Transposition of the great arteries (TGA)
Aorta arising entirely above the right ventricle and pulmonary artery more than 50 % above the left ventricle. Complete TGA is usually D-TGA with the aorta on the right side of the pulmonary valve designated as {S, D, D} in Van Praagh classification. The S stands for atrial situs solitus (normal). The first D is for a D-looped right ventricle. The second D stands for the right-sided aortic valve. L-TGA (the aorta to the left side of the pulmonary valve) is seen in situs inversus
Transposition with pulmonary atresia
Right ventricular aorta with pulmonary atresia often termed double outlet right ventricle with pulmonary atresia
Congenitally corrected transposition of the great arteries (ccTGA)
Atrioventricular discordance and ventriculoarterial discordance (Double discordance) resulting in normal physiology circulation {S, L, L}. The first L stands for an L-looped right ventricle. The second L stands for the position of the aortic valve, which is anterior and to the left of the pulmonary valve. ccTGA is usually L-TGA in situs solitus and D-TGA in situs inversus
Anatomically corrected malposition (ACM) of the great arteries
The aortic origin lies to the left of the pulmonary trunk when there is situs solitus and concordant ventriculoarterial connection. Parallel arterial trunks, instead of the normal spiral relationship of the aorta and the pulmonary trunk
Crisscross heart or twisted atrioventricular connection
Rare anomaly as a result of rotation of ventricular mass along its long axis causing supero-inferior relationship of the ventricles with the morphological right ventricle located superiorly. The right atrioventricular axis appears orthogonal, rather than parallel, to the left atrioventricular axis. It may be seen with all kinds of atrioventricular and ventriculoarterial connections. Many have discordant ventriculoarterial connections
Subarterial infundibulum (conus)
Four possible types: subpulmonary infundibulum (normal), subaortic infundibulum (TGA or ccTGA), bilateral infundibulum (ACM of the great arteries, double outlet right ventricle), and bilaterally absent conus (double outlet left ventricle). Typically, in the absence of infundibulum, an arterial-atrioventricular valvular fibrous continuity exists. The presence of conus under a semilunar valve usually indicates that the vessel originates from the right ventricle
Ventricular loop
D-loop: refers to the normal rightward (D = dextro) loop or bend of the embryonic heart tube and indicates that the morphological RV inflow tract is on the right side of the morphological LV (right hand topology)
L-loop: refers to a leftward (L = levo) loop or bend of the embryonic cardiac tube resulting in the inflow portion of the morphological right ventricle being to the left of the morphological left ventricle (left hand topology)
Loop rule
In complex cases, it may be difficult to characterize a right or left ventricle. In general, in the presence of a right-sided aortic valve, the right ventricle is located to the right of the left ventricle (D-loop), and in the presence of a left-sided aortic valve, the right ventricle is located to the left of the left ventricle (L-loop)
Atrioventricular connection
Five types exist: concordant (normal), discordant, ambiguous, double inlet, and absent right or left connection. Concordant, discordant, and ambiguous connections are used when two ventricles are present, whereas double inlet and absent right (or left) connections are used for a univentricular heart. Concordant cardiac loops are the D-loop with situs solitus and the L-loop with situs inversus. Discordant cardiac loops are L-loop with situs solitus and D-loop with situs inversus
Ventriculoarterial connection
Double arterial trunk may have concordant connection (normal), discordant connection (TGA), double outlet right ventricle (the great vessels arise from the right ventricle), and double outlet left ventricle (the great vessels arise from the left ventricle). Single outlet can exist as a common arterial trunk, a single pulmonary trunk with atresia of the aortic trunk, or a single aortic trunk with pulmonary atresia
Position of the atrioventricular valves
It is correlated with the orientation of the ventricular loop. In a D-loop, the tricuspid valve is located to the right of the mitral valve; in an L-loop, it is to the left of the mitral valve. In general, the mitral valve is associated with the morphological left ventricle, and the tricuspid valve is associated with the morphological right ventricle
Table 10.2
Morphological characteristics of the ventricles
Right ventricle | Left ventricle | |
---|---|---|
Trabeculations | Coarse and thick at the apex | Thickened wall |
Moderator band | Starts from the ventricular septum and extend towards the apex | None |
Atrioventricular valve | Tricuspid | Mitral |
Septal valve leaflet | Apical position of the septal tricuspid leaflet | Mitral-aortic valvular fibrous continuity |
Papillary muscle | Medial papillary muscle attaching to the septum. Anterior papillary muscle continuous with the moderator band | Anterosuperior and posteroinferior papillary muscle. No septal papillary muscle |
Infundibulum | Usually exists | Usually absent |
Transposition of the Great Arteries
TGA is a common cyanotic defect seen in newborns with an incidence of about 1 per 5,000 live births [1]. It is seen in 5 % of CHD [2]. It is due to embryologic defect of conotruncal septum rotation resulting in ventriculoarterial discordance. Almost all patients with this defect who reach adulthood have had prior reparative cardiac surgery, although some with a large ventricular septal defect (VSD) and subpulmonic stenosis can survive with Eisenmenger physiology [3]. Without treatment, 95 % of these patients die in the first year, mainly from hypoxemia. Patients who have undergone atrial-level repair for TGA comprise an important group of adults with CHD. It is estimated there to be approximately 9,000 patients in the United States currently alive with an atrial-level repair [4, 5].
Morphology
In typical TGA, the atria and ventricles are in the usual position (Fig. 10.1). It is also known as D-TGA; the “D-” refers to the dextroposition of the bulboventricular loop [i.e., the position of the right ventricle (RV), which is on the right side]. In this anomaly the aorta and associated coronary arteries arise anteriorly from the RV and the pulmonary artery arises posteriorly from the left ventricle (LV). The aorta also tends to be on the right and anterior, and the great arteries are parallel rather than crossing as they do in the normal heart (56 %) [6] (Figs. 10.2 and 10.3). The second most common arrangement is with the aorta just anterior to the pulmonary artery (26 %); the rarest fetal variant is side-by-side arrangement. In Massoudy et al.’s [7] study of 200 cadaveric hearts with TGA, five categories for the relationships of the great arterial trunks were found. The aorta could be anterior and to the right of the pulmonary trunk (75 %), directly anterior (10 %), anterior and to the left (3 %), side by side with the aorta to the right (10 %), or posterior and to the right (2 %). The latter arrangement is also called normal relations, albeit with discordant ventriculoarterial connections [8] (Fig. 10.4). In regard to the orientation of the sinuses of the aorta relative to those of the pulmonary trunk, in most cases, the commissures between the valvular leaflets face each other at the sinotubular junctions. Commissural mismatch is uncommon (13 %) and may have surgical implications during arterial switch [7] (Fig. 10.5).
