Neurovascular uncoupling (NVU) is one of the most important confounds of blood oxygen level dependent (BOLD) functional magnetic resonance imaging (fMR imaging) in the setting of focal brain lesions such as brain tumors. This article reviews the assessment of NVU related to focal brain lesions with emphasis on the use of cerebrovascular reactivity mapping measurement methods and resting state BOLD fMR imaging metrics in the detection of NVU, as well as the use of amplitude of low-frequency fluctuation metrics to mitigate the effects of NVU on clinical fMR imaging activation.
Key points
- •
Brain tumor-induced neurovascular uncoupling (NVU) may adversely affect the reliability of both task and resting state BOLD functional MRI.
- •
Cerebrovascular reactivity (CVR) mapping using breath hold techniques is an effective method for assessing NVU potential.
- •
Various resting state fMRI metrics are being investigated as potential alternatives to CVR mapping for assessment of NVU risk.
- •
Novel methods for mitigation of NVU are being investigated including the resting state fMRI metric amplitude of low frequency fluctuations (ALFF).
Introduction
In 1991, Belliveau and colleagues demonstrated that cerebral activation could be imaged using high-resolution MR imaging. Before this study, low-resolution PET studies had documented that regional cerebral blood flow increased near areas of neuronal activity in the human brain. This study obtained echo-planar images of the primary visual cortex before and after administration of a gadolinium bolus and calculated cerebral blood volumes in the stimulated versus nonstimulated states; the subtracted functional maps showed increased activity. The method used by Belliveau and colleagues is now called dynamic susceptibility contrast (DSC) MR imaging. In 1992, Ogawa and colleagues described blood oxygen level dependent (BOLD) imaging, which forms the basis for current functional MR imaging (fMR imaging). BOLD contrast was first demonstrated in a rodent model in 1990 and was first used for fMR imaging activation studies of the human visual cortex in 1992. Instead of using gadolinium contrast, BOLD exploited different magnetic properties of oxygenated and deoxygenated blood to detect changes in regional blood flow. Regional arteriolar dilatation with increased capillary filling occurs in response to brain activation, and therefore more oxygenated blood is supplied than is required for the brain’s immediate metabolic needs. In other words, the degree of regional cerebral blood flow (CBF) increase is significantly greater than the degree of concurrent increase in the cerebral metabolic rate of oxygen consumption (CMRO 2 ) during neuronal activity compared with the baseline. A decrease in the relative concentration of deoxyhemoglobin in active cortex reduces the T2/T2∗ shortening effects of deoxyhemoglobin with a resultant net increase in the BOLD signal in activated areas. In 1995, Biswal and colleagues demonstrated correlations among groups of brain regions that were known to function together even at rest (in the absence of the task performance). These findings suggested that networks of brain regions that activate or deactivate together during tasks maintain connectivity signatures that can be detected and studied even at rest. In 2001, Raichle and Snyder discovered that brain energy consumption is increased by less than 5% of its baseline energy consumption while performing a focused mental task.
Reliance on BOLD signal to represent activity in the brain has certain limitations. One major limitation is that the BOLD signal is an indirect measurement of neuronal activity. In the presence of brain tumors or other focal brain lesions, the coupling between neuronal activity and the hemodynamic changes occurring in the adjacent vasculature is often disrupted, which is called neurovascular uncoupling (NVU). , NVU is a major challenge when interpreting BOLD fMR imaging activation maps in the focal brain lesion setting, as it may lead to decreased or even absent activation in the electrically active, essential, functional (ie, eloquent) cortex.
This article reviews the challenges of brain tumor–induced NVU, which is a critical limitation of BOLD fMR imaging. NVU affects both task-based and resting state fMR imaging analysis in cases of structural brain lesions. , We focus our discussion on MR imaging approaches to detecting NVU and mitigating its effects.
The problem of neurovascular uncoupling in clinical functional MR imaging
Due to its feasibility and robustness, task-based BOLD fMR imaging has been established as the standard of care for noninvasive presurgical planning for patients with brain tumors and other focal brain lesions. In fMR imaging, the brain is repeatedly imaged while the subject is at rest and then while the subject performs an active sensory, motor, or cognitive task to localize activated brain functional regions related to the task. The resulting difference between the signals acquired during the rest period and the active period represents activation within the network of interest, which is useful in presurgical planning. Near brain tumors, the normally tight coupling between neuronal activity and the hemodynamic changes occurring in the adjacent vasculature may become disrupted due to abnormalities in the tumor vasculature or in the vessels near the tumor. This, in turn, results in abnormally decreased or absent task-related fMR imaging activation in the cortical regions near tumors, despite the patient’s ability to adequately perform tasks that would normally activate the network of interest involving these cortical regions ( Figs. 1–3 for an example of how NVU affects the language system and Figs. 4 and 5 for an example of how NVU affects the motor system). This abnormally decreased activation is called false negative activation. This differs from loss of expected activation in a similar cortex associated with a functional network in eloquent cortical destruction by tumor, where the patient’s ability to perform an fMR imaging task would be impaired in conjunction with the loss of activated BOLD signal. In the latter scenario, the absence of detectable BOLD signal would be considered a true negative rather than the result of NVU. Additionally, it is essential to differentiate unexpected lack of activation due to NVU from true cortical reorganization (neural plasticity). Clinically meaningful reorganization of language areas has been described multiple times in the neurosurgical literature. Ulmer and colleagues coined the term “pseudo-reorganization” to describe the effect of NVU on fMR imaging in brain tumors to distinguish this phenomenon form “true” reorganization. Thus, when present, NVU disguises, and may therefore result in the unintentional surgical resection of, the eloquent cortex. This could result in permanent postsurgical neurologic disability related to injury of cortical regions in the affected inadequately activated network.
