Master and Oppenheimer described the first stress test in 1929. Several methods of assessing myocardial ischemia have subsequently been developed, including those that incorporate electrocardiograms (ECGs), echocardiography, myocardial scintigraphy, and, most recently, cardiovascular magnetic resonance (CMR). Since 1987, stress CMR has been used to identify inducible ischemia, measure contractile reserve, and identify those at risk of future myocardial infarction (MI) and cardiac death. In this chapter, we review the utility of CMR wall motion stress testing, including the pharmacologic agents used during the procedure, the evolution of the procedure from dobutamine stress echocardiography (DSE), the performance and safety of a dobutamine stress CMR (DCMR) examination, and the efficacy of DCMR in identifying inducible ischemia, myocardial viability, and cardiac prognosis.
Intravenous Dobutamine and Atropine
Developed in 1975 by Tuttle and Mills, dobutamine is a synthetic beta-1-selective catecholamine agonist that binds to myocyte beta-1-receptors and increases intracellular calcium concentrations and myocardial contraction. Dobutamine was first employed as a stress-testing agent in 1984. In addition to enhancing myocardial contractility, it increases the heart rate by promoting peripheral vasodilation and a reflex tachycardia.
Because of rapid metabolism by catechol- O -methyltransferase, dobutamine’s onset of action and half-life are both approximately 2 minutes; inactive metabolites are readily excreted by the kidneys and liver. At low doses, <10 µg/kg/min, myocardial contractility is augmented and minor peripheral vasodilation occurs. As the dose increases (20–40 µg/kg/min), heart rate and myocardial oxygen demand are increased along with myocardial work. In study participants with flow-limiting epicardial stenoses, intravenous dobutamine promotes an oxygen supply/demand mismatch that induces left ventricular (LV) wall motion abnormalities.
Intravenous dobutamine increases myocardial oxygen demand in a fashion similar to exercise, and thus is useful for stress testing in study participants whose exercise ability is limited as a result of peripheral vascular disease, physical incapacitation, or chronic deconditioning. Other benefits of dobutamine include its tolerance for peripheral vein infusion and its effectiveness in both perfusion and wall motion imaging.
The common side effects associated with dobutamine administration for stress include chest pain, ventricular ectopy, dyspnea, nausea, hypertension, and arrhythmias. Despite its relative safety, dobutamine is specifically contraindicated in study participants with hypertrophic obstructive cardiomyopathy, epicardial luminal narrowings of >50% in the left main coronary arterial segment, severe aortic stenosis, second- and third-degree atrioventricular block, sudden death syndrome, supraventricular tachycardia, and previous episodes of dobutamine hypersensitivity.
Although dobutamine exhibits positive inotropic activity, its chronotropic response can be suboptimal. Studies using changes in LV wall motion to identify inducible ischemia exhibit heightened sensitivity in appreciating flow-limiting epicardial luminal narrowing when the heart rate response during testing exceeds 85% of the age-predicted maximum heart rate (APMHR) response (220 − age in years). For this reason, intravenous atropine may be needed to achieve an adequate peak heart rate response during testing. Atropine is a naturally occurring alkaloid that is a competitive antagonist of muscarinic cholinergic receptors; it increases heart rate by inhibiting vagal tone. It is particularly useful in study participants taking beta-blockers, rate-limiting calcium antagonists, or those possessing a high parasympathetic drive. Atropine may be administered in increments of 0.1 to 0.3 mg, up to a total of 2 mg, to facilitate the achievement of >85% the APMHR during testing. Common side effects of atropine include dry mouth, tachycardia, and hallucinations. Abnormally high heart rates after atropine administration are best treated with intravenous beta-blockers or rate-limiting calcium channel antagonists.
Safety Profile of Dobutamine and Atropine Stress Testing
Safety is a primary concern for physicians and health care providers who administer dobutamine/atropine stress examinations. The safety profile of dobutamine/atropine stress has been reported in 6 large studies, 3 using DSE and 3 using DCMR ( Table 17.1 ). Garcia et al., Picano et al., and Geleijnse et al. reported on the adverse events associated with DSE. These were classified into major and minor complications. Major complications included death, ventricular fibrillation, sustained ventricular tachycardia, complete atrioventricular block, acute MI, rupture of the LV free wall or ventricular septal defect, transient ischemic attack, and severe hypotension. Minor complications included nausea, anxiety, and atropine poisoning with hallucinations lasting up to several hours in the absence of either myocardial ischemia or hypotension.
