Fig. 23.1
Pedestrian-invested: bilateral, inhomogeneous, ground-glass opacification for lung contusions
But when a precise evaluation for the first diagnosis is needed, referral to CT is mandatory [16] (Fig. 23.2).
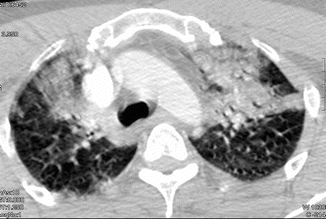
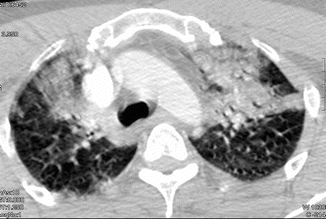
Fig. 23.2
Car crush: at MDCT, wide, dense, confluent anterior consolidations, for lung contusions. Typically, contusions are distributed according to impact force, as in this case anteriorly
Instead at the stage of follow-up, the development of a bedside noninvasive method is desirable.
Ultrasound could be such a method, but the lungs are traditionally deemed to be inaccessible [17].
However, it has been shown that a whole ultrasound semiology is available at the lung level if one takes care to analyze the artifacts [18]. Although indications for ultrasound of the lungs are increasingly numerous in ICUs, its clinical use is not yet fully developed.
By ultrasonography, lung contusions were defined as:
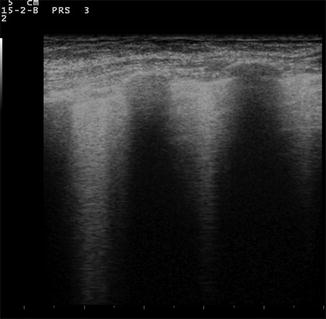
- (a)
A moderately hypoechoic blurred consolidation, with indistinct margins, whose dimensions remained unchanged during the inspiration phase. Internal hyperechoic punctiform images represent air bronchograms.
- (b)
Multiple (at least three) vertical hyperechogenic lines (B-lines) arising from and perpendicular to the pleural surface representing the involvement of interstitial spaces (Fig. 23.3). The distance between the B-lines can be 7 mm or 3 mm corresponding to the thickened interlobular septa and the ground glass areas, respectively.
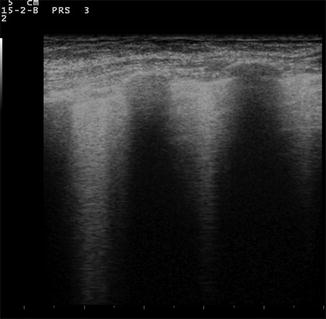
Fig. 23.3
Car crush: at lung ultrasound, anterior, bilateral, confluent B-Lines. The B-lines are “ring-down” artifacts that originate from the pleural line with vertical propagation
During follow-up, bedside chest radiography was a poor predictor of the extent of lung contusions compared with lung ultrasonography; CXR is less accurate and with a less sensitivity than LU (Accuracy CXR about 60–70% vs. LU about 80–90%). These data support the routine use of lung ultrasound (eventually in association with CXR) in the management and follow-up of thoracic trauma patients in the ICU. The information obtained from LU, that can show reduction/increase of B-lines and/or consolidations, was adequate to support therapeutical decisions in those patients, suggesting the possibility for a reduced need for further CT scans. However, we must recall the impossibility of a complete chest exploration with US probe, owing to the presence of tubes and wound medications was the main disadvantage of this method.
Limitations of ultrasound should be considered, for example in cases of small consolidations, if care is not taken to scan the entire lung surface, or by consolidations which do not reach the pleural surface, a rare finding, or by a poor echogenicity of the patient. Parietal emphysema, pleural calcifications, or dressings will prevent analysis. Furthermore, alveolar consolidations and B-lines are present in ARDS too, with the same semiotic aspects of lung contusions; only clinical background can differentiate the two different diseases.
Obviously, CT is considered the best imaging technique for visualizing the pulmonary interstitium involvement and alveolar consolidations in lung contusions; but while in less severe cases, repeated CT scans can be performed without major risks, the transporting of mechanically ventilated trauma patients to the CT room can be dangerous: several studies suggest that even intrahospital transfers of critically ill patients are associated with complications [19, 20], the most common and harmful being are hypoxemia and hypotension.
23.3 The Follow-Up of ARDS
The most difficult differential diagnosis is between cardiogenic pulmonary edema; the lung in acute respiratory distress syndrome is characterized by a marked increase in lung tissue and a massive loss of aeration. The former is homogeneously distributed, although with a slight predominance in the upper lobes, whereas the latter is heterogeneously distributed.
