Surgical Treatment for Congenital Heart Defects
2.1 Fundamentals of Surgical Treatment
Martin Kostelka
Farhad Bakhtiary
Friedrich Wilhelm Mohr
2.1.1 Evolution of Congenital Heart Surgery
The first successful surgical interventions for treating congenital heart defects were the correction of a PDA by Dr. Robert E. Gross at Children’s Hospital of Boston in 19381 and correction of an aortic coarctation in 1945.2 The decisive breakthrough in congenital cardiac surgery was first achieved with the development of the heart-lung machine, which made it possible to perform openheart surgery. Generally speaking, these surgeries were first performed after infancy. Anesthesia, intensive care, and surgical instruments for newborns were still in early stages of development, and before echocardiography was introduced, diagnosing congenital heart defects was dependent upon invasive techniques and their corresponding rates of complication. For this reason, gradual therapies with non-corrective palliative interventions were generally used instead of the early correction that is common nowadays. For simplicity’s sake, the pathophysiology of congenital heart defects can be reduced to the following main issues:
Volume overload: Right-side load in cases of septal defects (ASD, VSD) is one example of volume overload.
Pressure overload: This affects one or both ventricles and develops secondarily due to a stenosis in the outflow tract of the right or left ventricle.
Cyanosis: Cyanosis can develop secondarily as a result of reduced pulmonary artery flow or in cases of ineffective mixing between parallel operating circulatory systems, as in cases of TGA.
2.1.2 Cardiac Surgery for Congenital Heart Defects within the Scope of Gradual Therapy or Palliative Treatment
The following depiction of a surgical procedure serves as an example of the treatment process.
The goal of gradual therapy for congenital heart defects is to minimize the aforementioned pathophysiological issues. No complete correction of the congenital heartdefect occurs at a young age. This allows patients to grow and their size and weight to increase, thereby establishing the opportunity to operate upon these patients at an age where the risk of final surgical treatment is significantly lower.
Aortopulmonary Shunts
A systemic aortopulmonary shunt reduces cyanosis by increasing pulmonary arterial blood flow. Selecting an appropriate shunt size is a critical point during this usually technically simple procedure. A shunt that is too small can cause residual cyanosis, and a shunt that is too large can cause volume load or even the development of congestive heart failure. Shunt types include:
Blalock-Taussig shunt (▶Fig. 2.1): The Blalock-Taussig shunt was introduced by surgeon Alfred Blalock and cardiologist Helen Taussig at John Hopkins Hospital in Baltimore in 1947.3 In this surgery, the subclavian artery is anastomosed directly to the pulmonary artery in order to increase pulmonary blood flow. This method also allows the shunt to grow along with the child, but is very technically demanding (especially for small children), and is associated with the risk of early thrombosis. This was also one reason why other shunts that are technically easier to insert became more common.
Waterston shunt (▶Fig. 2.2): The Waterston shunt is an artificial connection between the ascending aorta and the right pulmonary artery. It is inserted into both vessels via partial unclamping within the scope of a right thoracotomy. The shunt’s size is crucial. Pulmonary volume overload occurs frequently. As the child grows, torsion or narrowing, primarily of the right pulmonaryartery, can also occur. Imaging can be helpful not merely for visualizing morphology, but also for determining shunt volume noninvasively.
Pott shunt (▶Fig. 2.3): The Pott shunt is an artificial connection between the descending aorta and the left pulmonary artery. It is inserted into both vessels via partial unclamping within the scope of a left thoracotomy. The Pott shunt has the same drawbacks as the Waterston shunt. In addition, it is often extremely difficult to remove it at a later date during final corrective procedures.
Pulmonary Artery Banding
VSD is, by far, the most common congenital heart defect. This defect leads to volume overload in both ventricles. If the defect is large and pulmonary resistance is also low, the patient will develop symptoms of congestive heart failure. Increased flow and pressure in the pulmonary arteries can lead to irreversible damage to pulmonary microcirculation, namely the development of pulmonary vascular disease. Muller and Darmann4 first described pulmonary artery banding to reduce flow and pressure (▶Fig. 2.4) in the 1950s. Similar to pulmonary artery shunts, pulmonary artery bands do not grow as the patient does. After a pulmonary artery band is placed, children can become cyanotic again as they grow. If scarring occurs, this can lead to distortion of both pulmonary arterial outflow tracts and pulmonary valves.