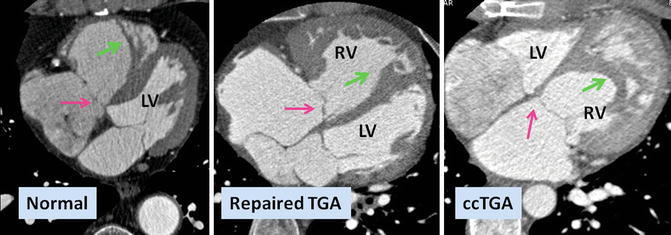
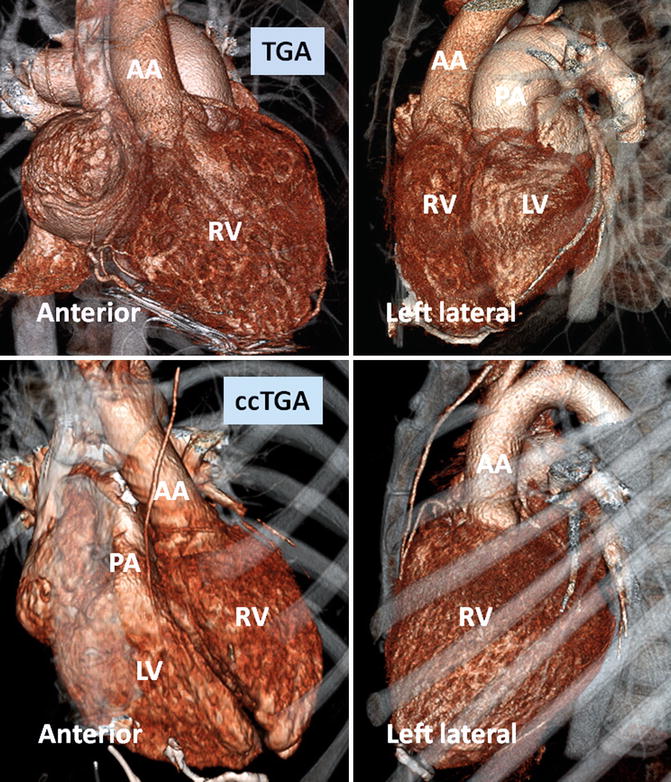
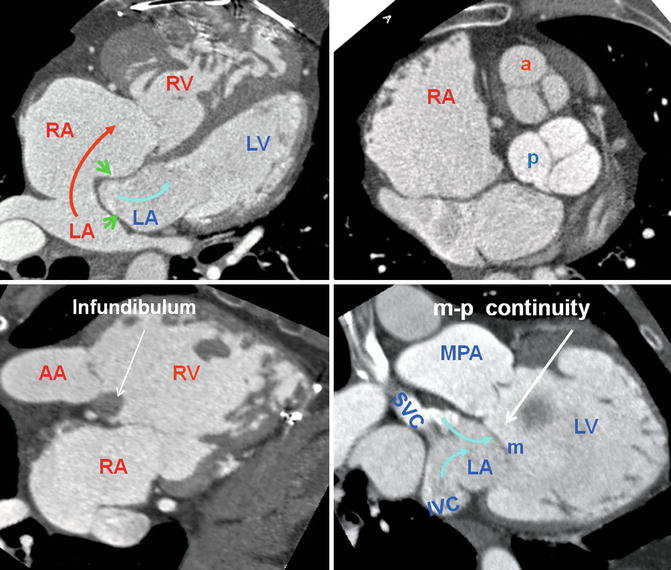
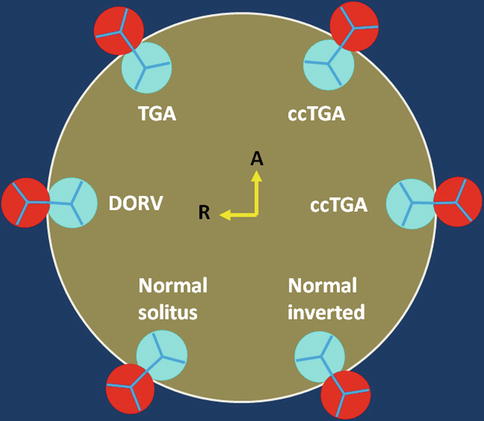
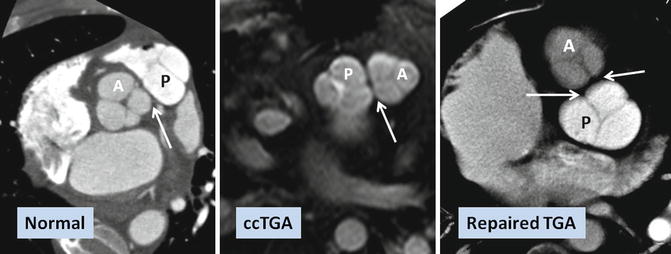
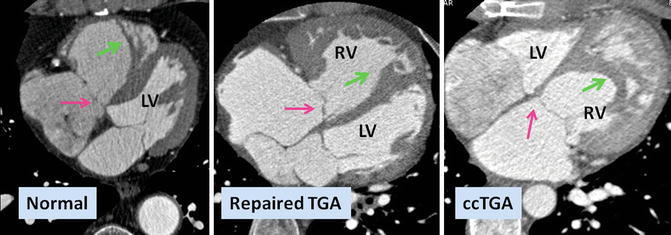
Fig. 10.1
Axial views of the heart demonstrate the orientation of heart in thorax, ventricular arrangement and the atrioventricular valves position in normal, repaired transposition of great arteries (TGA), and congenitally corrected TGA (ccTGA). The cardiac crux has a mirror image appearance in ccTGA. The moderator band of morphological right ventricle (RV) is shown by green arrows. In the RV the septal leaflet of tricuspid valve (pink arrows) is attached to the septum 1 cm closer to the apex compared to the mitral valve (pink arrows). Further apical migration of the tricuspid valve is common in ccTGA. LV morphological left ventricle
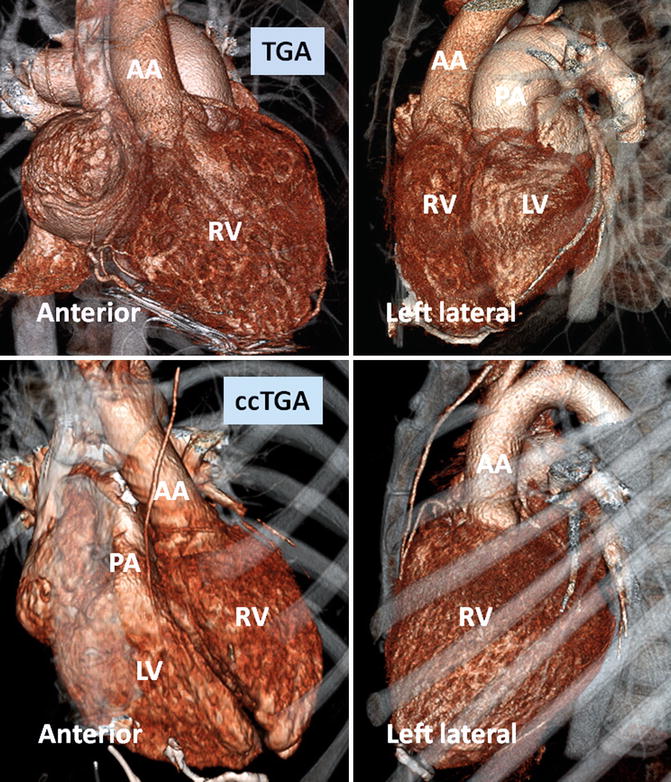
Fig. 10.2
Anterior and left lateral intrathoracic orientation of the heart in TGA and ccTGA. Note the anterior/posterior arrangement of the ventricles in TGA and their side-by-side position in ccTGA. In ccTGA, the morphological right ventricle (RV) forms the left lateral cardiac margin (levoposition). In TGA, the ascending aorta (AA) is located on the right anterior aspect of the main pulmonary artery (PA). LV morphological left ventricle
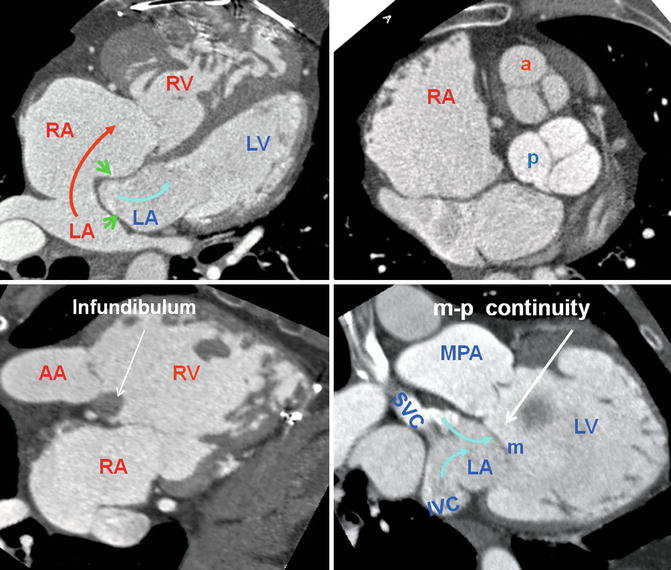
Fig. 10.3
Heart morphology in transposition of great arteries (TGA) status post atrial switch. Intra-atrial baffle (green arrowhead) diverts oxygenated blood of the left atrium (LA) into the right atrium (RA) (red arrow). Cardiac crux is normal. The morphological right ventricle (RV) works as systemic ventricle and appears hypertrophied. In TGA the aortic valve (a) is located anterior and to the left of the pulmonary valve (p) in most cases. The pulmonary valve is sitting between the two atria. The RV infundibulum is not well developed and there is fibrous continuity between the mitral (m) and the pulmonary (p) valves. AA ascending aorta, IVC inferior vena cava, MPA main pulmonary artery, SVC superior vena cava. LV left ventricle, blue arrows showing caval (non systemic) flow
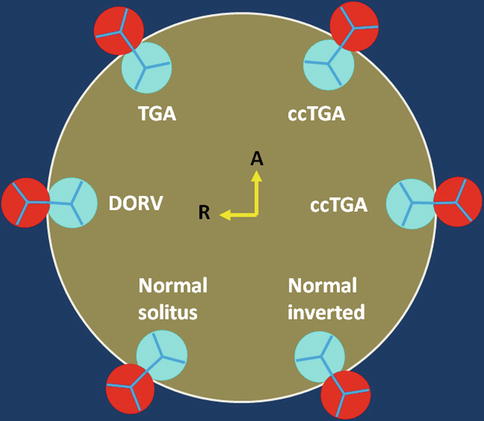
Fig. 10.4
Schematic representation of the aortic (red) and pulmonary (blue) valve relationships. Variability between the presented alignments is common
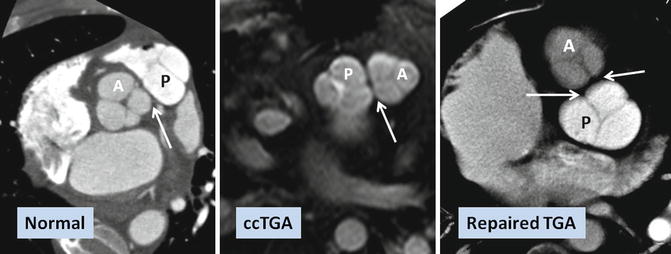
Fig. 10.5
Commissural malalignment of the aortic (A) and pulmonary (P) valves in TGA (shown by arrows). In normal heart and ccTGA, the intervalvular commissures are well aligned. Although uncommon, mismatch between aortic and pulmonary valves can occur in TGA patient which may have surgical implication for arterial switch operation
About two-thirds of TGA patients have simple transposition and may only have a small patent ductus arteriosus (PDA) and/or a patent foramen ovale (PFO) (Fig. 10.6). The presence of other cardiac lesions constitutes complex transposition. These include large PDA, atrial septal defect (ASD), VSD (22 %) [9], or coarctation of the aorta (4 %) [5, 10]. Rightward (more common) or leftward displacement of the outlet septum frequently results in subaortic or subpulmonary stenosis, respectively, and is frequently associated with a perimembranous VSD [11, 12]. Subaortic stenosis due to either deviation of the outlet septum or prominence of the ventriculo-infundibular fold and septoparietal trabeculations can occur.