The lower lobes are essentially non-aerated, whereas the upper lobes may remain normally aerated, despite a substantial increase in regional lung tissue.
The overall lung volume and the cephalocaudal lung dimensions are reduced primarily at the expense of the lower lobes, which are externally compressed by the heart and abdominal content when the patient is in the supine position.
23.3.1 Radiological Features
Two opposite radiologic presentations, corresponding to different lung morphologies, can be observed.
In patients with focal computed tomographic attenuations, frontal chest radiography generally shows bilateral opacities in the lower quadrants and may remain normal, particularly when the lower lobes are entirely atelectasis (Fig. 23.4).
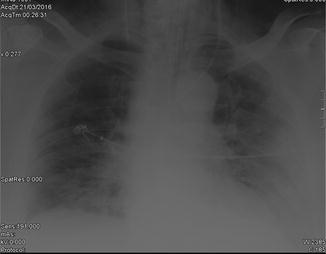
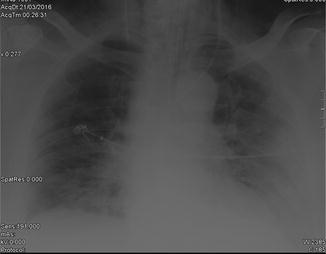
Fig. 23.4
Car crush: 3 days later, at Chest-XR, appearance of bilateral, inhomogeneous, patchy opacifications suggestive of ARDS
In patients with diffuse computed tomographic attenuations (Fig. 23.5), the typical radiologic presentation of “white lungs” is observed. If these patients lie supine, lung volume is preserved in the upper lobes and reduced in the lower lobes, although the loss of aeration is equally distributed between the upper and lower lobes.
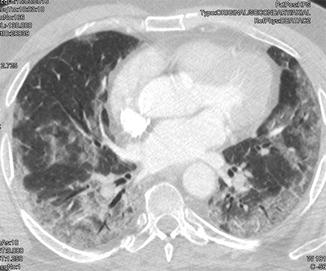
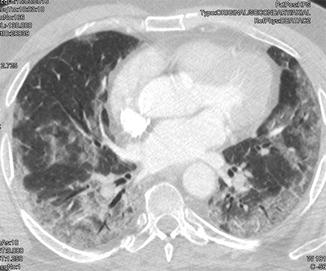
Fig. 23.5
Car crush: 3 days later, MDCT showed clearly appearance of bilateral, inhomogeneous, patchy opacifications suggestive of ARDS; within the areas of consolidation is also visible the air bronchogram. Asymmetric distribution of opacifications is characteristic of ARDS
In fact, interstitial edema, alveolar flooding, or both, not collapse, are histologically present in all regions of the lung in acute respiratory distress syndrome. Compression atelectasis is observed only in caudal parts of the lung, where external forces (such as cardiac weight, abdominal pressure, and pleural effusion) tend to squeeze the lower lobes.
Ultrasound can visualize a succession of B-lines, sign of interstitial involvement, with spared areas, and posterobasal consolidations for atelectasis areas (Fig. 23.6).
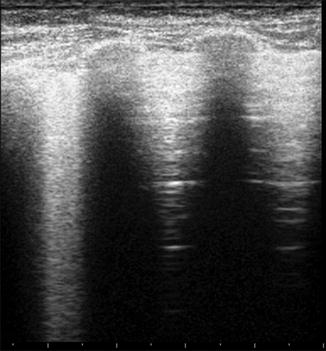
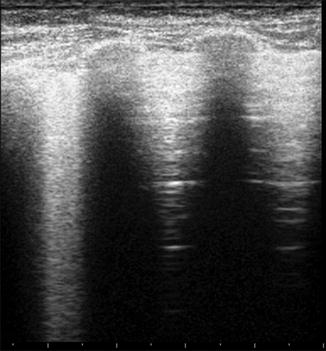
Fig. 23.6
Car crush: 3 days later, lung ultrasound confirmed alternance of B-lines and A-lines, named spared areas; signs suggestive of ARDS; the ultrasound imaging is similar to TC
When a positive intrathoracic pressure is applied to patients with focal acute respiratory distress syndrome, poorly aerated and non-aerated lung regions are recruited, whereas lung regions that are normally aerated at zero end-expiratory pressure tend to be rapidly overinflated, increasing the risk of ventilator-induced lung injury, like pneumothorax.