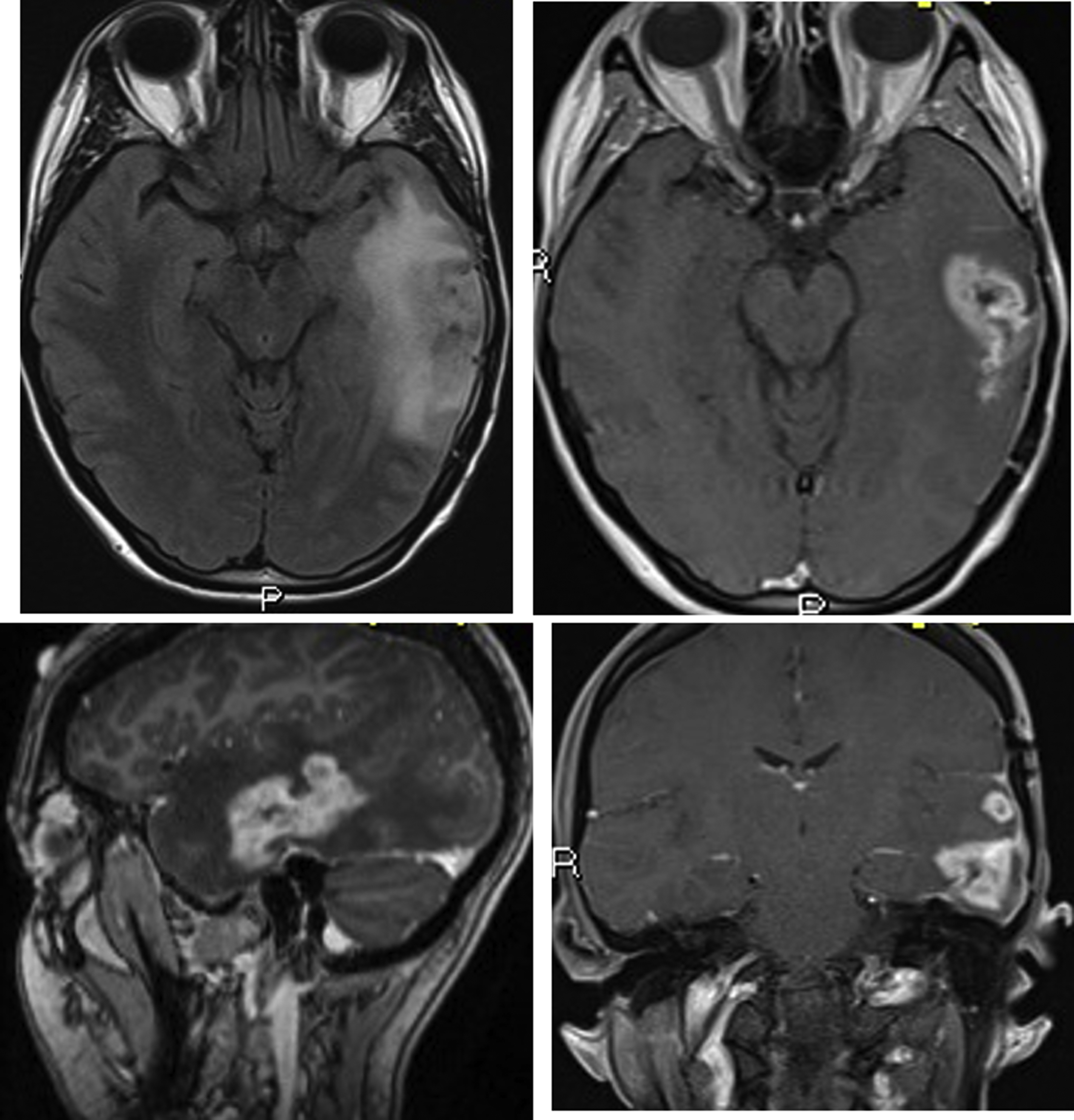
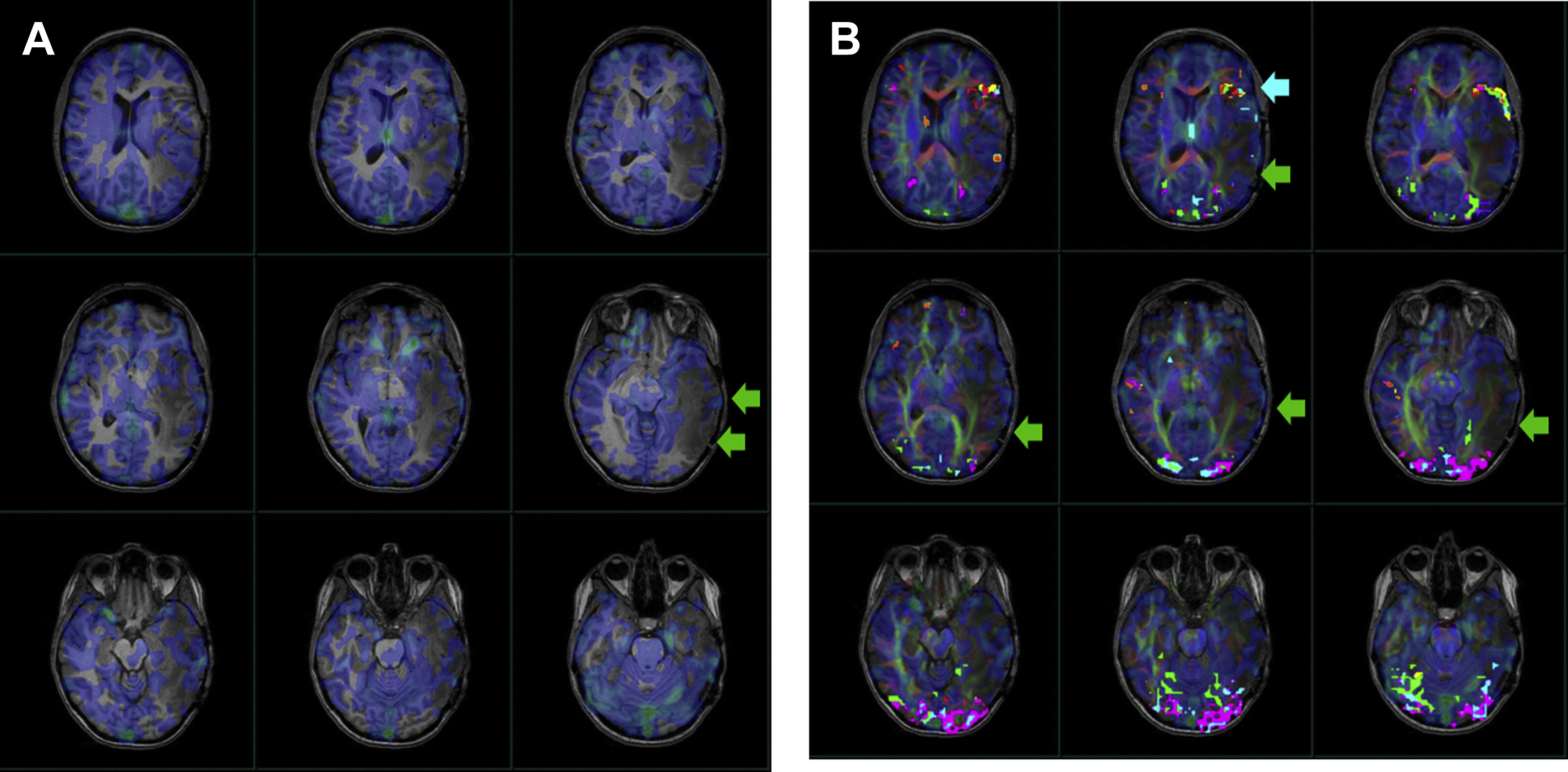
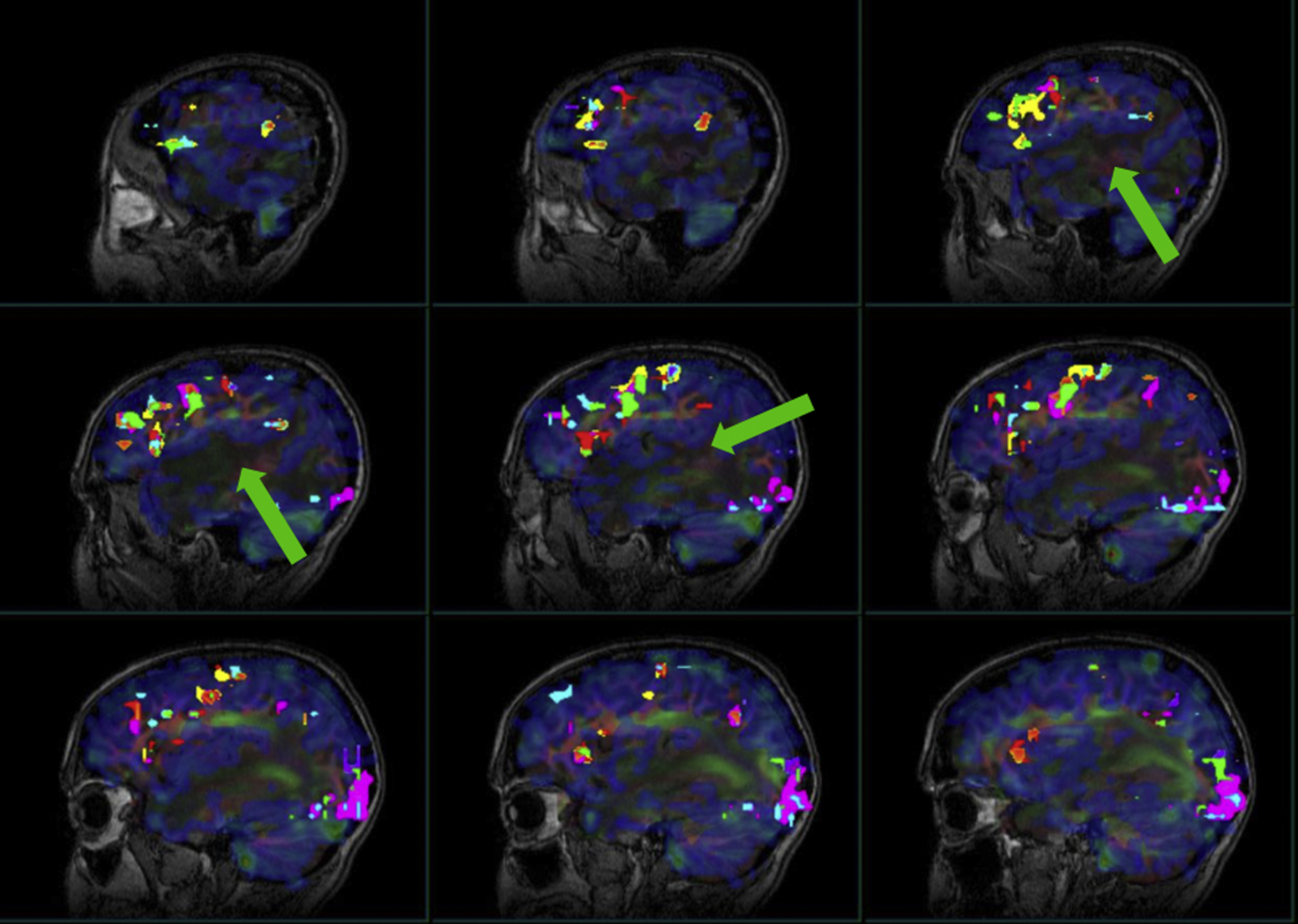
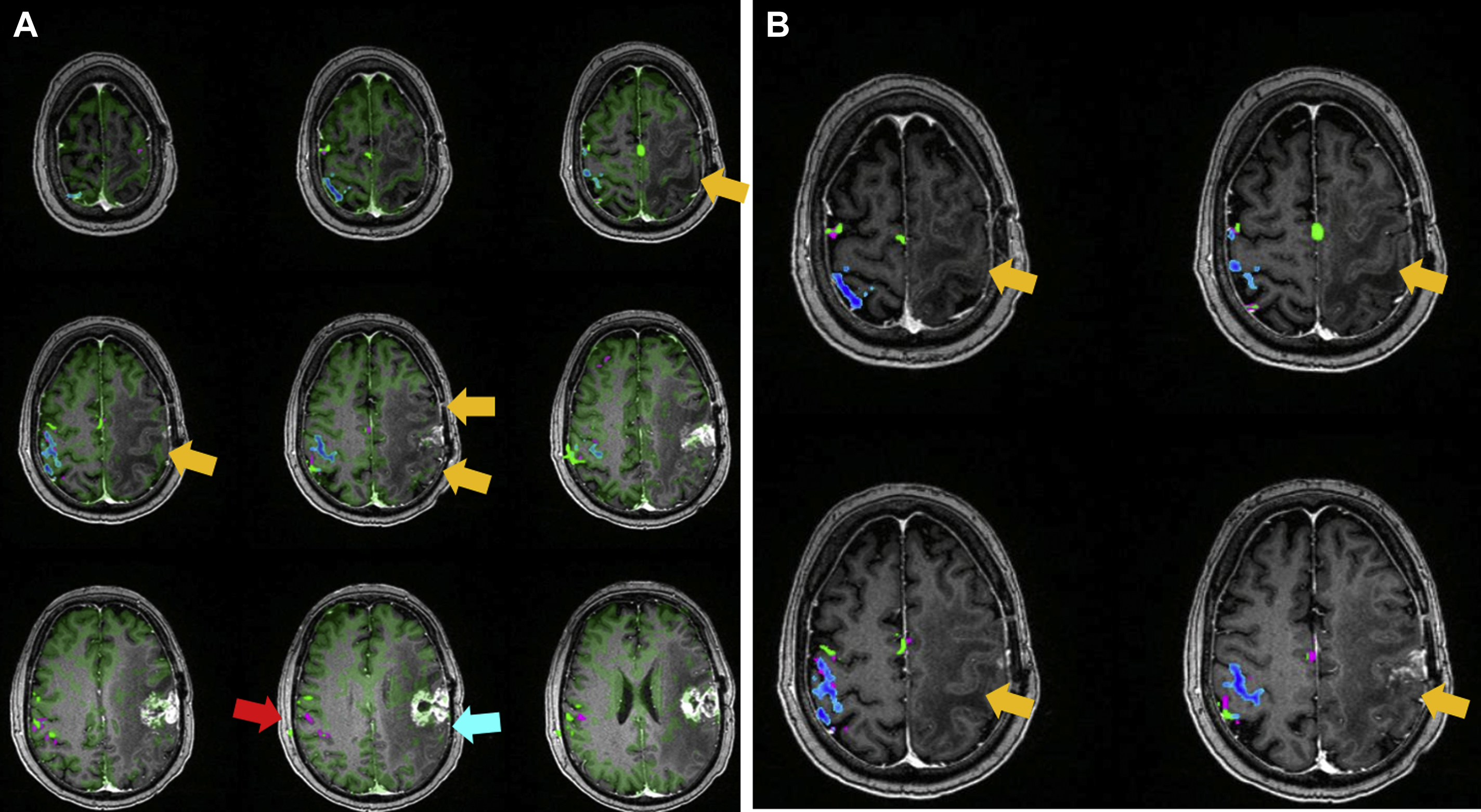
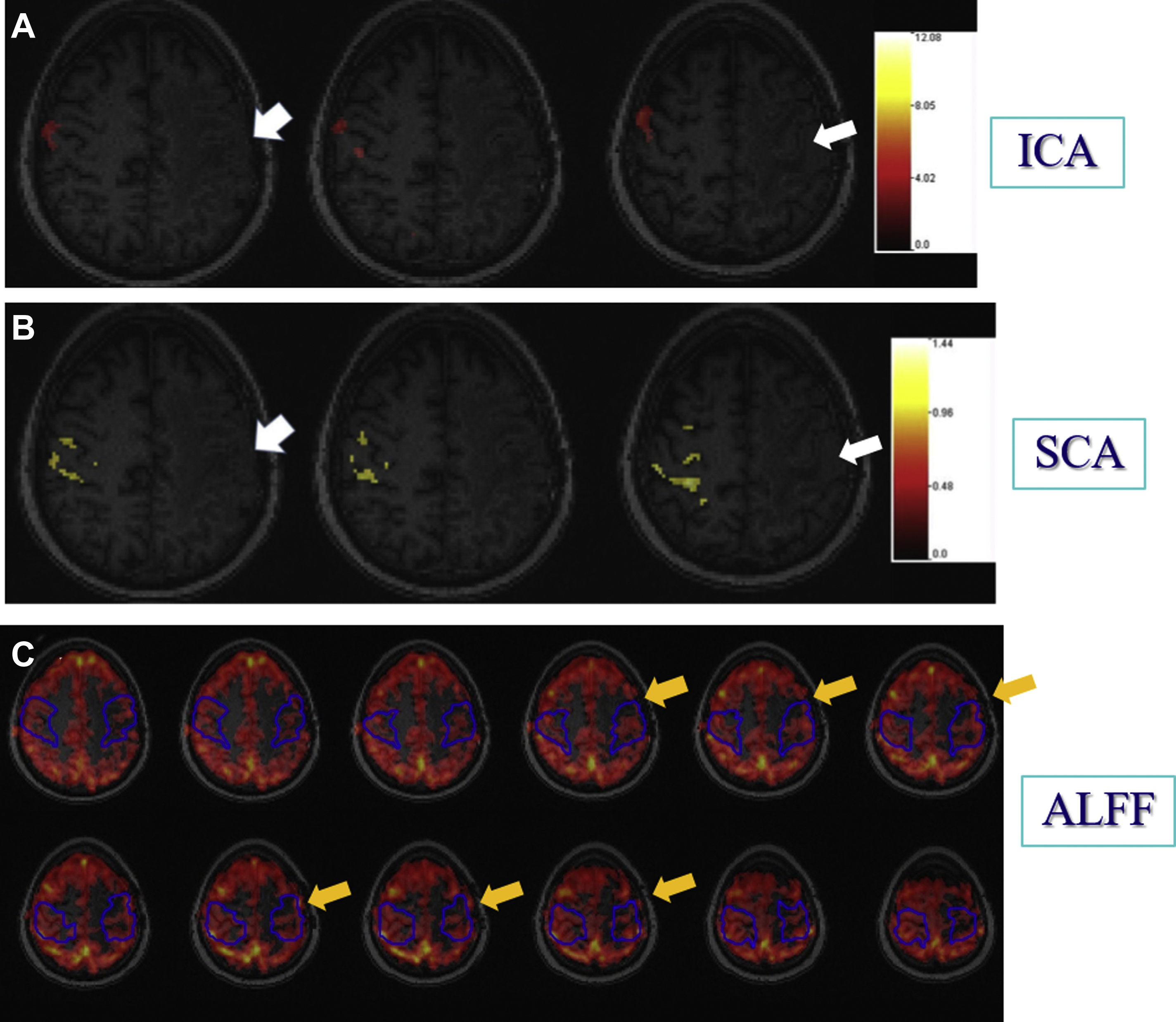
NVU is a critical limitation of clinical fMR imaging and has been widely studied in task-based fMR imaging. , NVU may be present in variable degrees, which results in variable degrees of reduction of expected ipsilesional BOLD activation in eloquent cortical regions, including complete absence of detectable activation in severe cases. NVU may result from disruption of any component of the normal neurovascular coupling cascade (NCC), which is composed of a series of biochemical steps at the cellular level, including (proximally) neurotransmitters released from activated neurons to astrocytes and (distally) chemical mediators that directly act on vascular smooth muscle of arterioles that are responsible for regulating blood flow. , Preclinical studies have suggested that tumors may induce remodeling of the extracellular matrix, changes in endothelial cells, and changes in endothelial-associated astrocytes that disrupt the NCC. Studies have suggested that the disruption of NCC in the vicinity of high-grade gliomas is primarily caused by tumor angiogenesis that results in compromise of normal cerebral autoregulatory capacity in the tumoral and peritumoral regions. , However, in cases of infiltrating low-grade gliomas, disruption of the NCC may be caused by a different etiology such as gliovascular uncoupling between astrocytic contacts and surrounding microvasculature. While the mechanisms underlying brain tumor–induced NVU are not yet completely understood, potential for NVU can be detected via demonstration of regionally impaired cerebrovascular reactivity (CVR), which is an indication of microvascular hemodynamic dysfunction.