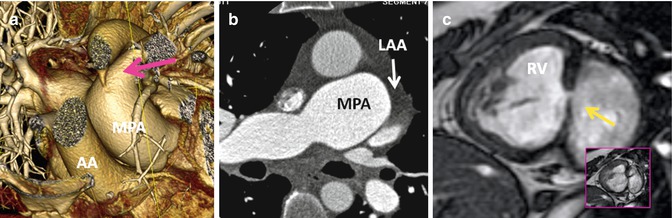
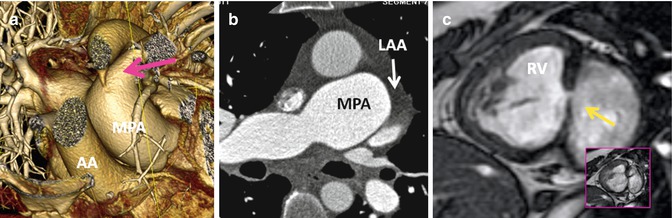
Fig. 10.6
Associated findings in repaired TGA. (a) Small patent ductus arteriosus (pink arrow). AA ascending aorta, MPA main pulmonary artery, RV right ventricle, inset box is systolic image showing the shunt as a dark line extending into the left ventricle. (b) Enlarged pulmonary arteries due to pulmonary hypertension. Note incomplete opacification of the left atrial appendage (LAA) suggestive of atrial fibrillation. (c) Recurrent small ventricular septal defect (arrow)
Postsurgical TGA
If there is no atrial defect or VSD, the cyanotic neonate with TGA will not survive the first few days of life without some early intervention. As the first intervention, TGA babies may be treated with percutaneous Rashkind atrial balloon septostomy procedure to create a more sizable atrial septal defect to allow mixing of systemic and pulmonary venous return [13]. This procedure can improve their oxygenation until definitive surgery is performed. Early attempts at surgical repair of TGA were aimed at physiologic correction, because techniques for anatomic repair were not available. The surgical techniques of an atrial switch for patients presenting with TGA were described by Senning in 1959 [14] and by Mustard in 1964 [15]. By the 1980s, late complications of these repairs including sinus node dysfunction, atrial flutter, systemic ventricular dysfunction, baffle leak, and stenosis of the superior vena cava (SVC) baffle had become well recognized [16]. These complications, along with advances in cardiac surgery, led to the adoption of the neonatal arterial switch operation (ASO), which is now standard therapy for TGA [17–19].
Intra-atrial Baffle
In the Senning repair, two longitudinal incisions are made through lateral walls for the right and left atria. A flap made from the atrial septum is sewn to the free wall of the left atrium (LA) between the left atrial appendage and pulmonary veins, isolating the pulmonary veins behind it. If the flap is not large enough, it may obstruct left pulmonary veins at their orifices. The posterior and anterior edges of the incision through the lateral wall of the right atrium are then sutured to the free margin of the atrial septum and the left atrial incision, respectively, to reroute the deoxygenated caval blood to the mitral valve and the oxygenated pulmonary venous blood to the tricuspid valve. In Mustard technique which is less complicated, the baffle is created by intra-atrial sewing of a trouser-shaped patch of pericardium or synthetic material to the atrial walls (Fig. 10.7). In Mustard, the atrial septum must be excised as much as possible. At the end, the right atrium may be enlarged by a pericardial or a synthetic patch (Fig. 10.8). In both techniques the anatomic LV continues to act as the pulmonary pump and the anatomic RV acts as the systemic pump (Figs. 10.9 and 10.10). The Senning and Mustard repairs still have a small role in the treatment of TGA in patients with fixed subpulmonary obstruction and as part of the “double-switch” type of repair used in the treatment of congenitally corrected transposition of great arteries (ccTGA).