Selection of the optimal positive end-expiratory pressure level should not only consider optimizing alveolar recruitment, it should also focus on limiting lung overinflation and counterbalancing compression of the lower lobes by maneuvers such as appropriate body positioning. Prone and semirecumbent positions facilitate the reaeration of dependent and caudal lung regions by partially relieving cardiac and abdominal compression and may improve gas exchange.
For these reasons, CT is essential to monitoring all exchanges [21] (Fig. 23.7); computed tomography allows an accurate assessment of the volumes of gas and lung tissue, respectively, and lung aeration. If computed tomographic sections are contiguous from the apex to the lung base, quantitative analysis can be performed either on the whole lung or, regionally, at the lobar level.
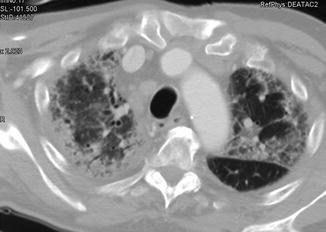
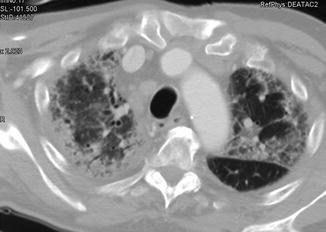
Fig. 23.7
Car crush: 15 days later, MDCT showed early signs of subpleural interstitial thickening, bronchial dilatations, suggestive of fibrosal evolution of ARDS
Analysis requires a manual delineation of lung parenchyma and is facilitated by software, including a color-coding system that allows direct visualization of overinflated, normally aerated, poorly aerated, and non-aerated lung regions.
In addition, lung recruitment can be measured as the amount of gas that penetrates poorly aerated and non-aerated lung regions after the application of positive intrathoracic pressure.
In ARDS, the radiographic abnormalities follow a sequence by tending to mirror the histopathological changes; in the first 24 h following insult, when there is a little alveolar edema, the chest X-ray is generally normal. As ARDS evolves, widespread ground-glass opacification invariably becomes apparent and, during the next 36 h, there is a frank consolidation on the chest radiograph (Fig. 23.8); obviously this is a nonspecific radiological sign.
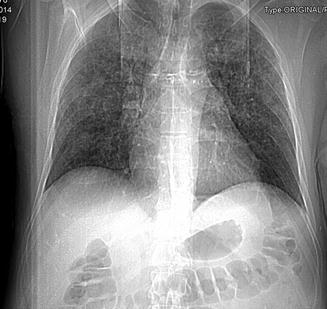
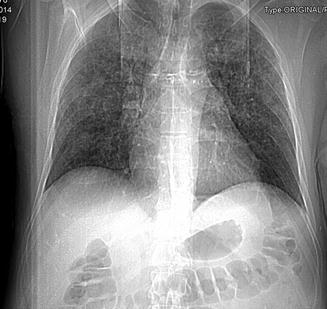
Fig. 23.8
Pedestrian-invested: after 3 days, most evidence of bilateral, inhomogeneous, ground-glass opacification, with appearance of fine micronodular opacities, suggestive for adipose embolism in ARDS
The radiographic changes of uncomplicated ARDS usually plateau after initial catastrophic burst and remain unchanged for a variable time thereafter. Knowledge of this radiographically stable phase can be of practical value to the radiologist; any significant change in serial radiographic appearances like the development of new focal areas of air space opacification may be the harbinger of nosocomial pneumonia.
In the final phase, the chest radiographic abnormalities begin to resolve. Although the lungs may revert to normal, there may be coarse reticular opacities and cysts, likely to be a consequence of the effects of both lung repair and barotrauma; this phase will already start after 8–10 days and requires CT for diagnosis and follow-up [22].
Interstitial emphysema, pneumothorax, and pneumomediastinum may be relatively frequent iatrogenic complications of mechanical ventilation support; thoracic ultrasound permits an accurate and early diagnosis of pneumothorax but not of the two other conditions that require CT.
An important finding of earliest CT studies was that lung involvement in ARDS is totally inhomogeneous, a “patchy” distribution intermittent with normally aerated lung (Fig. 23.9); furthermore the dependent (dorsal) region are more densely opacified than the nondependent (ventral) lung, with a gradient of density [23].