Study | Modality | No. of Patients | Minor Events | Major Events | Deaths |
---|---|---|---|---|---|
Picano | DSE | 2799 | 78% | 5% | 0 |
Geleijnse | DSE | 2246 | 71% | 5% | 0 |
Garcia | DSE | 325 | 57% | 21% | 1 |
Hamilton | DCMR | 469 | 67% | 0% | 0 |
Kuijpers | DCMR | 400 | 71% | 3% | 0 |
Wahl | DCMR | 1000 | 64% | 6% | 0 |
Across the 3 DSE studies, the rates of major and minor complications ranged from 0.1% to 4%, and 38% to 71%, respectively. Wahl et al., Kuijpers et al., and Hamilton et al. have reported on the incidence of major and minor side effects associated with DCMR. In Wahl’s study of 1000 participants, 54% received atropine; in Kuijpers’ study of 400 participants, none received atropine; and in Hamilton’s study of 469 participants, 27% received atropine. There were no episodes of ventricular fibrillation, MI, or death during DCMR (see Table 17.1 ). The number of study participants sustaining other major events was 0.6%, 0.2%, and 0%, respectively. The percentage of participants sustaining minor events in all three studies combined was 54%. In these studies, the rates of major and minor events observed during DCMR are similar to those reported with DSE and would be expected as the dose administration is similar.
It is important to recognize that the major events are usually associated with continued administration of pharmacologic stress in the setting of concurrent myocardial ischemia. For this reason, it is important to identify ischemia promptly, and to discontinue the DCMR protocol when ischemia is recognized. In the studies reported above, many of the study participants (56%) who developed side effects had known coronary atherosclerosis and a diminished resting LV ejection fraction (LVEF). For this reason, a high level of scrutiny is used when reviewing image or ST-segment data for ischemia in the setting of reduced LVEF. Because of the risk of major adverse events, certain study participants should not receive intravenous dobutamine and atropine. Medications used for testing and emergency medications ( Table 17.2 ) for treatment of potential cardiac complications need to be readily available to improve the probability of a successful outcome should complications occur during DSE.
Stress Agent | |
Dobutamine | Stimulates beta-1-receptors to increase myocardial contractility and stroke volume; also acts as a peripheral vasodilator |
Atropine | Anticholinergic that inhibits acetylcholine at the parasympathetic neuroeffector junction, blocking vagal effects on the sinoatrial and atrioventricular nodes; enhances conduction through the atrioventricular node and increases the heart rate |
Adenosine | Peripheral and central vasodilator; increases heart rate and blood flow |
Treatment for Ventricular Dysrhythmia | |
Lidocaine | Decreases depolarization, automaticity, and excitability in the ventricles; can suppress ventricular dysrhythmia |
Procainamide | Decreases excitability, conduction velocity, automaticity, and membrane responsiveness with prolonged refractory period |
Treatment for Supraventricular Tachycardia | |
Metoprolol | Beta-1-antagonist that decreases myocardial contractility, heart rate, cardiac output, and blood pressure and reduces myocardial oxygen demand |
Diltiazem | Calcium channel antagonist; reduces myocardial contractility and sinoatrial node conductor |
Verapamil | Calcium channel antagonist; reduces myocardial contractility and oxygen demand; decreases atrioventricular nodal conduction rate; and dilates coronary arteries and arterioles |
Treatment for Chest Pain | |
Nitroglycerin | Reduces cardiac oxygen demand by decreasing left ventricular end-diastolic pressure (preload) and systemic vascular resistance (afterload) |
Aspirin | Analgesic/antipyretic that impedes clotting by blocking prostaglandin synthesis |
Heparin | Anticoagulant that accelerates the formation of antithrombin III–thrombin complex and deactivates thrombin, preventing the conversion of fibrinogen to fibrin |
Morphine sulfate | Opium derivative with primary effects on the central nervous system and organs containing smooth muscle |
Treatment of Allergic Reaction or Anaphylaxis | |
Diphenhydramine | Prevents histamine-mediated responses |
Hydrocortisone | Corticosteroid that decreases inflammation, mainly by stabilizing leukocyte lysosomal membranes and suppressing the immune responses |
Anxiolytic | |