Atrial Septostomy
Cyanosis generally occurs due to reduced pulmonary blood flow. It can, however, also occur in cases of TGA. If neither PDA nor a septal defect is present, the newborn will die as a result of cyanosis. Blalock and Hanlon described a refined method in 1950: they surgically removed a large portion of the atrial septum in order to create an iatrogenic ASD in newborns with TGA. After the introduction of the Rashkind balloon septostomy, also known as the Rashkind maneuver (▶Fig. 2.5) in 1966,5 the Blalock and Hanlon surgical method is seldom used.
Surgical Treatment of Functional Univentricular Heart
A general goal in pediatric cardiac surgery is to correct the congenital heart defect as soon as possible. Biventricular correction, however, is often not possible. If only one functional ventricle is capable of independently sustaining one of the two circulatory systems—either systemic or pulmonary—independently, this is known as “univentricular heart.” The most common forms of a functional univentricular heart occur due to the following defects:
Tricuspid atresia
DIV
HLHS
Pulmonary atresia with intact septum
Heart with a univentricular atrioventricular connection
Straddling atrioventricular valves
Unbalanced hearts (AVSD)
The umbrella term “hypoplastic left heart syndrome” encompasses a broad spectrum of heart defects with varying degrees of expression of the underdevelopment of the left ventricular structures:
Aortic valve atresia
Aortic stenosis
Mitral stenosis
Mitral valve atresia
Hypoplasia of the left ventricle
Lack of left ventricle
Pronounced hypoplasia of the ascending aorta (a diameter smaller than 3 mm in 55% of cases)
Aortic coarctation (in 80% of cases)
The most commonly associated defects are IAA, TAPVR, or an abnormal atrioventricular valve.
In cases of univentricular heart, the main treatment goals are to separate systemic and pulmonary circulation, ensure normal volume load for the functional singular ventricle, and reach normal arterial saturation. Surgical management generally occurs in three steps:
Up to the age of 7 days, only a palliative surgery is performed, regardless of functional status. In cases of pulmonary overflow, pulmonary artery banding is performed (▶Fig. 2.4), while a Blalock-Taussig shunt is placed in cases of deficient pulmonary perfusion (▶Fig. 2.1). HLHS presents as a very specific anatomical and functional entity that can generally be treated neonatally using the complex Norwood I operation (▶Fig. 2.6). During this surgery, a neoaorta and an aortopulmonary shunt are placed, and an atrial septostomy is performed.
The second step, generally occurring at the age of 4-6 months, is the Glenn procedure, meaning placement of a bidirectional cavopulmonary connection (▶Fig. 2.7).
The last step, generally occurring at the age of 3-4 years (at a body weight of 12 kg), is placement of a TCPC. This step completes Fontan circulation. The TCPC can be placed intracardially, with a GORE-TEX tunnel, through the right atrium. Alternatively, it can be placed extracardially, which has become the preferred surgical technique for avoiding intraatrial manipulation. The extracardiac tunnel is placed outside of the right atrium, between the inferior vena cava and the right pulmonary artery, and connected to the superior vena cava (▶Fig. 2.8).
2.1.3 Early Correction vs. Gradual Treatment
Drawbacks of Gradual Therapy for Congenital Heart Defects
All aforementioned palliative interventions render a thoracotomy necessary. The latter can lead to scarring in the mediastinum and vessels, distortion, or even dissection. Furthermore, gradual therapy always means a minimum of two interventions, and thus an additional burden.
Benefits of Early Correction of Congenital Heart Defects
Early correction of congenital heart defects has only become possible with the introduction of open-heart surgery. Temporary interruption of circulation, supported by hypothermia, allows early correction of less complex congenital heart defects. The first complex cardiac surgical interventions—TOF or AVSD—were performed either via cross-circulation (in which one parent was utilized as oxygenator6) or cardiopulmonary bypass. In the 1950s and 1960s, however, the two-step treatment with initial palliative and subsequent corrective intervention remained standard for older patients.
Brian Barrat-Boyes and his successor Castañeda completed numerous procedures at Children’s Hospital of Boston using a heart-lung machine in 1972. The introduction of Prostaglandin E1 in the late 1970s made it possible to hold the ductus arteriosus or ductus Botalli open in newborns purely via medication, and thus achieve preoperative stabilization for the early correction of certain congenital heart defects. Optimizing invasive and noninvasive diagnostics also improved the conditions for early surgical correction.