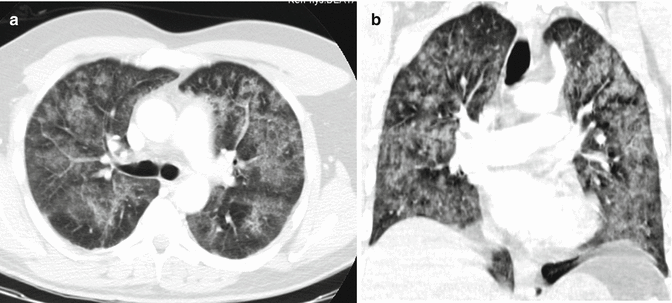
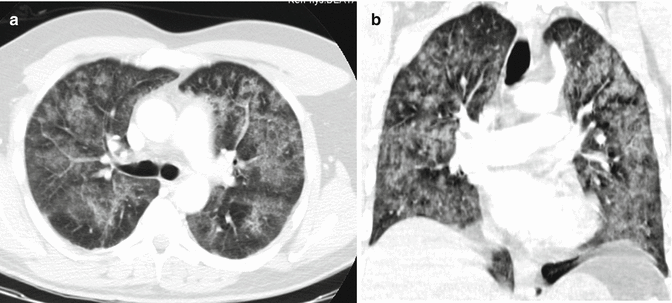
Fig. 23.9
(a) Axial CT scan; (b) coronal reconstruction. Pedestrian-invested: after 3 days, “cottony” opacifications with spared subpleural regions; signs suggestive of adipose embolism in ARDS
It’s a common thinking that appearance of nondependent opacities may represent foci of organizing pneumonia. ARDS appears to have a far more diffuse opacity than that associated with congestive heart failure; there are many signs to differentiate the two conditions and the principle is pulmonary picture really inhomogeneous in ARDS.
Bronchial dilatation is seen frequently on CT in patients with ARDS; airway dilatation persists in the majority of survivors and is generally associated with ground-glass opacification. The most common abnormality on CT in survivors is a reticular pattern seen in about 85% of patients [24]. The extent of the reticular pattern, that has a predilection for nondependent lung, at follow-up was negatively correlated with the extent of dense parenchymal opacification (in dependent lung) during acute phase; maybe, atelectasis somehow protects dependent regions from the long-term effects of barotrauma (see Fig. 23.5).
One of the principal advantages of CT is that the global extent of parenchymal abnormalities can be quantified accurately and followed-up; furthermore, the effects of PEEP (Positive End-Expiratory Pressure), of recruitment maneuvers, of prone positioning, can be studied using CT.
23.4 The Follow-Up of Pneumothorax
Pneumothorax is one of the most common injuries in thoracic trauma resulting in an abnormal accumulation of gas within the pleural space [25]. This is a virtual space normally filled with a few milliliters of fluid with a negative intrapleural pressure, usually about −5 cmH2O. In case of pneumothorax, the entry of air into the pleural space compromises the normal dynamics of airflow by compressing the lung that collapses.
Post-traumatic pneumothorax can happen in several chest traumas, like blunt or penetrating trauma, as isolated lesion or in polytrauma patient, with an incidence of 30–40% [26].
Often the presence of pneumothorax, especially if greater than 20%, requires a chest tube insertion to ensure the drainage of air from the pleural space and allow the lung re-expansion [27].
Follow-up of pneumothorax is required after chest tube placement by chest radiographs or, sometimes, by MDCT, to monitor the clinical condition and to identify the etiology of a persistent air leak [28] (Fig. 23.10).
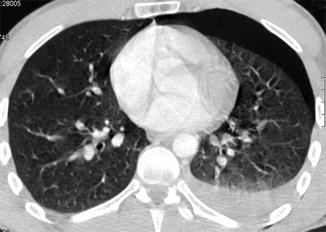
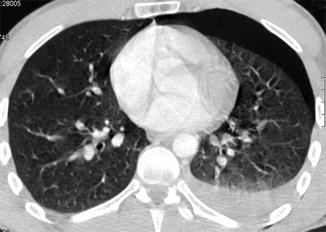
Fig. 23.10
Moto-car crush: at MDCT wide left anterior pneumothorax. Left posterior hemothorax is associated in this patient
A persistent pneumothorax, in fact, is a condition in which a continued bubbling of air from an in situ chest drain, for more than 48 h after its insertion [29], that can make several difficulties in diagnostic iter, aimed at its recognition and at the identification of potentially treatable etiologies. The most common etiologies may be chest tube related, like malposition or kinking or obstructing (Fig. 23.11), requiring a simple repositioning of the chest tube/drain, whereas lung parenchymal disease, bronchopleural fistula (BPF), alveolar-pleural fistula (APF) or, rarely, esophageal-pleural fistula, especially in penetrating thoracic injury, sometimes not immediately or easily discernible, requires a careful interpretation of imaging studies to make the right diagnosis and to guarantee an optimal therapeutic management.