Diazepam | Benzodiazepine with relatively long half-life that works through the immune system to exert a calming effect |
Midazolam | Water-soluble benzodiazepine with a short half-life exerts calming effect at low doses and a central nervous system depressant effect at high doses |
Reversal Agents | |
Flumazenil | Benzodiazepine receptor antagonist that can be used to reverse the effects of midazolam or diazepam |
Naloxone | Narcotic antagonist that can be used to reverse the effects of morphine sulfate |
Other | |
Furosemide | Loop diuretic for treatment of pulmonary congestion due to intravascular volume overload |
Albuterol (nebulizer) | Inhaled beta-agonist that causes bronchodilation; important for emergent treatment of bronchospasm |
Aminophylline | Relaxes smooth muscle of the bronchial airways and pulmonary blood vessels for treatment of bronchospasms |
Dobutamine Stress Echocardiography
Since 1977, transthoracic echocardiography (TTE) has been used to acquire images before, during, and after treadmill or bicycle exercise stress, or pharmacologically induced stress. During DSE, images are acquired in four standard imaging planes: parasternal short-axis (PSAX), parasternal long-axis (PLAX), apical four-chamber (A4C), and apical two-chamber (A2C) views. The most common dobutamine infusion protocol used involves initiating dobutamine at 5 to 7.5 µg/kg/min for a period of 3 minutes, followed by 10 µg/kg/min for 3 minutes and subsequent increments of 10 µg/kg/min in 3-minute increments to a maximal dose of 50 µg/kg/min. If the patient does not achieve 85% of their APMHR, supplemental atropine is administered in 0.1 mg to 0.3 mg increments per minute (up to 2.0 mg total) until the target heart rate is reached.
Throughout the test, the four echocardiographic planes are reviewed to monitor for ischemia. During testing, LV myocardial wall motion is defined as 1 = normal, 2 = hypokinesis, 3 = akinesis, and 4 = dyskinesis. Myocardial ischemia is defined as an increase in wall motion score during testing, or the persistence of severe hypokinesis during testing. The scoring system is determined using a 17-segment model defined by the American Heart Association and American College of Cardiology. A DSE is interrupted when there is evidence of ischemia in at least two adjacent segments, severe hypertension or hypotension, dysrhythmias, unstable angina, or unexpected neurologic findings.
DSE is useful for assessing risk of future MI, death, and the need for coronary intervention. For many years, it was the modality of choice for risk stratification of cardiovascular events for patients being considered for noncardiac surgery. However, DSE is of minimal value in study participants with poor acoustic windows, often because of a large body habitus, prior cardiothoracic surgery, or pulmonary disease. In addition, there is a high interobserver variability for image interpretation; consequently, the accuracy of the test depends heavily on the proficiency of both the sonographer (acquisition) and physician (interpretation).
Dobutamine Stress Cardiovascular Magnetic Resonance
CMR is well suited to overcome the acquisition and interpretative limitations encountered during DSE. It has no acoustic window limitations and thus can obtain comprehensive visualization of the LV myocardium regardless of body habitus. In addition, image acquisition can be standardized and there is less reliance on an individual technologist’s technique for acquiring high-quality images.
DCMR is commonly performed on short, 1.5 tesla (1.5 T) closed-bore systems, with bore diameters ranging from 55 to 70 cm. At the initiation of the DCMR examination, a 12-lead ECG is performed outside of the magnet. After intravenous access is established, study participants are told to lay flat on the CMR scanning table with a phased-array surface coil, brachial blood pressure cuff, pulse oximetry monitor, and respiratory gating belt attached ( Fig. 17.1 ). A physician and nurse are present throughout testing to continuously monitor the heart rate and rhythm, respiratory rate, oxygen saturation, and blood pressure. The protocol is similar to that described for DSE in that dobutamine infusions are started at 5 to 7.5 µg/kg/min, followed by 10, 20, 30, 40, and 50 µg/kg/min infusions in 3-minute stages. If study participants do not achieve 85% of their APMHR, atropine is added in 0.1 mg to 0.2 mg/min increments (up to 2 mg total) to augment the heart rate response ( Fig. 17.2 ).
