provided nearly 100% triggering performance during CMR examinations. This system represents a significant improvement for CMR in stabilizing this important gating signal.
Should interpretation prove impossible for technical reasons, a standard vascular Doppler can be used to monitor heart rate during CMR. The Doppler and telemetric ECG do not contain enough ferromagnetic material to cause visible image degradation. Jorgensen and associates evaluated whether patients could be monitored during CMR with 1.5 T machines in a manner that complies with monitoring standards.
The high magnetic field can interfere with normal functioning of equipment, not only monitoring equipment but also smaller items such as infusion pumps used for stress testing. In general, the influence of the CMR scanner on nearby equipment depends on several factors, such as the strength of the CMR magnetic field, the proximity of the equipment to the scanner, the amount of ferromagnetic material in the equipment, and the design of the electrical circuitry.
Finally, simple devices, such as closed-circuit television and a two-way intercommunication system, also aid in monitoring by allowing a constant view of the patient and easy communication if the patient is in discomfort. However, the latter may be impaired during imaging as a result of the noise of the gradients. Because sequences are now commonly being reduced in duration to a breath-hold, however, this limitation is becoming much less important.
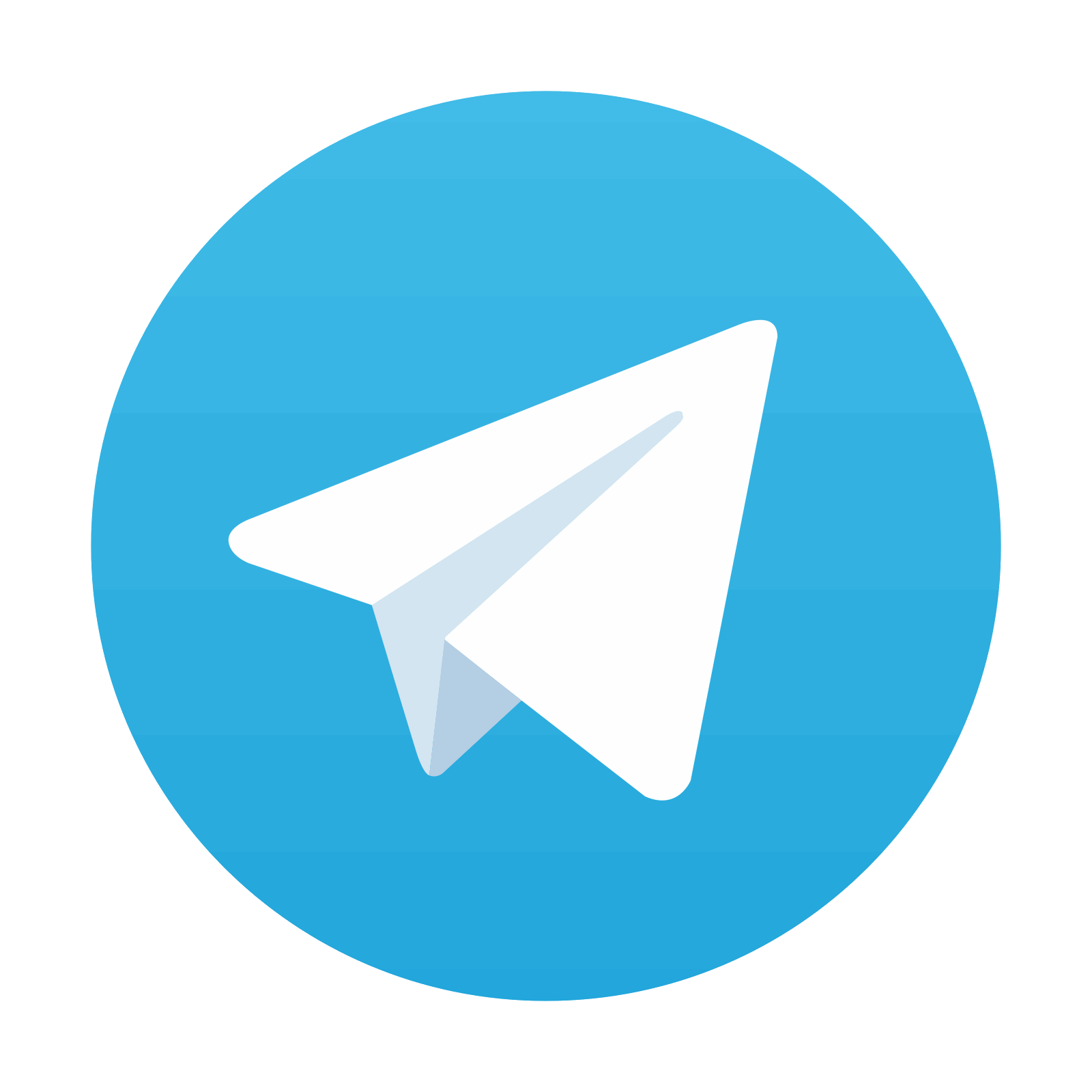
Stay updated, free articles. Join our Telegram channel

Full access? Get Clinical Tree
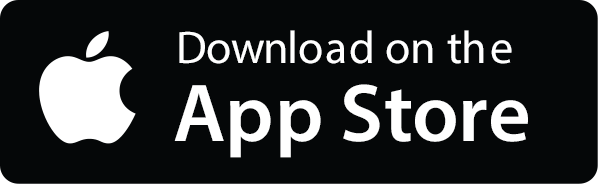
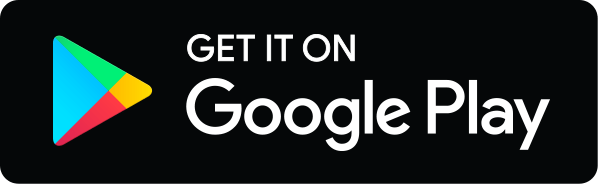