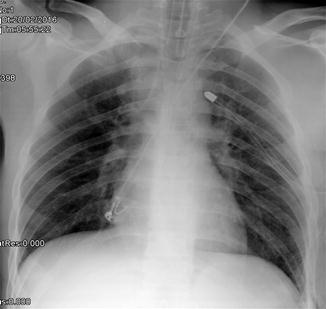
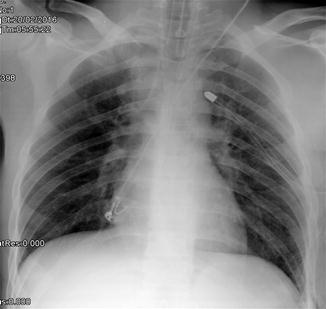
Fig. 23.11
Moto-car crush: kinking of left pleural drainage; residual basal hyperlucency and minimal deep sulcus sign, suggestive of residual left anterior pneumothorax
In these cases, in fact, although radiographs remain the mainstay for diagnosis and follow-up of pneumothorax, able to identify chest tube-related causes of persistent pneumothorax, the use of MDCT is essential to lead to early and proper diagnosis and treatment of non-chest tube-related cases.
The radiologic features of pneumothorax are encoded and represented by typical characteristics on RX, US, and TC imaging.
In fact, in trauma patients, usually chest radiograph is the primary modality imaging performed in emergency department, able to identify pneumothorax as a pathological lucency and a visceral pleural line separating the lung, occasionally completely collapse, from the chest wall; in supine chest radiographs, presence of a deep sulcus sign is useful to make the right diagnosis [30].
However, sensitivity of supine chest radiography in traumatized patients, especially in the presence of subcutaneous emphysema along chest wall, is very low, showing low accuracy in the assessment of pneumothorax during the primary survey in the emergency room [31, 32] because free air collects anteriorly, medially, and basally, therefore difficult to identify on supine chest radiographs [33].
Nowadays, however, the use of thoracic ultrasound is been approved; in fact, it has gained a main role in unstable patients with chest trauma during the primary survey in the emergency room for diagnosis of PNX, with high sensitivity (77%) and specificity (99.8%), positive predictive value 98.5%, negative predictive value 97%, accuracy 97.2% [6], useful to guide the chest drainage and in follow-up of persistent pneumothorax too [34, 35].
In healthy patient, there is a movement due to sliding of the two pleural sheets during breathing. This movement is precisely called “sliding or gliding.” The of loss of sliding movement due to the presence of air in pleural space, in fact, indicates the presence of pneumothorax [36], but nevertheless it isn’t adequate to identify the etiology of pneumothorax, nor even of a persistent pneumothorax (Fig. 23.12).
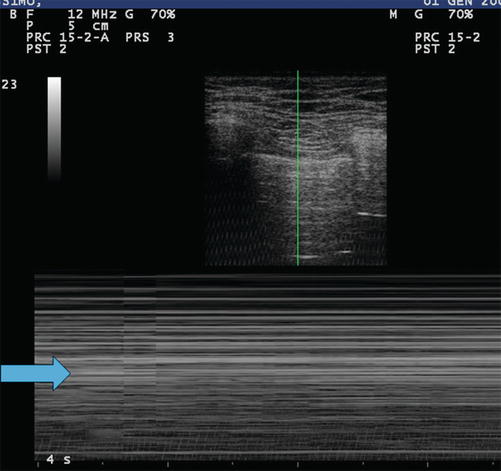
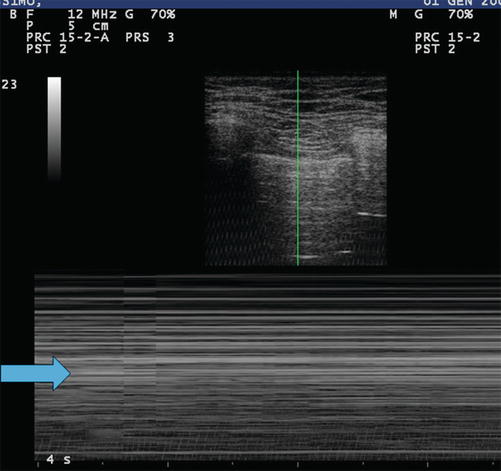
Fig. 23.12
Moto-car crush: at lung ultrasound, with M-Mode, “stratosphere sign” and absence of pleural sliding suggestive of residual left anterior pneumothorax
In these conditions, in fact, computed tomography (MDCT) is the best choice in detecting the main features of a persistent pneumothorax because, although the other imaging techniques consent to make diagnosis of PNX, only CT, thanks to its high sensitivity and specificity, its rapidity and its post-processing potentiality as Multiplanar reconstruction (MPR), maximum intensity projections (MIP), minimum intensity projection (minIP), volume rendering, and virtual bronchoscopy, is useful to identify other chest trauma related pathologies as contusion or the etiology of a persistent air leak [37, 38], allowing surgical planning too.