Detection of brain tumor–induced neurovascular uncoupling using cerebrovascular reactivity mapping
CVR is the response of cerebral blood vessels to a physiologic challenge. CVR is quantified as the change in CBF per change in vasoactive stimulus. Near tumors, the vasoactivity of cerebral vessels decreases, resulting in reduced capacity for dynamic blood flow control in response to increasing neural demand. Thus, regional reduction in CVR serves as a biomarker for potential NVU.
CVR mapping can be performed using BOLD fMR imaging, which measures changes in blood oxygenation in response to vasodilatory stimulus. Davis and colleagues described the BOLD signal change in terms of CBF and CMRO 2 . Under the most CVR challenges (ie, hypercapnic conditions), changes in CMRO 2 relative to the baseline are negligible, leading to effectively linear dependence of BOLD on CBF, making BOLD suitable for CVR measurement. Previously, the vasodilating drug acetazolamide was used for CVR assessment ; however, for clinical assessment of CVR, noninvasive methods are preferred.
Hypercapnia (increased blood carbon dioxide [CO 2 ] concentration) has been widely used as a noninvasive method to elicit increased blood flow via vasodilation of cerebral arteries by endothelial smooth muscle cell relaxation. Hypercapnia can be induced via inhalation of CO 2 -enriched air or breath-holding. , , , In the former, CO 2 -enriched air is supplied through a breathing-mask to the subject during the fMR imaging scan.
CVR is calculated as the change in BOLD fMR imaging signal in response to changes in the end-tidal (ie, end expiratory) partial pressures of carbon dioxide (etCO 2 ) between the baseline and a vascular challenge condition expressed as Δ%BOLD/ΔetCO 2 . The measurement of etCO 2 is an important component for accurately assessing CVR. Complex and expensive computerized systems have been developed to implement increased etCO 2 stimuli , via dynamic end-tidal forcing , and prospective targeting. , In addition, simple respiratory circuits that administer fixed concentrations of inspired gases have been developed. Although issues have been raised regarding the performance of simple circuits using fixed inspired concentrations, , this method is less expensive and easier to use. A recent review article by Fisher offers a detailed comparison between the fixed inspired concentration methods and computer-controlled prospective targeting methods regarding issues such as complexity/simplicity, effectiveness, and cost. A detailed technical review of CVR MR imaging mapping with CO 2 challenges is provided in a recent article by Liu and colleagues.
Regardless of the type of system used, CO 2 inhalation methods require MR imaging-compatible equipment that may not be available with all scanners. Not all patients can tolerate the breathing-mask, and these patients are often excluded from CO 2 breathing studies for CVR measurements. Thus, breath-holding (BH) BOLD fMR imaging is becoming a popular alternative for CVR mapping. , , , BH CVR mapping has been demonstrated to be comparable in CVR assessment to gas inhalation techniques and is thus considered reliable. Several clinical studies have been performed using BH to assess vascular health. , , , , , , In these studies, BH CVR is measured by inducing hypercapnia using a block design paradigm under physiologic monitoring. Paced breathing between BH blocks provides a consistent baseline condition from which the BH signal response can be more accurately extracted.
Pillai and Zaca have demonstrated the advantages of BH CVR mapping over T2∗ DSC MR gadolinium perfusion imaging in the detection of NVU in brain tumors of varying grades. T2∗ DSC MR perfusion imaging has been widely used to assess tumor vascularity. Hemodynamic variables such as elevated relative cerebral blood volume and relative CBF are associated with tumor neovascularity in high-grade gliomas, , which are indirectly associated with impaired CVR due to NVU. However, in low-grade gliomas, in which regional hyperperfusion is not generally seen, static perfusion imaging is not a reliable option to assess NVU. Therefore, BH CVR mapping may be an effective tool to evaluate both high-grade and low-grade gliomas. , , , , In another study by Iranmahboob and colleagues, BH maps based on peak-to-trough were used to characterize vascular reactivity in brain tumors (ie, BOLD signal between the minimal signal seen at the end of a deep inhalation at the beginning of a BH block and maximal signal seen at the end of a BH block). The study evaluated 16 patients with various grades of gliomas and demonstrated that vascular reactivity maps in patients with brain tumor appear to be caused by a mechanism other than gadolinium enhancement. Hsu and colleagues evaluated 6 low-grade gliomas and one high-grade glioma and found that BH mapping can demonstrate differential cerebrovascular response between normal tissue and cerebral glioma. Another study by Zaca and colleagues compared BH CVR maps with clinical task fMR imaging activation maps of 12 patients with histologic diagnosis of Grade II glioma who performed multiple motor tasks and a BH task. Sensorimotor activation maps and BH CVR maps were compared in 2 automatically defined regions of interest involving the ipsilesional and contralesional perirolandic cortex, respectively. The study demonstrated that BH CVR maps were effective for assessment of NVU affecting the primary sensorimotor cortex in patients with low-grade perirolandic tumors. Pillai and Mikulis extensively described the details of BH CVR map acquisition and analysis in the clinical setting, including applications for presurgical mapping. See Figs. 2–4 for examples of how BH CVR maps can be useful in detecting NVU.
