Fig. 1.
Usual coverage of whole ventricles by short axis full cine stack (left) as oppose to the 3 short axis slices typically used in the stress exam (middle). The LV volume plot on the right demonstrates a decreased ejection during infusion of beta-blocker esmolol.
A positive test is defined by a regional wall motion abnormality during stress and is currently clinically assessed entirely qualitatively. An estimation of some constitutive parameters in a biomechanical model is a way of assessing mechanical properties of myocardium [4, 12, 13], and in [3] quantitative values directly targeting regional myocardial contractility were estimated using cine MRI as observations. Such an approach has a potential to provide a higher reproducibility of the exam, lower inter-observer variability and possibly may even allow a decrease in the dose of dobutamine as the quantitative measure may increase the sensitivity. However, in comparison to [3] – where a full short axis cine stack covering whole ventricles was used for contractility estimation – in the stress test we need to deal with the limited spatio-temporal coverage. The presented study focuses on exploring the feasibility of translating the methodology of [3] into the clinical setup of the dobutamine stress exam with respect to the challenge of limited coverage.
Due to the associated risks in using dobutamine, it would be impossible to perform trial acquisitions in a healthy volunteer or significantly prolong the patient exam to acquire additional short axis slices under the dobutamine stimulation. Therefore, to get an insight into various image sampling modes, we estimate a change of contractility in a healthy volunteer under the safe pharmacological stimulus of the beta-blocker esmolol. This allows safe acquisition of high quality image data of a full cine stack, which can be then downsampled in space or time, in accordance to the real clinical data of the dobutamine stress test. As opposed to the positively inotropic drug dobutamine, the negatively inotropic beta-blocker globally reduces the contractility. This is reflected in the decreased stroke volume (see Fig. 1, right) and we expect that the estimated contractility values should follow a similar trend.
After describing the experimental data together with image processing in Sect. 2, and the modeling and parameter estimation framework (Sect. 3), we present in Sect. 4 the results of contractility estimation at baseline and under beta-blocker stimulation by using processed cine MRI of various resolution. We discuss our results and give some perspective remarks in Sect. 5 and conclude the paper in Sect. 6.
2 Clinical Data
2.1 Data Acquistion
Cardiac MRI was performed on 1.5T Philips Achieva system. The acquisition of a healthy volunteer dataset was performed at baseline and under the infusion of beta-blocker esmolol (50–200
g/kg/min to achieve 10–20 % decrease in heart rate) and the following data were acquired in each part of the study:

Cine bSSFP sequence in retrospective ECG gating with spatio-temporal resolutionmm and 40 time frames/cardiac cycle, FOV
mm. The short axis cine stack covering contiguously whole ventricles and standard long axis views of left ventricle (2-, 3-, and 4-chamber view) were taken.
3D tagged MRI of LV in prospective ECG triggering with acquired spatial resolutionmm (for 3 orientations of tag planes) reconstructed into one resulting 3D tagged image interpolated to
mm voxel size and temporal resolution
33 ms for both baseline and esmolol scan.
2.2 Data Processing
Image processing consists first of spatial registration of all the image sequences (rigid registration using the Image Registration Toolkit IRTK1). Then, we select 3 slices from the short axis stack such that the inter-slice spacing corresponds to that one in real stress exam. Non-rigid image registration based motion tracking [11] is consequently employed to track the LV endo- and epicardial surfaces in cine MRI data. By motion tracking of various types (and combinations) of cine images, we generate three types of observations (deforming LV endo- and epicardial surfaces) which are used in sequel for the contractility estimation. These will be denoted as:
1.
full s.a. stack: the short axis cine stack covering whole ventricles with the temporal resolution downsampled to 20 time frames/cardiac cycle;
2.
3-s.a.+3-l.a.: the selected 3 slices in short axis with 3 long axis views in original temporal sampling 40 time frames/cycle (standard clinical protocol);
3.
3-slice s.a.: the selected 3 slices of the full stack (40 time frames/cycle).
Remark 1
The temporal resolution for full s.a. stack used in the parameter estimation is such that the MRI acquisition time corresponds to the standard clinical protocol 3-s.a.+3-l.a.
Additionally, we extract full tissue displacements from 3D tagged MRI by using the tracking algorithm [11] tuned to the specific spatio-temporal resolution of the tagged data. The full displacements will not be used in this study as observations, however the error between the simulated and extracted displacement will be assessed. More details about image processing of such particular type of MRI dataset can be found in [2].
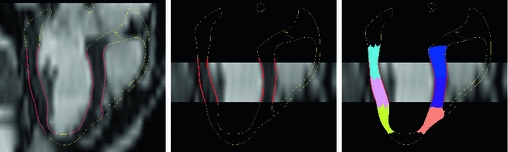
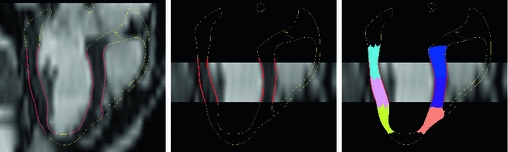
Fig. 2.
From left to right: “pseudo 4-chamber” view reconstructed from full short axis cine stack consisting of 8 mm-slices without gap with the contours of geometrical model; p4-ch view from 3-s.a. slices (inter-slice spacing 16 mm); standard AHA segments. The red parts of LV endo- and epicaridal surfaces are used in the estimation to measure the data-model discrepancy (Color figure online).
3 Modeling and Parameter Estimation Framework
The model and data-asimilation procedure used in this work are described in [3, 5], respectively, with more particular details of the estimator setup in [2]. In a nutshell, we use a continuum mechanics based model of biventricular anatomy. The model consists of an active contraction component (Bestel-Clément-Sorine model) and the passive tissue is represented by the visco-elastic material with the hyperelastic potential given by
(Ciarlet-Geymonat). The model is activated by an analytically-prescribed physiological electrical activation wave.