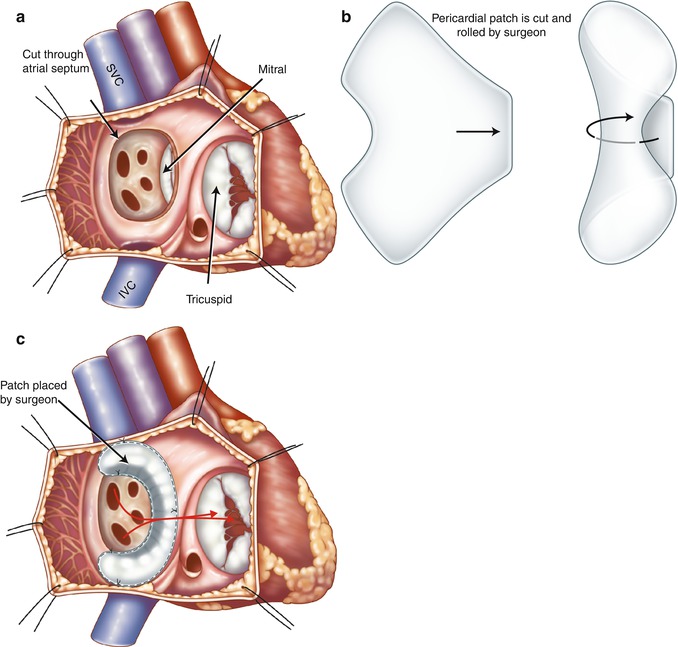
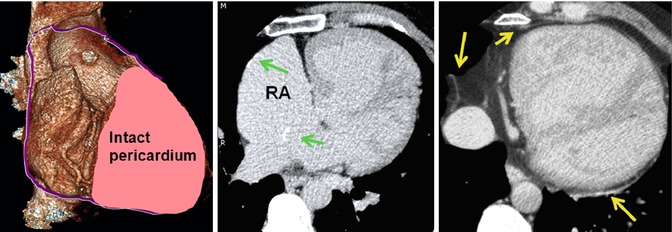
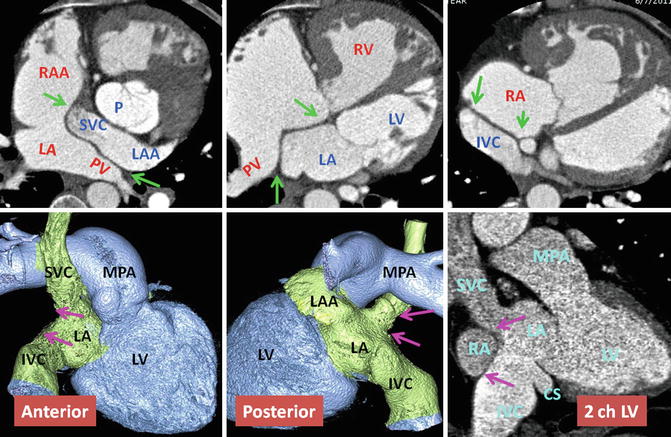
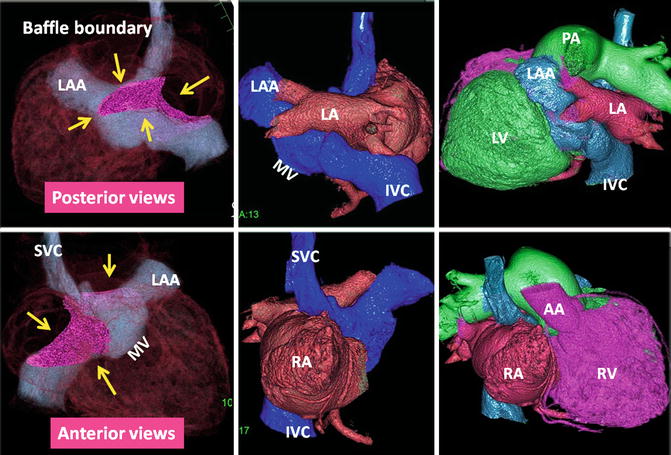
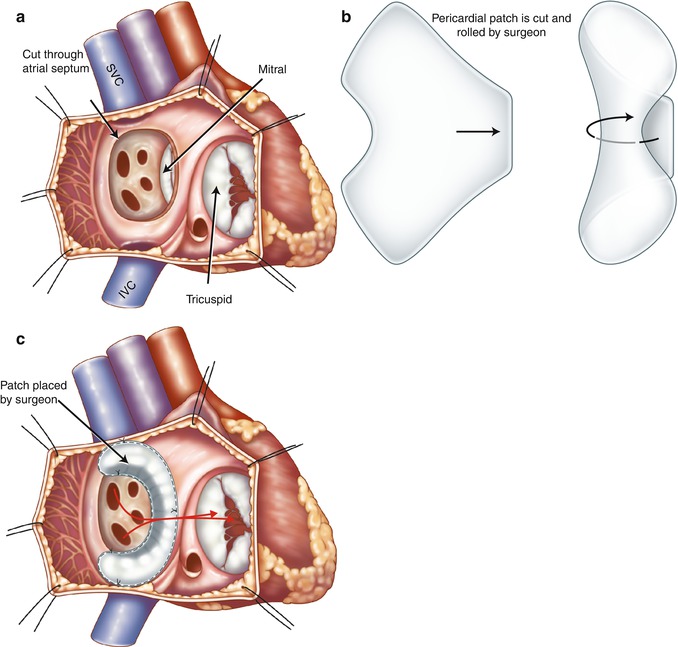
Fig. 10.7
Illustration of Mustard procedure. (a) The interatrial septum is removed through a right atrial incision. (b) A trouser-shaped patch of pericardium is created. (c) A baffle is formed by suturing the patch to the right atrial wall, the ostia of the inferior vena cava (IVC) and superior vena cava (SVC), and the anterior margin of atrial septal window to reroute the deoxygenated caval blood to the mitral valve and the oxygenated pulmonary venous blood (red arrows) to the tricuspid valve. Note in this example the coronary sinus ostium is not covered by the patch. Some surgeons may leave the coronary sinus to drain to the systemic venous atrium to lower possible suturing damage to the conduction system
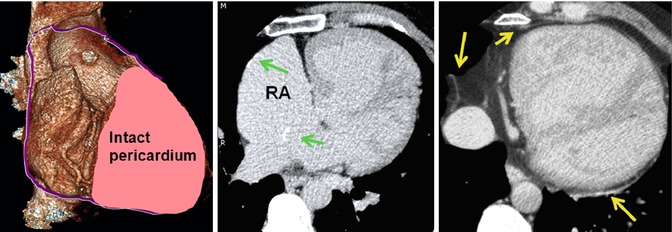
Fig. 10.8
Pericardiectomy to prepare a pericardial patch for Mustard surgery. A large part of the right pericardium is usually removed. It is mostly used for baffle construction. Part of it can be used to repair or enlarge the right atrial wall. The left anterior and posterior pericardium remain intact (yellow arrows). Note calcified foci at the surgical sites of the right atrial wall and interatrial baffle (green arrows)
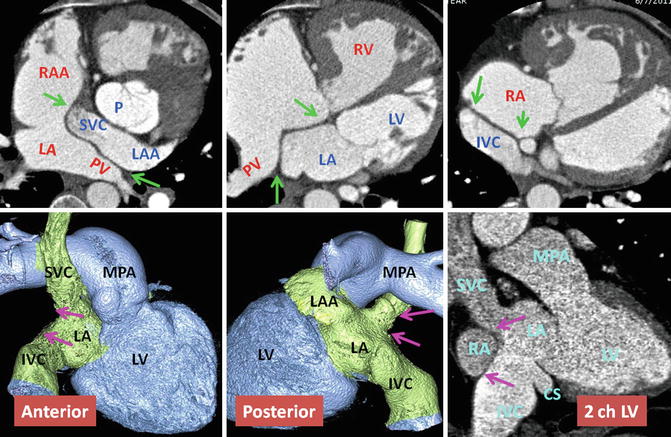
Fig. 10.9
Upper row: Intra-atrial baffle boundaries in transposition of great arteries (TGA) after atrial switch operation. The pericardial baffle covers the ostia of the SVC and IVC and from there extends into the left atrium (LA), dividing atrium into inner (venous) and outer (systemic) chambers. The baffle then attaches to the lateral wall of the LA between the pulmonary veins (PV) and left atrial appendage (LAA) ostia. Points of suturing of the patch, marked by green arrows, include superior (SVC ostium), inferior (IVC), midline (remainder of atrial septum at cardiac crux), and lateral (free wall of the left atrium). Lower row: nonsystemic circulation of TGA. The intra-atrial baffle location is shown by pink arrows. The structures participating in nonsystemic circulation include the SVC, IVC, LAA, anterior wall of LA, left atrial vestibule, mitral ring, morphological LV, and pulmonary arteries. Color-coded images show no connection to the coronary sinus. The coronary sinus (CS) may be covered by the baffle and becomes part of the nonsystemic circulation. This is shown in sagittal two-chamber (2ch) CT in a different patient. IVC inferior vena cava, LV left ventricle, MPA main pulmonary artery, P pulmonary artery, RA right atrium, RAA right atrial appendage, RV right ventricle, SVC superior vena cava
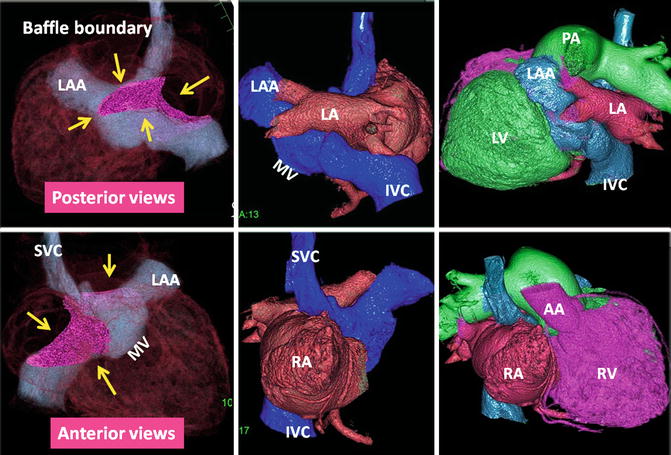
Fig. 10.10