Despite its advantages, BH BOLD fMR imaging poses several challenges. BH responses vary between populations depending on the inhalation depth before BH, requiring parallel use of respiratory bellows to monitor subject compliance during the BH task. Although BH signal response depends on the subject’s capacity to comply with longer BH durations (resulting in more robust BH responses), , breath-holds following inspiration (ie, end-inspiration) with short BH durations of 20 seconds or less can generally be comfortably performed without reaching the subject’s tolerance limit. Motion artifacts tend to be increased in BH protocols. BH stimulus can also vary across time points; for example, BH following inspiration is biphasic, consisting of an initial signal dip followed by a signal increase, , which is not seen in end-expiration BHs. The BH hemodynamic response is slower than neuronal task-related BOLD signal changes, as the peak occurs several seconds after the BH period; therefore, modeling the BH response for CVR mapping is often challenging. , , Birn and colleagues modeled the BOLD fMR imaging signal changes resulting from BH and cued depth and rate changes with a respiration response function, which consists of an early overshoot followed by a later undershoot (peaking at approximately 16 seconds). Unfortunately, repeatability of BH fMR imaging may be limited due to variability in task performance, especially in the absence of end-tidal CO 2 measures.
Resting state BOLD fMR imaging signals have been explored as a useful alternative to CVR measurements in clinical applications when CO 2 -hypercapnic challenge and BH are not feasible. Kannurpatti and Biswal presented the resting state fluctuation of amplitude (RSFA), a task-free marker obtained from resting state BOLD fMR imaging (rsfMR imaging) scans, as a viable CVR biomarker that may be useful in a wider population than BH techniques. Various studies of BOLD amplitude changes during CO 2 -hypercapnic challenge or BH indicated a strong correlation between resting state BOLD fluctuations and end-tidal CO 2 fluctuations, establishing RSFA as a strong CVR correlate. , Liu and colleagues proposed another method for CVR mapping using rsfMR imaging that does not require gas inhalation. This approach exploits the natural variation in respiration and its influence on BOLD fMR imaging. The study demonstrated that global BOLD signal fluctuation in the frequency range of 0.02 to 0.04 Hz contains the most prominent contribution from natural variation in arterial CO 2 .
RsfMR imaging has attracted wide attention as a preoperative mapping tool due to its less stringent requirements for patient compliance. , , Brain tumor-related NVU limits rsfMR imaging just as it limits clinical task-based fMR imaging. , , , , For this reason, using rsfMR imaging as a reliable presurgical mapping tool necessitates further study of brain tumor-related NVU in rsfMR imaging.
Detection of neurovascular uncoupling in resting state functional MR imaging
There have been considerable efforts to clinically implement rsfMR imaging in the domain of presurgical brain mapping. , Our group at Johns Hopkins has conducted various retrospective rsfMR imaging studies related to the sensorimotor network, demonstrating the prevalence of NVU affecting this network in patients with brain tumors. , Our recent review articles have discussed in detail methods of image acquisition, monitoring, and analysis of rsfMR imaging, as well as the impact of NVU on its application to presurgical mapping in the language network. ,
Despite much exploration, there is still no consensus regarding optimal processing approaches for clinical application of rsfMR imaging. In rsfMR imaging, different areas of the brain display temporally correlated BOLD signal fluctuations in the absence of task performance, and this property can be exploited to derive intrinsic brain networks known as resting state networks (RSNs). , , The most common methods to identify RSNs are seed-based correlation analysis (SCA) and independent component analysis (ICA). In SCA, voxels within a region of interest (seed region) are selected and their average BOLD time course is correlated with that of all other voxels in the brain. Voxels showing a correlation with the seed region above a certain threshold are considered to be functionally related to the region of interest. In SCA, a priori selection of seed regions is required. In contrast, ICA is a model-free approach, which separates the BOLD time courses of all voxels into different spatial components and ensures maximum statistical independence. Because this is a purely data-driven approach, in contrast to the “seed region” approach, there is no clear link between components and specific brain functions. Other methods of rsfMR imaging analysis include cluster analysis and graph theoretic analysis.