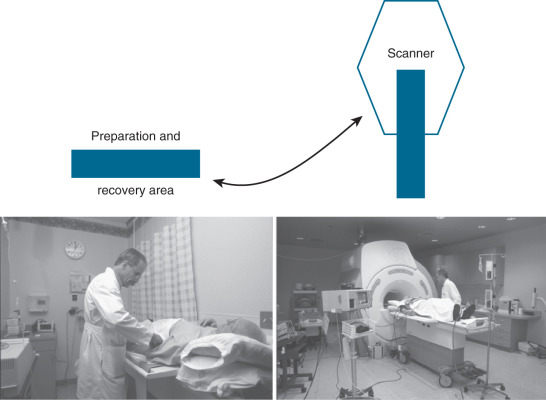
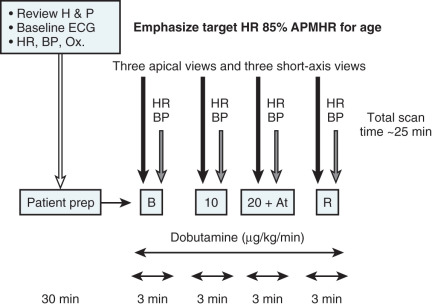
Breath-hold cine balanced steady-state free precession (bSSFP) images are obtained in three apical views (horizontal, vertical, and apical long axis) and in at least three short-axis LV planes (base, middle, and apex) at each level of pharmacologic stress ( Fig. 17.3 ). If Simpson’s rule is used to calculate LV volumes, short slices spanning the entire LV from base to apex can be acquired. These views are also obtained after 10 minutes of recovery to confirm that LV wall motion has returned to baseline. After the patient is removed from the magnet, the 12-lead ECG is repeated. The DCMR test is stopped if there is development of ischemia, as evidenced by a new wall motion abnormality ( Figs. 17.4 and 17.5 ): a fall in systolic blood pressure >40 mm Hg, significant ventricular arrhythmias, or achievement of the target heart rate of 85% APMHR. Although there are multiple image strategies available for the acquisition of bright-blood cine images, in general, most favor the use of bSSFP at 1.5 T, with multiple slices acquired during each breath-hold using parallel imaging techniques ( Table 17.3 ).
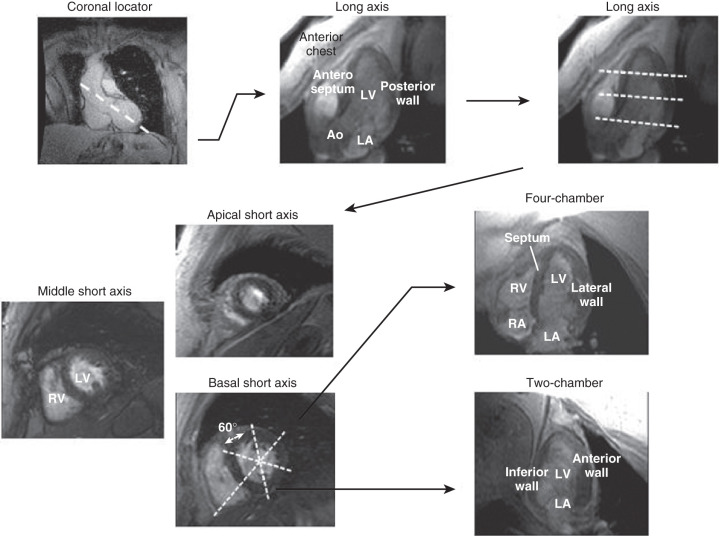
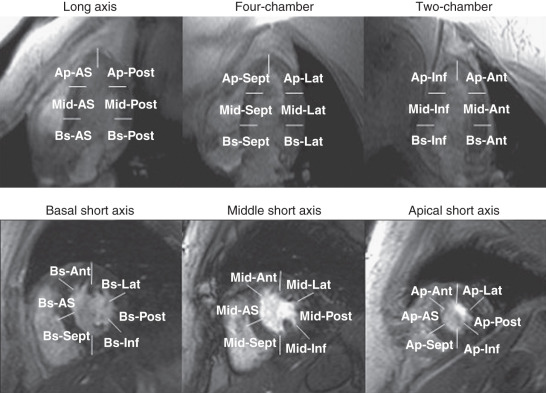
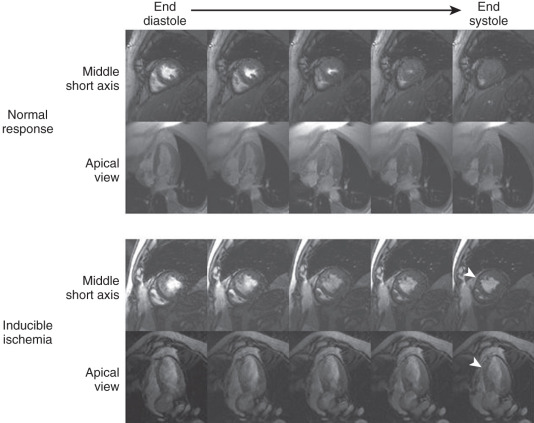
Technique | Advantage | Disadvantage |
---|---|---|
GRE (breath-hold) | Reliable ↑ heart rate | Breath-hold = 12 s |
GRE (respiration triggered) | No breath-hold | 45-s scan |
SSFP | Apical views | Artifact ↑ heart rate |
SENSE | Rapid acquisition | Unknown reliability |
Real time | Rapid acquisition | Noise, low spatial, and temporal resolution |
Utility of Dobutamine Stress Cardiovascular Magnetic Resonance for Identifying Inducible Ischemia