The status of atrial baffle after atrial switch for transposition of great arteries. The boundaries of intra-atrial baffle are shown by yellow arrows on volume-rendered CT images. Homogenous enhancement of both atria was achieved using a double injection CT technique. As a result of modest attenuation values throughout the heart chambers, high-quality three-dimensional images can be reconstructed. After atrial switch non-oxygenated blood of the IVC and SVC is diverted into the vestibule of left atrium (LA), the left ventricle (LV), and finally the main pulmonary artery (MPA). The oxygenated blood of the venous part of LA enters the right atrium (RA), the right ventricle (RV), and the ascending aorta (AA). IVC inferior vena cava, LAA left atrial appendage, MV mitral valve location, SVC superior vena cava
Arterial Switch: Jatene Procedure
With improvements in critical care and technical skill, the Jatene arterial switch has become the optimal surgery of TGA and can be performed in early weeks of life [17, 18]. To restore the ventriculoarterial relationships, the coronary arteries are excised from the aorta, followed by transection and switch of the ascending aorta and the pulmonary artery. To reduce the risk of coronary artery kinking before their reimplantation, the pulmonary artery is relocated anterior to the aorta (the Lecompte maneuver) (Fig. 10.11). The pulmonary artery may be augmented by using pericardial patch to prevent the possibility of stenosis. Patients with anomalous coronary arteries are not good candidates for the Jatene procedure. In cases in which TGA is associated with a VSD and subpulmonary stenosis, the Rastelli procedure is the preferred method of repair. This procedure consists of a patch closure of the VSD to the aortic valve and placement of a conduit between the RV and the pulmonary arteries [20]. After the Rastelli operation, assessment of possible stenosis or incompetence of the RV-to-PA conduit, LV outflow tract flow, biventricular function, and possible residual shunt is needed. This is probably best achieved by cardiac MRI. After the Mustard or Senning procedure, adults with TGA may develop right ventricular failure and require consideration of new therapies. A 2-stage pulmonary artery banding and ASO may be performed as an alternative to heart transplantation (Fig. 10.12).
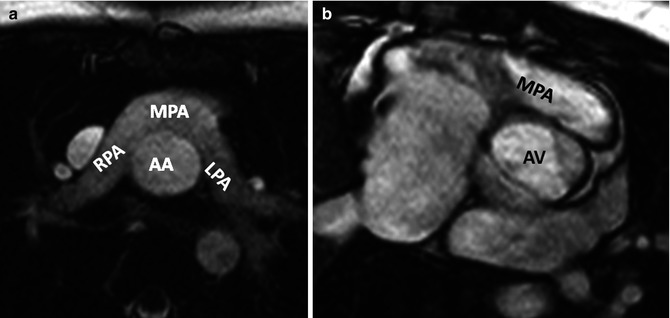
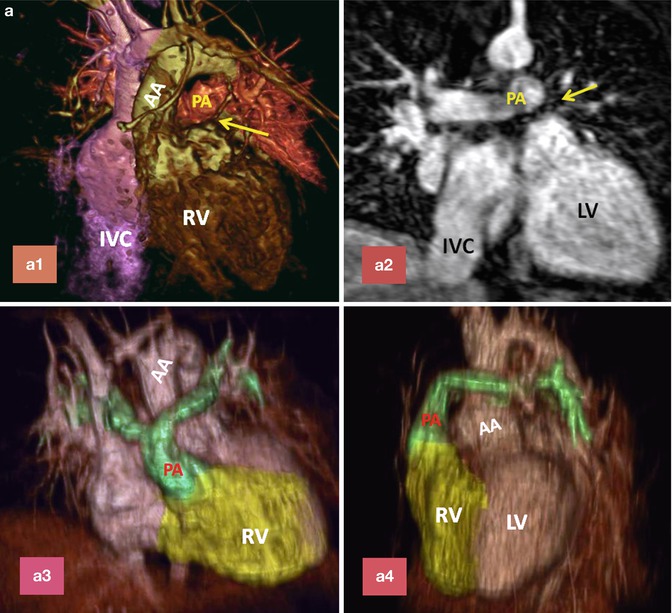
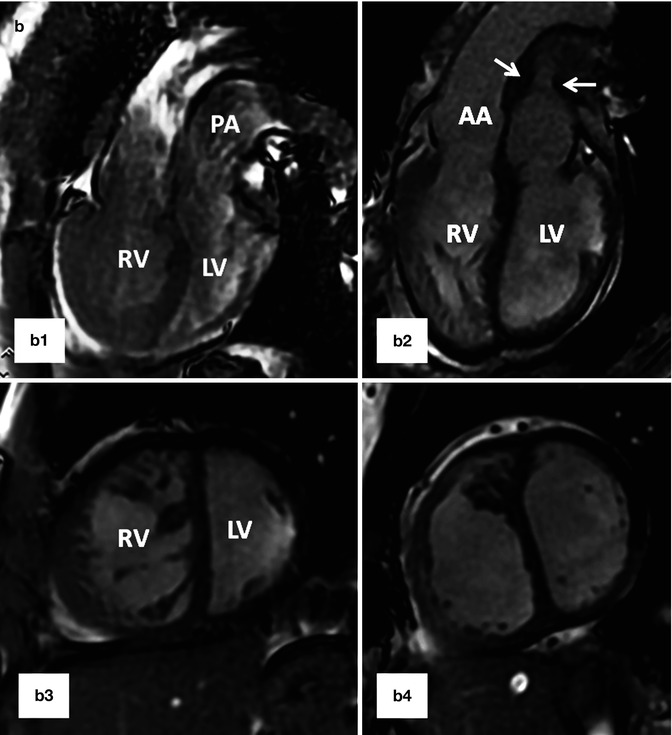
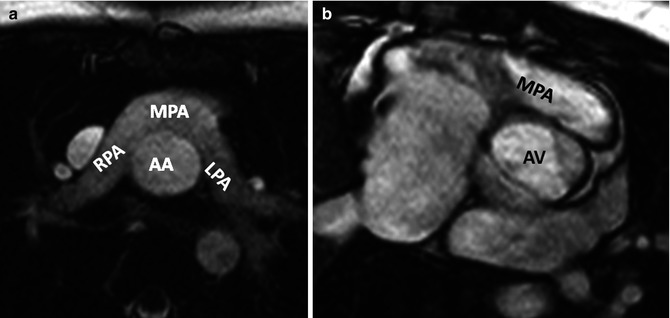
Fig. 10.11
Pulmonary artery appearance after Jatene arterial switch procedure. (a) Axial MRI and (b) Short-axis MRI show retrosternal course of main pulmonary artery (MPA). Dilated aortic annulus (neoaorta) is shown in (b). AA ascending aorta, AV aortic valve, LPA left pulmonary artery, RPA right pulmonary artery
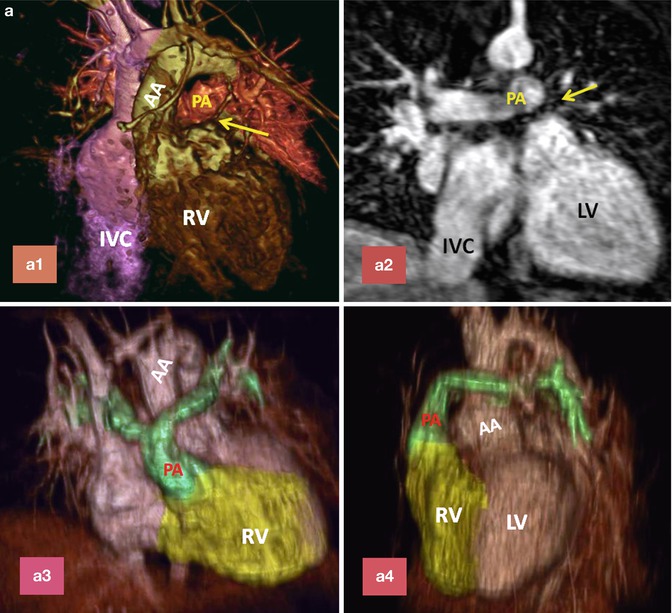
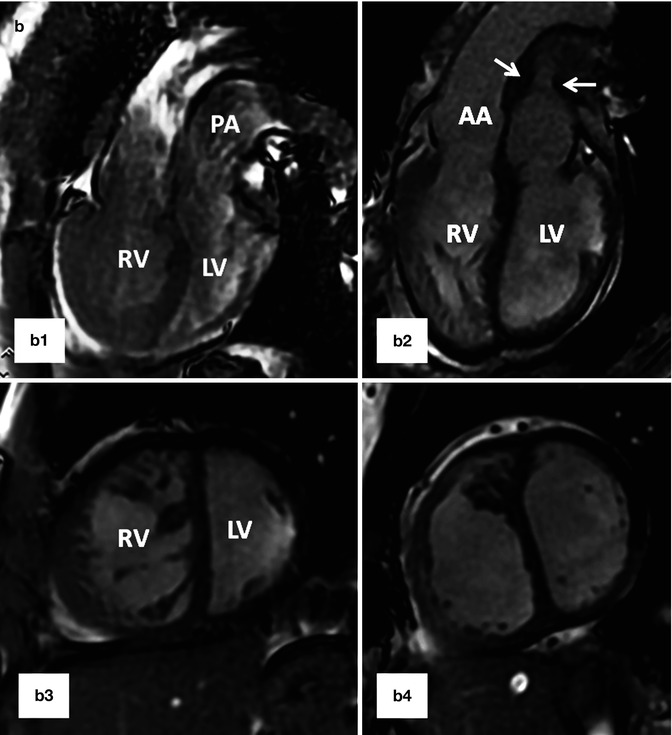
Fig. 10.12
(a) A 26-year-old patient with post Mustard TGA, complete correction was performed after 2-stage pulmonary artery banding and arterial switch operation. (a1) and (a2) are MR angiograms after pulmonary banding. The pulmonary band creates a dark signal on MR angiogram (yellow arrow). Note anterior position of the ascending aorta (AA). (a3) and (a4) are MR angiogram after arterial switch performed 1 year after pulmonary banding. Note anterior position of the pulmonary artery (PA). (b) A 25-year-old female status post Mustard TGA before (b1 and b3) and 6 months after (b2 and b4) pulmonary artery (PA) banding showing improved left ventricular (LV) function as shown by ventricular dilatation and decreased septal straightening. The right ventricle (RV) load is diminished. Stenosis of the main pulmonary artery at level of banding is shown (arrows). IVC inferior vena cava