In case of persistent pneumothorax, in fact, follow-up CT, usually employing intravenous contrast, consents to evaluate and to distinguish the possible causes as malposition of chest tubes, or an air leak due to mediastinum or pleural laceration or, even rarely, an esophago-respiratory fistula, usually detected after ingestion of positive oral water-soluble contrast [39].
23.4.1 Chest Drains/Tubes
One of the most frequent etiologies of non-resolving PNX is chest drains/tubes related, as its malposition or its obstruction. These conditions occur usually in emergency department, especially in traumatized patients in which the chest tube placement can be difficult and incomplete, determining a poor drainage of the air from the pleural space [40].
Nowadays, in fact, chest tube placement represents a cornerstone in acute chest trauma management, usually performed as a primary diagnostic procedure, often life-treating decompressing pneumothorax and the underlying collapsed lung, with a success rate ranging between 79 and 95% [41].
The British Thoracic Surgery (BTS) guidelines [42] have described the correct placement of a chest drain in a standardized technique preferably performed by surgeons or emergency physicians, after a prior physical and radiological basic examination with RX or e-FAST or, rarely, CT scan.
Although in emergency care could be difficult,, the chest drain should be inserted, in supine patient’s position, within the “safe triangle,” namely a thoracic area characterized by a low risk of complications like vascular injuries, circumscribed between the pectoralis major and the latissimus dorsi muscle, with the apex of triangle localized below the axilla and the basis as a superior line passing through the nipple; furthermore, it’s also preferable to avoid incisions below the level of the nipple, because of the high risk of intra-abdominal malposition or intra-abdominal organ injuries.
Chest tube should be inserted, after a transverse skin incision, in the 4th–6th intercostal space in the mid-axillary line [43], although, in case of anterior or apical pneumothorax, the 2nd–3rd intercostal space in the medial clavicular line is suitable for chest tube placement, always on the superior rib margin to avoid intercostal neurovascular bundle.
The chest drain insertion in emergency is usually performed percutaneously and requires the correct progression in the pleural space, so that its most peripheral hole, called “sentinel hole,” results all inside the pleural space, to avoid false position or insufficient drainage, and its attachment to the skin with a stitch, to avoid its dislodgement and its inefficiency.
After chest drain placement, it’s important to check its correct position through the execution of a chest radiograph, as well as rule out any complications, occurring between 2% and 10% [44], or iatrogenic injuries, especially in case of residual PNX, or pleural/mediastinal abnormalities.
An incorrect placement of chest tube, as false position or inadequate drainage, in fact, can occur and, sometimes, requires another chest drain to insert. A suboptimal drainage can occur, in fact, in case of kinking of the chest tube involving the intra- or extra-thoracic portion. The incomplete insertion of the chest drainage, detected with a chest radiograph performed after its placement to ensure the correct positioning, or with computed tomography scan during follow-up of a persistent air leak, is a typical etiology of non-resolving PNX, usually characterized by an abnormal seating of the tube, as outside the pleural cavity or even the chest wall; in the first case, the evacuation of air is inadequate; in the second case, instead, a backflow of atmospheric air into the pleural space can lead to suboptimal drainage and persistent PNX.
Risk factors for improper chest drainage placement, as in the chest wall outside the pleural cavity, are, especially in emergency department in traumatized patients, the presence of chest wall emphysema, or obesity or chest habitus like anomalies or deformities too, as well as the presence of scars of previous chest surgeries suggesting extensive intrathoracic adhesions and the risk of complications, especially if performed without any imaging guide.
Also a wrong seal of tissue around the chest tube insertion site could cause a persistent air leak because of the backflow of atmospheric air into the pleural space.
Other malposition or obstruction etiologies are due to intraparenchymal placement of the chest drain, usually due to traumatic pleuro-parenchymal injury or to a wrong and forced insertion, causing pleuro-parenchymal lesions too.
A more rare occurrence is the mediastinal placement of chest drainage, with high risk of severe complications as cardiovascular injuries or esophagus perforation.
Likewise, intrafissural positioning of the chest tube could lead to inadequate evacuation of air from pleural space.
The radiological features of chest drains/tubes malposition are several and detectable on RX or CT scan [29, 45].
Sometimes chest radiographs show an abnormal seating of the tube, as outside the pleural cavity or even the chest wall, leading to inadequate drainage of air and persistent PNX.