Investigators in the early 1990s began using DCMR to detect LV wall motion abnormalities indicative of ischemia. Pennell et al. were among the first to report the clinical use of DCMR ( Fig. 17.6 ). In this study, 25 study participants underwent DCMR and dobutamine thallium-201 single-photon emission computed tomography (SPECT), and x-ray coronary angiography. Study subjects received dobutamine infusions of up to 20 µg/kg/min and underwent conventional gradient recalled echo (GRE) cine CMR. Twenty of the 22 study participants with significant coronary artery disease (CAD) after angiography had wall motion abnormalities consistent with CMR, and 21 had reversible ischemia as identified by dobutamine thallium tomography. Thus the sensitivity of DCMR for detection of significant CAD was 91%. Between DCMR and dobutamine thallium tomography there was a 96% agreement at rest, 90% agreement during stress, and 91% agreement for the assessment of reversible ischemia. This study was the first to demonstrate that DCMR could be effectively used to assess regional LV systolic function in participants with CAD.
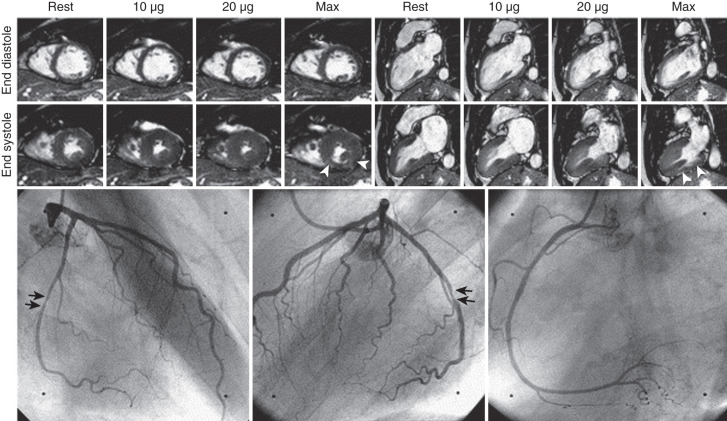
Subsequently, multiple studies have examined the utility of DCMR for a variety of clinical situations. Baer et al. reported on the utility of DCMR for individuals with no previous infarction or wall motion abnormalities by left ventriculography, but with high-grade coronary artery stenosis (≥70%). Twenty-eight subjects underwent wall motion assessment with DCMR (5, 10, 15, and 20 µg/kg/min at 1.5 T). Segmental wall motion analysis was performed in basal, mid, and apical short-axis planes, as well as two transverse planes. Each of 72 segments was related to a coronary artery territory and was graded as normal, hypokinetic, or akinetic. Eighty-seven percent of epicardial coronary artery narrowings were correctly identified in the left anterior descending (LAD), 62% in the left circumflex (LCX), and 78% in the right coronary artery (RCA) territories. Single vessel and multivessel disease was identified with 73% and 100% sensitivity, respectively. This study illustrated the utility of DCMR in individuals with known CAD, but with normal wall motion at rest.