Postsurgical Complications
Complications After Atrial Switch
Baffle-related complications are a frequent cause of reoperation and account for 30 % of reoperations in patients with history of Mustard operation and 5 % of Senning operation [21–24]. The reason for a higher incidence of baffle-related complications in the Mustard group may be seen in the use of synthetic material or folding of excessive baffle material. Early complications include baffle obstruction or leak and vena cava obstruction [21, 24]. Late surgical complications include leak and pulmonary and/or systemic venous pathway stenosis [24].
Baffle Stenosis. The systemic venous pathway obstruction occurs more often after Mustard repair (15 %) than after the Senning procedure (1.4 %) [22], but there is a trend towards greater obstruction of the pulmonary venous pathway in those who have undergone the Senning procedure (7.6 % versus 3.8 %) [25]. Obstruction of the pulmonary venous pathway can manifest as pulmonary venous hypertension and pulmonary edema. The site of obstruction is typically between the inferior vena cava (IVC) baffle limb and the lateral atrial wall. Less commonly, the left pulmonary veins can become obstructed, causing left-sided edema. Both the superior and inferior caval baffle limbs can develop stenosis, but the SVC limb is more frequently affected (Fig. 10.13). With MR and CT the site of stenosis can be easily identified. The degree of stenosis can also be quantified using MR velocity encoding. Severe stenosis is defined as narrowing on cine images with a peak velocity of over 1.5 m/s and a damped and continuous flow curve. Mild stenosis is defined when the flow is pulsatile and peak velocity in early diastole not exceeding 1 m/s [26]. In chronic SVC stenosis, patients are often asymptomatic, as they may develop collateral flow into the chest wall and paravertebral veins with retrograde drainage into the IVC [27]. Patients may also develop persistent pleural effusions or chylothorax. Chronic stenosis of the IVC is not well tolerated owing to subsequent hepatic congestion and ascites. Venous stenosis can often be treated with transcatheter-delivered stents [28] (see Fig. 25.11). Pulmonary venous pathway obstruction after Mustard is often identified using phase-contrast imaging that demonstrates increased venous flow velocity or loss of normal phasic flow patterns. However, in the setting of severe obstructions, pulmonary arterial blood flow is redistributed towards the unaffected side resulting in very low venous flow on the affected side masking flow acceleration. In this situation, measuring pulmonary artery flow can be helpful. In case of left pulmonary vein obstruction, arterial flow will be redistributed with more flow shifting to the right side. Reversed diastolic flow in the ipsilateral artery (in the absence of pulmonary regurgitation) and continuous flow in the contralateral pulmonary artery may be seen in severe pulmonary vein obstruction [29].