The differences between the right and left ventricle do not develop until shortly after birth. Compared to the left ventricle, the right ventricle is significantly thinner. The left
ventricle rapidly increases in thickness and mass. This is achieved by hyperplasia and hypertrophy of the left ventricular myocytes. The myocytes’ ability to divide is believed to persist only until the third month of life. Developing new myocytes within a growing individual requires the concurrent formation of new coronary arteries by means of angiogenesis. Muscular hypertrophy, e.g., sustained high blood pressure, reduces the ability to form collateral arteries. Cardiac development can be disrupted by the palliative intervention, itself. The best-known examples are a large VSD accompanied by tetralogy of Fallot, which must then be treated using a shunt, and complete atrioventricular canal that must then be treated via pulmonary artery banding. In these cases, the right ventricle is exposed to pressure overload—with the corresponding hypertrophy—and thus assumes a round form rather than a semicircular one.
ventricle rapidly increases in thickness and mass. This is achieved by hyperplasia and hypertrophy of the left ventricular myocytes. The myocytes’ ability to divide is believed to persist only until the third month of life. Developing new myocytes within a growing individual requires the concurrent formation of new coronary arteries by means of angiogenesis. Muscular hypertrophy, e.g., sustained high blood pressure, reduces the ability to form collateral arteries. Cardiac development can be disrupted by the palliative intervention, itself. The best-known examples are a large VSD accompanied by tetralogy of Fallot, which must then be treated using a shunt, and complete atrioventricular canal that must then be treated via pulmonary artery banding. In these cases, the right ventricle is exposed to pressure overload—with the corresponding hypertrophy—and thus assumes a round form rather than a semicircular one.
![]() Fig. 2.7 Norwood II or Glenn operation. Schematic depiction of the Norwood II operation with placement of a bidirectional cavopulmonary anastomosis instead of an aortopulmonary shunt (▶Fig. 2.6). A = atrium Ao = aorta IVC = inferior vena cava LPA = left pulmonary artery RPA = right pulmonary artery SVC = superior vena cava V = ventricle |
This altered geometry has considerable consequences for the tricuspid valve, whose tendinous cords (unlike those of the mitral valve) insert primarily into the ventricular septum. These cases often result in tricuspid valve insufficiency, meaning that volume overload of the right ventricle occurs in addition to pressure overload.
Early correction, on the other hand, allows the pulmonary vessels to develop normally during the first year of life. Pulmonary resistance is reduced with the involution of the smooth musculature in the arterioles. If pulmonary arterial pressure and flow are not reduced within the first 1-2 years of life, this can begin to contribute to the development of pulmonary hypertonia. Generally speaking, a child is considered inoperable if pulmonary vessel resistance has exceeded threefourths of systemic resistance and remains fixed. From that point onward, the child becomes increasingly cyanotic due to reduced pulmonary blood flow. The initial left-right shunt can convert to a right-left shunt. This phenomenon is known as the Eisenmenger reaction or Eisenmenger syndrome. Palliative interventions are meant to prevent this conversion. Pulmonary bands are placed to maintain pressure well below that of half of systemic pressure.
2.1.4 Cardiac Surgical Treatment for Common Congenital Heart Defects
Certain surgical procedures are described below as examples of treatment processes.
Surgical Treatment of a Ventricular Septal Defect
Indication for surgery—in the sense of large left-right shunts and pulmonary overflow—occurs in infancy for all cases of hemodynamically relevant ventricular septal defects.
The usual route to VSD correction consists of a median sternotomy with subtotal, bilateral thymectomy, longitudinal perdicardiotomy, and removal of the pericardial portion for later use as a VSD patch. VSDs are treated primarily with the assistance of a heart-lung machine and under conditions of mild-to-moderate hypothermia. In addition, the ascending aorta and the superior and inferior hollow veins are cannulated. After stabilizing the flow of the heart-lung machine, the ascending aorta is clamped off. A cardioplegic solution is used to induce cardiac arrest, which is then concluded with the closure of both hollow veins.
For children with pulmonary hypertonia, the PDA is inspected and may be clamped off. During cardiac arrest, a longitudinal incision is made in the right atrium. The fossa ovalis, tricuspid valve, and coronary sinus are then inspected. The type (membranous or muscular) and position of the VSD with respect to the aforementioned structures determine how surgery proceeds:
In cases of perimembranous VSD, both intraoperative representation and patch closure are possible via the tricuspid valve; a portion of the anchoring stitches can
be placed through the tricuspid valve ring. At the end of the procedure, the valve must be tested and the surgery may need to be supplemented by a commissuroplasty of the tricuspid valve.