Comparison of DCMR and SPECT for the detection of CAD was examined in another study by Baer et al. In 35 consecutive study participants, sensitivity for detection of CAD was 84% and 87% for CMR and SPECT, respectively. This comparison between the two techniques indicated that the efficacy of DCMR is maintained when compared with a well-established clinical tool.
Van Rugge et al. assessed the efficacy of quantitative and qualitative measures of LV wall motion during DCMR for identification and localization of myocardial ischemia in a study of participants with CAD. For the qualitative study of 45 study participants, 37 had CAD and 30 (81%) showed an LV wall motion abnormality during DCMR with a specificity of 100%; these values were superior to those from exercise electrocardiography (70 [63%]) or DSE (51 [63%]). Sensitivity for detection of single-, double-, or triple-vessel disease was 75%, 80%, and 100% respectively. In the quantitative portion of the study, 39 study participants with previously demonstrated LV wall motion abnormalities and 10 normal volunteers were assessed using a short-axis stress cine and analyzed with a modified centerline method. With preselected cuts, stress wall motion was considered abnormal if four or more adjacent cords (the LV was divided into a total of 100 cords) showed systolic wall thickening below two standard deviations of that obtained from the normal volunteers. This method had high sensitivity in detecting single- (88%), double- (91%), and triple-vessel (100%) disease, and in specifically detecting stenosis or specific abnormalities in the LAD (75%), RCA (87%), and LCX (63%). Unfortunately, there was no direct comparison between the qualitative and quantitative groups to determine if endocardial and epicardial tracing gave a significant diagnostic improvement. However, these studies demonstrated the utility of DCMR using both quantitative and qualitative methods.
Each of the early studies was relatively small, performed in a single institution, and enrolled participants in whom the investigators were aware of the extent of coronary artery luminal narrowings before stress CMR. LV wall motion was assessed at baseline and peak stress, and not visualized continuously throughout the course of stress testing. Infusions were terminated prematurely when study participants developed chest pain. Nevertheless, this series of small studies effectively demonstrated the feasibility and diagnostic utility of DCMR.
Over the last 15 years, advancements in computer software and CMR hardware have made it possible to study subjects with suspected CAD more easily, without awareness of the extent of CAD before testing. In 1999, Nagel et al. published a landmark study using high-dose dobutamine (up to 40 µg/kg/min) and atropine during DCMR to detect CAD; the efficacy of the test was compared with DSE. In this study, 208 participants (61 women, 147 men) underwent DCMR and DSE before x-ray coronary angiography. Dobutamine was infused intravenously at doses of 5, 10, 20, 30, and 40 µg/kg/min during 3-minute periods to achieve ≥85% of the APMHR, with atropine added as an adjunct if necessary. Regional LV wall motion was assessed using a 16-segment model; 50% of diameter or greater vessel stenosis was defined as CAD, which was detected in 107 of the study participants. Eighteen study participants could not be examined by DSE because of poor image quality and 18 could not be examined by DSMR (adipositas 6 and claustrophobia 11). Only 4 study participants failed to reach their target heart rate. Results indicated that the diagnostic accuracy of DCMR was higher when compared with DSE ( P < .05) because the specificity increased from 69.8% to 85.7%, and sensitivity increased from 74.3% to 86.2% ( Table 17.4 ). This was primarily related to patients with suboptimal DSE image quality. The authors concluded that wall motion abnormalities induced during DCMR could be detected with a significantly higher diagnostic accuracy compared with DSE in study participants with suspected CAD.