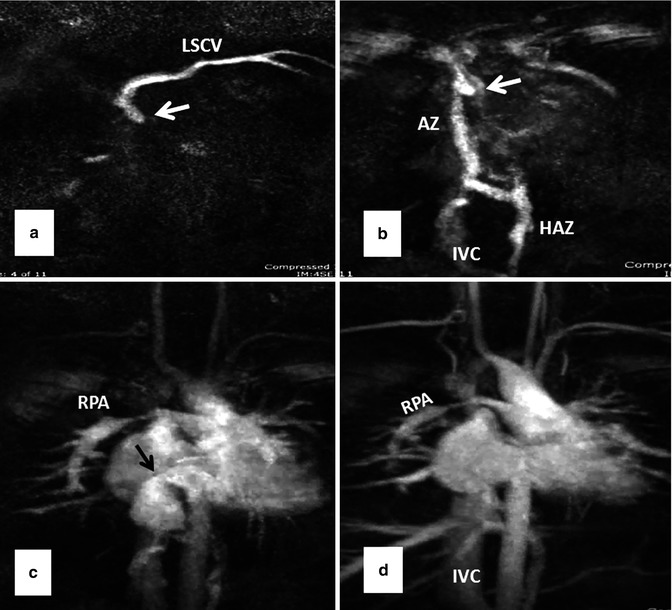
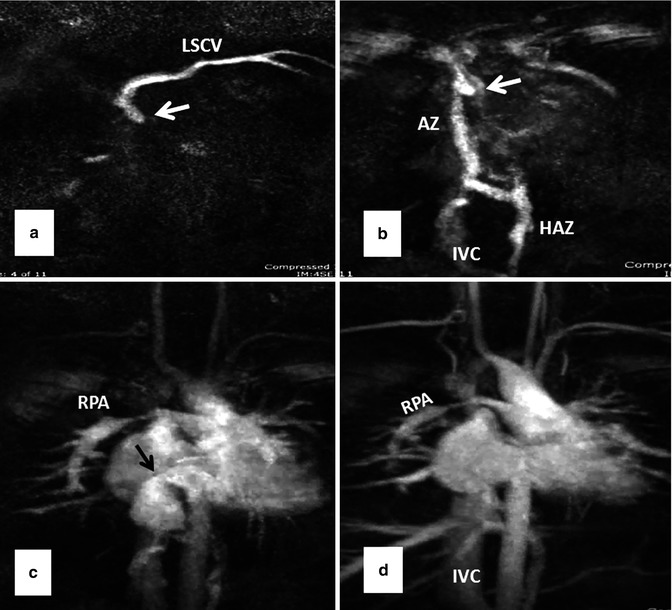
Fig. 10.13
Serial MR angiography images (a–d) show severe superior caval baffle stenosis (white arrows) causing shift of contrast injected from left subclavian vein (LSCV) into the azygos (AZ), hemiazygos (HAZ), and inferior vena cava (IVC) (a and b). Mild stenosis of the inferior caval baffle is also shown (black arrow in c). Note stenosis of the right pulmonary artery (RPA in c and d) and occlusion of the proximal right subclavian artery as sequelae of a Blalock-Taussig shunt (Courtesy of Tiffanie R. Johnson MD, Section of Pediatric Cardiology, Riley Hospital for Children, Indiana University)
Baffle Leaks. Leaks in the intra-atrial baffle are more common with Mustard than with Senning repairs. Small defects in the wall of baffle are common and usually hemodynamically insignificant (Fig. 10.14). However, small defects can increase the risk of paradoxical embolus and cerebrovascular event, especially in patients with history of tachyarrhythmias or in the presence of an endocardial pacemaker (see Fig. 30.13, extracardiac complications). In order to decrease this risk, all TGA patients who require pacemaker implantation typically undergo a thorough pre-procedural imaging evaluation to assess for the presence of a baffle leak. Complex anatomy of the interatrial septum after atrial switch can make detailed analysis of the baffle difficult (Fig. 10.10). Although transesophageal echocardiography can provide most information required to evaluate these cases, in many cases it is not conclusive and additional diagnostic tests are necessary for final decision making. CT is known for its ability to show detailed anatomic structures. It is fast and easy to interpret (Figs. 10.9 and 10.10). However, special injection technique is required to show both sides of the baffle without much streak artifacts (Fig. 10.10). CT has also the advantage of showing additional anatomic problems including a patent ductus arteriosus or anomalous venous return (Fig. 10.6). When the LV is enlarged, it is important to rule out a significant pathway leak with predominant systemic-to-nonsystemic shunt (equivalent of RV enlargement in concordant heart chambers with an ASD). Baffle leaks can sometimes be treated with placement of occluder devices [30] (see Fig. 30.13, extracardiac complications). The combination of a baffle leak proximal to a baffle stenosis will exacerbate nonsystemic-to-systemic shunting and leads to desaturation, especially with exercise.
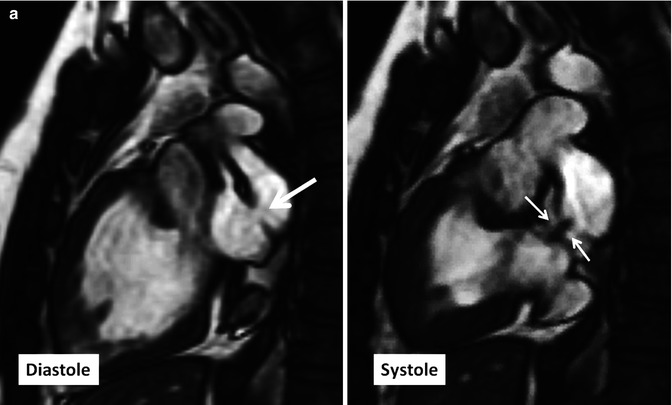
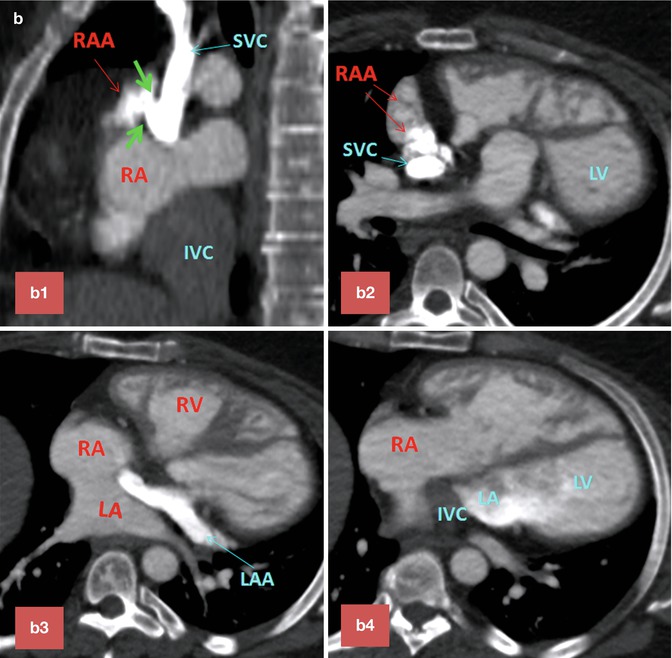
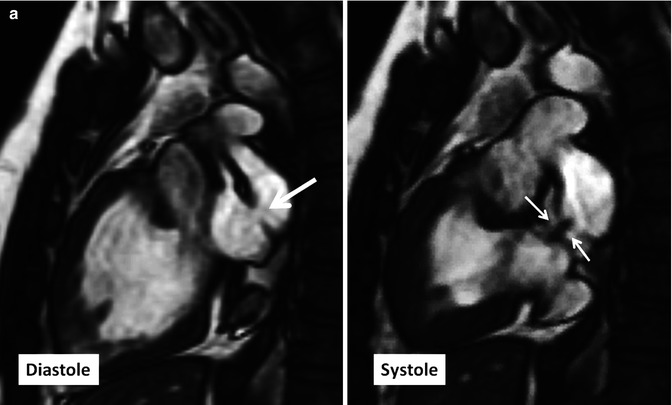
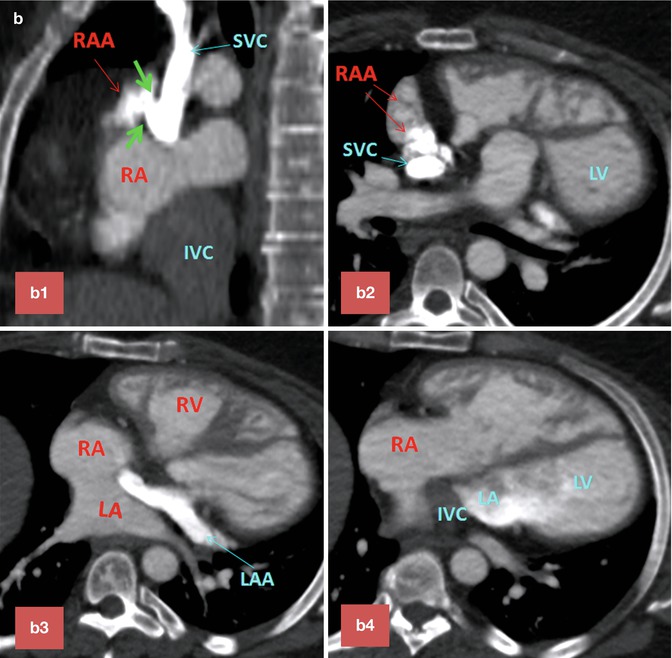
Fig. 10.14
(a) Two-chamber long-axis cine MR images show an atrial baffle small defect of atrial baffle show a small defect in diastole (large arrow) and a shunt (small arrows) though it during ventricular systole. (b) Baffle leak shown by CT angiography. (b1) is sagittal cut through the caval veins and (b2–b4) are axial images at three levels of baffle. Contrast arrival through the superior limb of baffle shows a defect (between green arrows) at superior caval limb between the superior vena cava (SVC) and right atrial appendage causing small right to left shunt. IVC inferior vena cava, LA left atrium, RA right atrium, LV left ventricle, RV right ventricle
Other Complications. Direct operative trauma to the sinus node or damage to its blood supply and scar lines across the atrium have been suggested as the principal causes of sinus node dysfunction in both types of atrial switch operations [7, 31]. Atrial flutter is not uncommon after Mustard repair [22, 31]. Long-term neurologic complications include stroke and seizures, often related to thromboembolic events (see Fig. 30.14, extracardiac complications). Right (systemic) ventricular failure with secondary tricuspid valve insufficiency is common [23, 25]. These patients can develop progressive cardiomegaly and may ultimately require end-stage heart failure management, including cardiac transplant [25]. Less common complications include pulmonary arterial hypertension (6 %), residual VSD, and dynamic subpulmonary stenosis [32–34] (Fig. 10.6). A mild degree of subpulmonary stenosis is common in patients with TGA following atrial-level repair [32]. More-severe subpulmonary stenosis after baffle reconstruction is rare and can be treated with a left ventricle-to-pulmonary artery valved conduit [33].
Complications After Arterial Switch