If the tube is displaced across the lateral chest wall, chest radiograph is able to identify its abnormal seating, while in case of anterior or posterior chest wall dislocation of drainage, RX is not satisfactory, requiring a CT evaluation.
In case of intraparenchymal tube placement, chest radiograph and/or chest CT identify the etiology of non-resolving PNX; the first modality imaging, in fact, although it could be unremarkable, usually reveals the presence of an opacity along the course of the intrathoracic portion of the chest tube, due to the formation of a hematoma.
CT scan, instead, especially with post-processing modality, as multiplanar reconstruction (MPR), is able to demonstrate the chest tube crossing the lung parenchyma, usually affected by contusive or lacerative injuries too.
CT, in fact, can demonstrate not only an abnormal seating of chest tube, as outside the pleural cavity or the chest wall or within the lung parenchyma or the mediastinum, or a pathological kinking of the chest drain involving the intra- or extra-thoracic portion, or an incomplete seal, often difficult to identify especially in case of subcutaneous emphysema along chest wall. In case of major subcutaneous emphysema, in fact, CT is able to identify correctly a minimum PNX too, which, instead, is very difficult with the RX scan.
CT can also detect any pleuro-parenchymal complications as lung contusions or lacerations, resulting in persistent tubular opacities along the course of the chest drain or any pathological air leak as a bronchopleural or esophageal-pleural fistula or even any cardiovascular injuries.
23.4.2 Bronchopleural Fistula (BPF)
Bronchopleural fistula (BPF) or persistent air leak (PAL) is an abnormal communication between the bronchial tree, usually a main stem, lobar, or segmental bronchus, or lung parenchyma and the pleural space [46] classified as either central or peripheral, the latter described by some authors as alveolar-pleural fistula (APF), potentially incurring in chest trauma [33].
Another, although more rare entity, is a fistula developing between the esophagus and the pleural space, named esophageal-pleural fistula.
Luckily BPF is not a common entity as it is an important cause of significantly increased morbidity, prolonged hospitalization and mortality, reaching between 18% and 50%, because of the high risk of aspiration pneumonia and acute respiratory distress syndrome, therefore requiring an early diagnosis and a proper management.
BPF can recognize several etiologies, including lung infection (as abscess, tuberculosis, etc.), traumatic injury, neoplastic invasion or iatrogenic causes (as lung biopsy or chest tube insertion or surgery) [46]. Our attention is focused on traumatic bronchopleural fistulae; the reported incidence of BPF after chest trauma, in fact, is extremely variable with a changeable time of onset distinguishable as early (1–7 days), in which the clinical condition is often very unstable needing a timely management with surgical repair and assisted ventilation, or intermediate (8–30 days), or late (more than 30 days), mainly caused by impaired healing of the bronchial leak, often associated with empyema, therefore requiring a pleural drainage too.
Major thoracic trauma, in fact, especially in presence of displaced fractured ribs, can easily cause pleuro-parenchymal injuries as pulmonary lacerations or BPF, because the elastic return of the lungs.
The presence of a bronchopleural fistula would be suspected by clinical signs and confirmed by imaging or endoscopic visualization. Nowadays, in fact, multidetector computed tomography (MDCT) is not only able to detect the fistula tract, but it can also evaluate the number, size, and location of the fistulas as well as identify any underlying traumatic diseases, sometimes avoiding further invasive diagnostic procedure such as bronchoscopies, whose role seems limited to therapy.
The radiological findings suggestive of BPF are aspecific on a plain chest radiograph, usually used as screening modality; in fact, BPF can be suspected in presence of an increased pneumothorax, or an increased opacity of the chest, usually due to pleural effusion or other pleuro-parenchymal complication, like hemo- or pyothorax.
CT scans, in fact, is the best choice in detecting BPF because it can demonstrate the airway injury and the site and the size of fistula as well as help in the surgical planning [47, 48].
CT is an effective noninvasive tool, considered superior to bronchoscopy [47], identifying, on thin slices and by image reconstruction algorithms, the defect of visceral pleura, usually recognized thanks to the presence of extraluminal air bubbles adjacent to a dehiscent bronchial stump.
CT images can demonstrate the direct communication between the bronchial tree, usually a main stem, lobar, or segmental bronchus, or lung parenchyma and the pleural space, as a dehiscent bronchial stump, distinguishing as either central or peripheral BPF.