DSE | DCMR | P | |
---|---|---|---|
Sensitivity | 74% | 86% | < .05 |
Specificity | 70% | 86% | < .05 |
Positive predicting value | 81% | 91% | < .05 |
Negative predicting value | 61% | 78% | < .05 |
Accuracy | 73% | 86% | < .005 |
Whereas Nagel et al. compared DSE and DCMR directly, Hundley et al. used DCMR to study individuals who had poor TTE acoustic windows that prevented the use of second harmonic contrast-enhanced DSE imaging. One hundred and sixty-three subjects (74 women and 89 men) underwent DCMR after DSE was insufficient to view all endocardial segments as defined by the American Society of Echocardiography. Ten percent of study participants undergoing DSE had 5 to 8 and 90% had ≥8 endocardial segments not visualized with echocardiography. Study participants were excluded if they had intracranial metal, a pacemaker, claustrophobia, or a known contraindication to receiving dobutamine or atropine. Images were obtained in three apical (horizontal, vertical, and apical long-axis) views and three short-axis (base, middle portion, and apex) views of the left ventricle. Images were continuously acquired while 5-minute dobutamine infusions of 5, 10, 20, and 40 µg/kg/min were given to achieve 85% APMHR (with atropine administered in 0.3 mg/min increments if 85% APMHR was not reached). After 10 minutes of recovery, additional images were obtained to confirm any inducible LV wall motion had returned to baseline. Inducible ischemia was evident in 36 study participants and absent in 103 study participants; although 29 study participants developed chest discomfort, only 8% developed a concurrent new LV wall motion abnormality. In study participants who later underwent x-ray coronary angiography, the sensitivity and specificity for detecting a >50% luminal diameter narrowing were both 83%. Based on the results of this study, the authors concluded that DCMR provides a useful mechanism to diagnose inducible ischemia and assess LV contraction in study participants not well suited for stress echocardiography.
Stress echocardiography is difficult when subjects have resting LV wall motion abnormalities because there are false positives and a tendency for the observer to overinterpret. In many of the aforementioned studies, study participants with a prior MI or resting wall motion abnormalities were excluded. Wahl et al. evaluated a group of 160 participants to document the utility of DCMR in individuals with previously documented resting LV wall motion abnormalities. The subjects had prior revascularization with underlying resting LV wall motion abnormalities, and were difficult to assess with DSE because of the variability in interpreting LV wall motion with poor visualization. The study demonstrated that the sensitivity and specificity of DCMR for detecting coronary artery luminal narrowing ≥50% in this patient population was 89% and 84%, respectively ( Fig. 17.7 ). The sensitivity of detecting luminal narrowing of one, two, or three epicardial arteries was 87%, 91%, and 100%, respectively. Throughout this study, there was only a single major complication which resulted in external defibrillation. This study demonstrates that high-dose DCMR can be useful even in study participants with resting LV wall motion abnormalities and prior coronary artery revascularization.
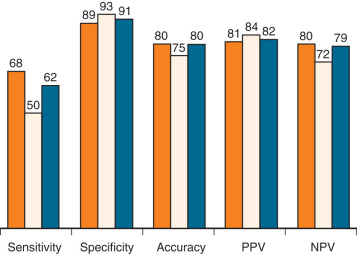
Many of the previous studies were unable to use single breath-hold techniques and consequently led to longer examination times and less accurate images. Paetsch et al. was able to use bSSFP sequences combined with parallel imaging acquisitions to acquire images to identify ≥50% coronary artery diameter narrowing with sensitivity and specificity of 89% and 80%, respectively. These bSSFP imaging techniques allow for the acquisition of multiple slice positions during a single breath-hold and therefore reduce scan time by a factor of 3 to 4 (see Fig. 17.6 ). As shown in Table 17.5 , the sensitivity and specificity of DCMR for identifying >50% coronary arterial luminal narrowings remains similar or higher to that observed with other noninvasive imaging techniques.