Agarwal and colleagues evaluated 7 patients with de novo peri-rolandic tumors who underwent comprehensive clinical fMR imaging exams for presurgical mapping with conventional 3T MR imaging. On both ICA and SCA analysis, abnormally reduced synchronized BOLD signal fluctuations were noted ipsilesionally within the primary motor network (despite intact motor function) as a manifestation of motor network NVU, as the motor network is normally a non-lateralized network. Another study reported similar motor network NVU in 2 de novo brain tumor patients at ultrahigh field (7T) strength MR imaging; the higher signal-to-noise ratio was not sufficient to mitigate the effects of NVU in these patients. Mallela and colleagues examined functional connectivity of the primary and supplementary motor areas in 24 glioma patients using rsfMR imaging and correlated this with tumor characteristics and clinical information to characterize functional reorganization of RSNs. The study demonstrated that the decreased sensitivity of rsfMR imaging in high-grade gliomas was caused by NVU. In a recent study, Sun and colleagues investigated the association between tumors, tumor characteristics, and changes in resting state connectivity using SCA in 45 glioma patients and demonstrated that differences in resting state connectivity may be caused by false negatives, possibly related to NVU. In another recent study, Lemee and colleagues investigated rsfMR imaging ICA-derived RSNs versus task-based fMR imaging for language mapping with correlation with intraoperative cortical mapping during awake craniotomies in 50 adult patients. The study concluded that rsfMR imaging for presurgical language mapping allows identification of the functional brain language network with greater sensitivity than task-based fMR imaging, but with lower specificity, which may be due to NVU in the vicinity of the tumor. In another recent study, Bathla and colleagues examined the resting-state functional connectivity of the supplementary motor area (SMA) in 14 brain tumor patients and compared the SMA subdivisions (pre-SMA, SMA proper, central SMA) connectivity with the motor (precentral) gyrus and language centers. All 14 patients showed connectivity between the pre-SMA and language centers and between the SMA proper and motor gyrus; 13 patients showed connectivity between the central SMA and both the language and motor areas. The only case that did not show central SMA connectivity was caused by tumor infiltration and resultant NVU.
Another way to look at resting state connectivity is through the hypothesis that intrinsic brain activity is manifested by clusters of voxels rather than single voxels. Zang and colleagues proposed this hypothesis of regional homogeneity (ReHo) metrics. ReHo does not require a priori definition of regions of interest and can provide information about the local/regional activity of regions throughout the brain. Agarwal and colleagues demonstrated that NVU is detectable in ReHo maps and that these findings may correlate with abnormally reduced motor fMR imaging task activation. Amplitude of low-frequency fluctuations (ALFF) and fractional ALFF (fALFF) have been used as additional rsfMR imaging analysis methods, particularly for frequency domain analysis. Since rsfMR imaging focuses on spontaneous low-frequency fluctuations (<0.1 Hz) in the BOLD signal, ALFF is defined as the total power within the frequency range between 0.01 to 0.08 Hz. fALFF is defined as the ratio of the power spectrum of the low-frequency (0.01–0.08 Hz) range to that of the entire frequency range, representing the relative contribution of specific low-frequency fluctuation. Our group recently demonstrated that ALFF may be sensitive enough to detect NVU in brain tumors that affect the sensorimotor network, as ALFF may reflect both a lower frequency band CVR component and a higher frequency band neuronal component and may be used to mitigate the effects of NVU on task-based fMR imaging activation maps. Fig. 5 displays the effects of NVU on rsfMR imaging maps derived through ICA, SCA, and ALFF analysis methods in a patient with left perirolandic glioblastoma.
In addition to the CVR and resting state methods, another less commonly used method to detect NVU is functional field mapping, which was first described in research applications in the human visual system. This article will not describe this approach as it has not yet been applied widely to clinical NVU detection.
Mitigation of neurovascular uncoupling using amplitude of low-frequency fluctuations
In a preliminary study, our group enhanced the detectability of motor task activation in the ipsilesional sensorimotor cortex affected by tumor-induced NVU using ALFF from resting state fMR imaging. We observed that the average motor task activation time course in the sensorimotor cortex in contralesional (CL) and ipsilesional (IL) hemispheres of brain tumor patients were similar in periodicity; however, the relatively low amplitude of IL BOLD signal compared with CL signal reflects the effects of NVU on the IL voxels in the vicinity of the lesion. In this study, we applied an ALFF-based correction approach that utilized ratios of CL/IL ALFF values to enhance the detectability of task activation in the NVU-affected primary sensorimotor cortex.
Other approaches that have been used to mitigate the effects of NVU include a voxel-based BH CVR calibration approach and a promising recent coherence-based model that incorporates identical block design BH CVR and task-based fMR imaging activation to overcome the limitations of tumor-induced NVU. , Using this approach, Voss and colleagues studied 16 patients (9 glioblastomas, one anaplastic astrocytoma, 5 low-grade astrocytomas, and 1 metastasis) and 6 healthy controls. The study used an independent measurement of NVU quantified by the BH method to adjust the BOLD fMR imaging signal in patients with brain tumors. In the patient group, adjustment of the BOLD fMR imaging scan by incorporating the BH data resulted in the identification of clinically meaningful areas of BOLD fMR imaging activation that were not seen on routine analysis ( Fig. 6 ).