A high membranous or subarterial VSD may be reachable via the pulmonary valve and closed with a pericardial patch.
In cases of infundibular muscular VSD, a right ventriculectomy with RVOT reconstruction and an additional pericardial patch (▶Fig. 2.11d) is needed.
Muscular apical VSDs cannot be reached via the tricuspid valve. They must be accessed via a right or left apical ventriculectomy in order to achieve adequate view of the VSD margins during the operation, so that a safe patch closure can be performed.
Surgical Treatment of Transposition of the Great Arteries
The arterial switch operation, also known simply as “arterial switch,” is definitively one of the greatest success stories in pediatric cardiac surgery. Before the arterial switch operation was established by Jatene et al. in 1975,7 surgical treatment consisted of the choice to perform physiological correction via either a Mustard8 or Senning atrial switch operation. The blood from the superior and inferior hollow veins was diverted into the anatomic left ventricle via the mitral valve using a venous baffle (▶Fig. 2.9). This blood was then transported into the lungs so that the blood from the pulmonary veins was pumped into the aorta via the right atrium and right ventricle.
The weaknesses in non-anatomical surgical atrial switch surgical procedure have been proven over the long term; among other factors, patients suffer from rhythm disorders due to surgical manipulation of the atria in order to construct the venous baffle (bradyarrhythmia, sinus node dysfunctions, tachyarrhythmia, especially atrial reentry tachycardia [occurring in 50% of cases after approximately 20 years]) and subsequently die of sudden cardiac arrest. “Right heart failure” is typical, as the morphologic right ventricle now functions as a systemic ventricle. Additional common complications, which must be taken into consideration with the help of imaging procedures, include tricuspid valve insufficiency, baffle stenoses, and baffle leaks.9
Despite the intermittently increased rate of early mortality for the arterial switch operation, which is generally performed in infancy during the first (5-10) days of life and is thus extremely technically demanding, anatomical correction has established itself due to its significant benefits over the long term.
The arterial switch operation is performed as follows: after a median sternotomy and subtotal bilateral thymectomy (▶Fig. 2.10a) have been performed, a piece of the pericardium is removed (▶Fig. 2.10b) to be used for reconstructing the neopulmonary artery (▶Fig. 2.10e) after the coronary button has been removed (▶Fig. 2.10c and d). It may also be used to close the concurrent ASD. The pulmonary arteries are exposed up to the bifurcation, and the ductus arteriosus is dissected. After being attached to the heart-lung machine via bicaval cannulation, the left atrium is drained via the right superior pulmonary veins using a vent catheter, supported by the superior position of the aortic cannula (nearly in the aortic arch). During a total bypass, the patient is cooled to a state of moderate hypothermia. The blood flow within the pulmonary arteries has to be controlled and the ductus arteriosus doubly suppressed, divided, and overstitched. After cardioplegic cardiac arrest, the aorta is transected approximately 2 cm above the valve, and the commissures are marked with three retaining stitches. At the same time, the aorta is stretched. The area between the aorta and the pulmonary artery is dissected completely. Both coronary buttons are excised, taking care to protect the semilunar valves, and retaining stitches are placed. The defect in the aortic wall is covered using a pericardial patch of the appropriate size (▶Fig. 2.10f). After transection of the pulmonary artery, the pulmonary valve is stretched using three retaining stitches. Then, based on the position of the commissures and the size, position, and anatomy of the coronary button, the pulmonary arterial root is reconstructed and the coronary buttons are sewn into the neoaorta, so that they are neither exposed to tension nor possess too much clearance. The aorta is closed using the Lecompte maneuver,10 namely displacement of the pulmonary arterial bifurcation ventral to the aorta (▶Fig. 2.10g). If the ASD is closed either directly or with a pericardial patch, the aortic clamp is removed, enabling coronary perfusion. The pulmonary artery is stitched on the beating heart (▶Fig. 2.10h), and the atrium is then closed. The chest cavity is either closed or left open depending on the patient’s hemodynamic and respiratory condition.