The data-assimilation is based on reduced-order unscented Kalman filtering [8]. The discrepancy between the model and processed image data is evaluated by means of signed distances between the corresponding LV endo- and epicardial surfaces [10]. The parts of the cardiac surfaces on which the model-data discrepancy is evaluated is given by the coverage of the heart by the observations (see red surfaces in Fig. 2). Anatomically-created AHA regions are encompassed by the full s.a. and 3-s.a.+3-l.a. observation surfaces. In the 3-slice s.a. case the mid-cavity AHA segments are nearly fully encompassed by those surfaces, however the top half of the basal segments and the apical segments are not included in the imaging data (Fig. 2, right).
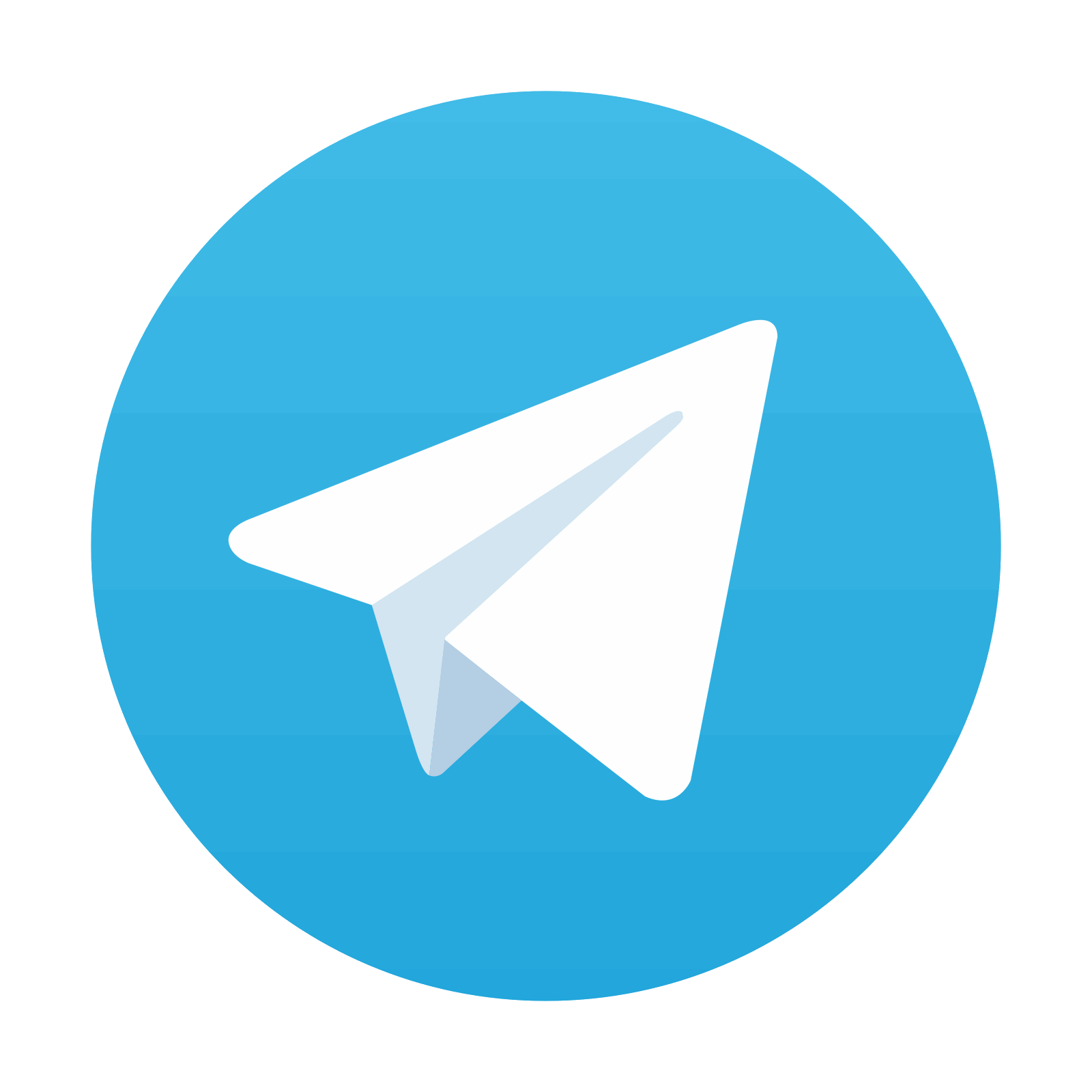
Stay updated, free articles. Join our Telegram channel

Full access? Get Clinical Tree
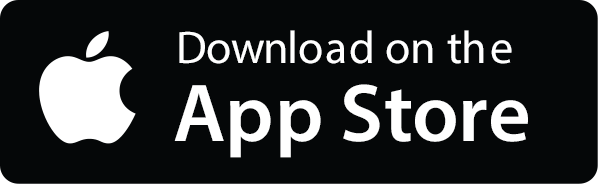
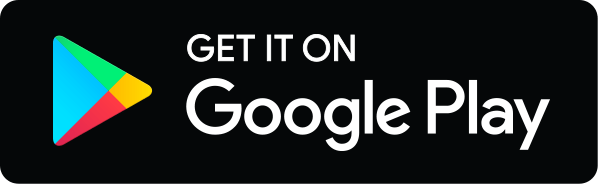