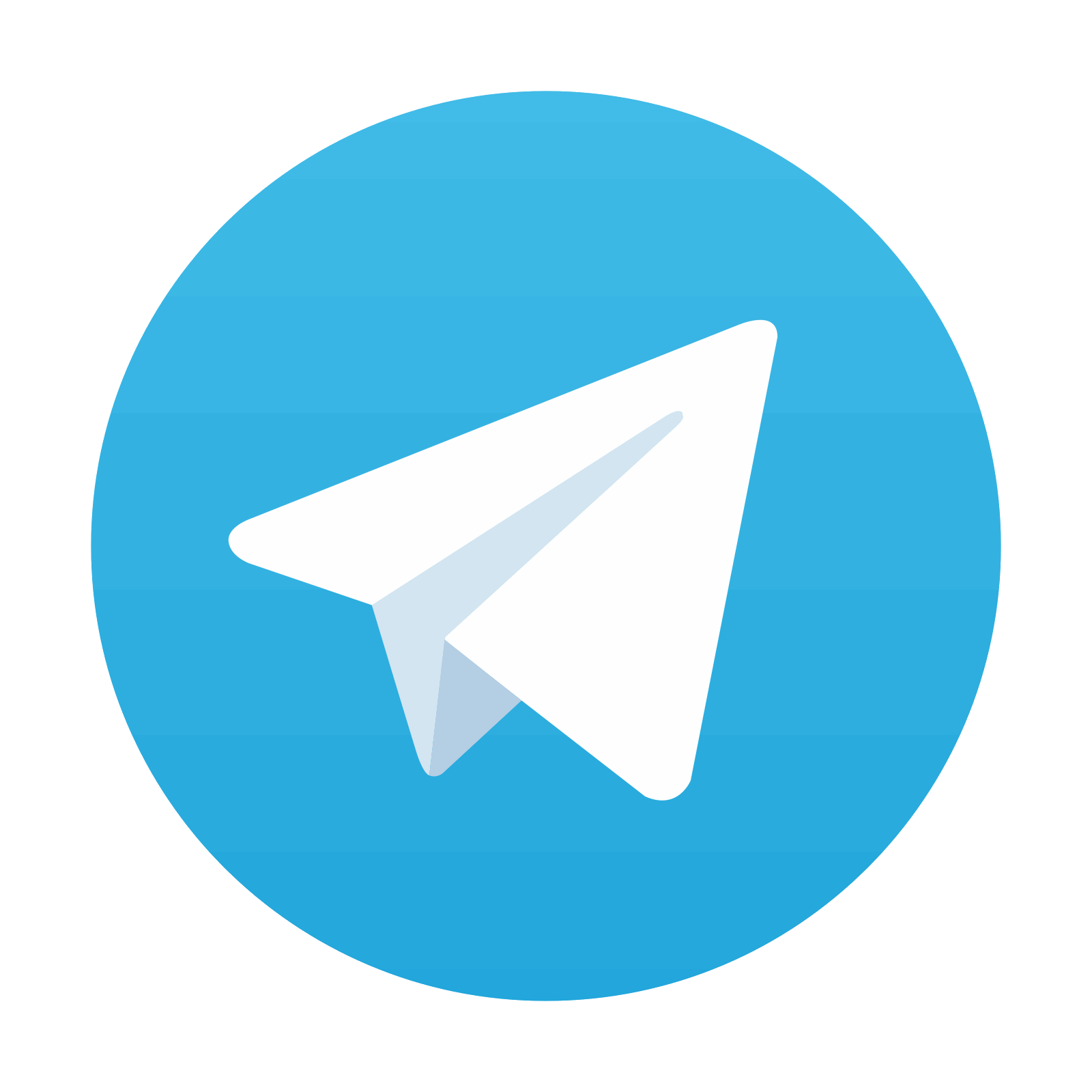
Stay updated, free articles. Join our Telegram channel

Full access? Get Clinical Tree
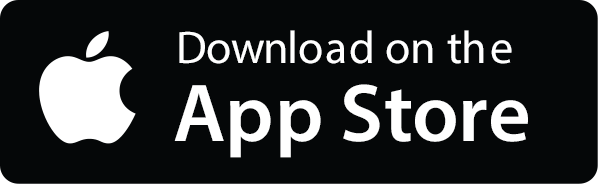
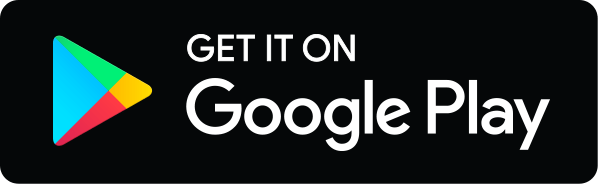