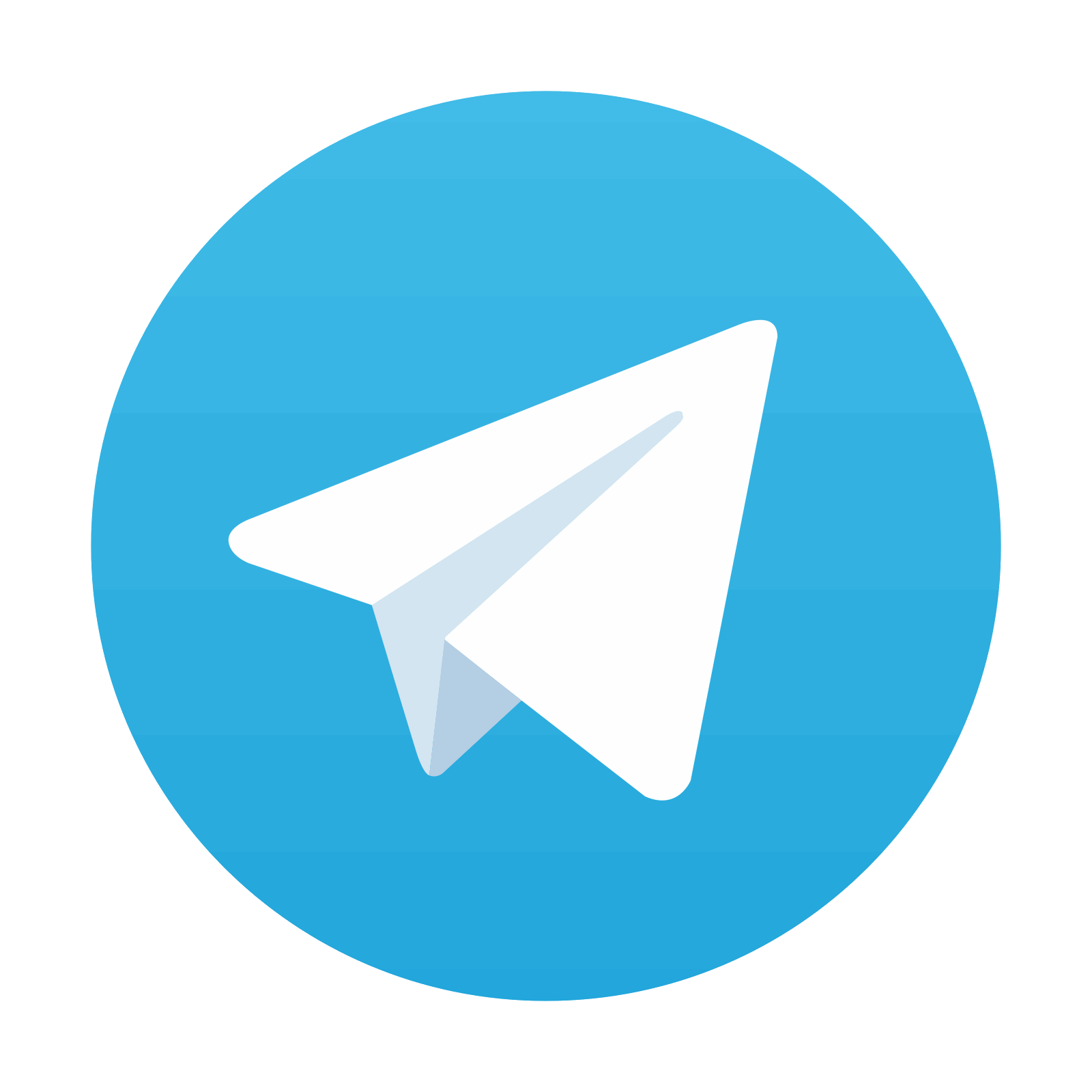
Stay updated, free articles. Join our Telegram channel

Full access? Get Clinical Tree
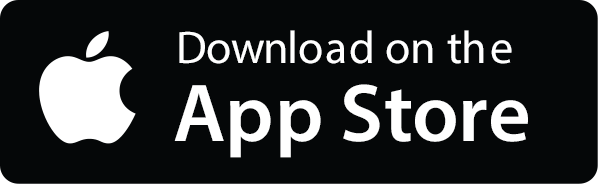
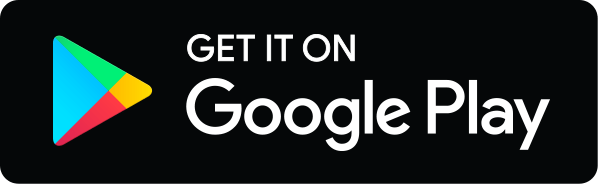