Compared to atrial switch, the rate of dysrhythmias is less [35]. Complications that may be seen at imaging after an arterial switch procedure include main pulmonary artery and branch pulmonary artery obstruction, aortic root dilatation with aortic regurgitation, right ventricle outflow tract (RVOT) obstruction, and coronary artery stenosis [36, 37]. The most frequent types of stenosis are discrete pulmonary narrowing at the anastomosis and the right pulmonary artery segmental stenosis because they are draped across the aorta behind the sternum. Dilatation of the neoaortic root is also common, but hemodynamically significant neoaortic valve regurgitation is uncommon [38]. Increased dimensions of the aortic annulus lead to loss of coaptation of the aortic valve leaflets and varying degrees of central aortic regurgitation (Fig. 10.11a). Reduced elasticity of the proximal aorta, reduced LV systolic function, and increased LV dimensions as measured with MRI are frequently observed these patients [39]. It has been reported that there is a substantial risk of early and late coronary artery occlusion or stenosis in patients undergoing an arterial switch procedure. Most of these patients are asymptomatic, at least initially [40]. Because the RVOT and the pulmonary arteries are positioned immediately behind the sternum after the arterial switch procedure, transthoracic echocardiography is a poor method for evaluating obstructive lesions in these vessels. The anatomic and functional significance of such obstructions can be more accurately assessed with MR imaging by using a combination of techniques including cine imaging, gadolinium-enhanced MR angiography, and velocity-encoded MRI [41]. In patients in whom the presence of coronary ischemia is suspected, coronary CT angiography is the imaging modality of choice. However, coronary MR angiography is also useful for the noninvasive investigation of proximal segments of the coronary arteries [42].
Conversion to Arterial Switch
If the systemic RV is failing after an intra-atrial repair, morphologically left ventricular reconditioning and an arterial switch operation is an alternative to cardiac transplantation in selected patients [43]. The first step in such an approach is a surgically placed pulmonary artery band (average 1 year). This is necessary because the LV rapidly becomes deconditioned when exposed to the low-resistance pulmonary circuit, losing its ability to generate systemic pressure. Pulmonary artery banding provides adequate LV training if done at an early age, but results in older patients may not be satisfactory [44]. After banding, MRI is used to evaluate ejection fraction, ventricular mass, and tricuspid valve function. With increasing morphological LV pressure after banding, a shift in the interventricular septum and reduction of the tricuspid regurgitation and right ventricle dysfunction are expected. Later the atrial baffle can be taken down, the pulmonary artery band removed, and the arterial switch procedure performed [44] (Fig. 10.12).
Anatomic Variability of Coronary Vessels
Anomalous origin of the coronary artery is not uncommon in TGA patients and may cause problem during arterial switch operation [7]. In adult patients with the history of atrial switch, these anomalies can be seen in diagnostic tests including MRI and CT and could be of surgical significance at the time of anatomic conversion surgeries (Fig. 10.15). In Massoudy et al.’s study [7], the frequency of the potentially relevant surgical features in a large series of autopsied hearts was reported. In 60 % of cases, the right coronary artery (RCA) arose from the right sinus and the left anterior descending (LAD) and left circumflex arteries from the left sinus. In 10 % the LAD originated from the right sinus, taking a retropulmonary course in 50 %, interarterial in 10 %, and preaortic in 40 %. High takeoff above or at the level of sinotubular junction was seen in 16 %. Juxtacommissural origin of coronary arteries, intramural or tangential takeoff, and commissural mismatch of arterial trunks are relatively rare but can lead to accidental injury when the aorta is transected during arterial switch [45] (Fig. 10.5). Furthermore, other features, such as retropulmonary course of the left coronary artery or origin of both the RCA and the LAD from the right sinus, have been shown to be important [46]. These abnormal features can now be diagnosed with accuracy using cardiac CT angiography. With MRI diagnostic-quality images of the coronary ostium and proximal coronary artery, course can be obtained [42]. Anomalies of the coronary veins are rare. However, in patients with intra-atrial baffle after Senning of Mustard operations, part of the coronary sinus tributaries may be divided between the right and left atria (Fig. 10.15). In patients with systemic right ventricle, the coronary veins draining into the right atrium are enlarged.
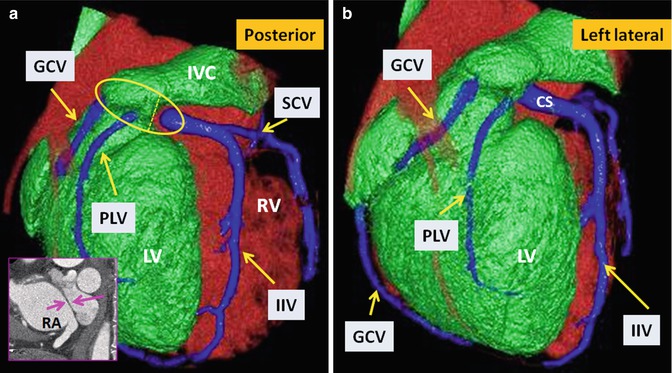
Get Clinical Tree app for offline access
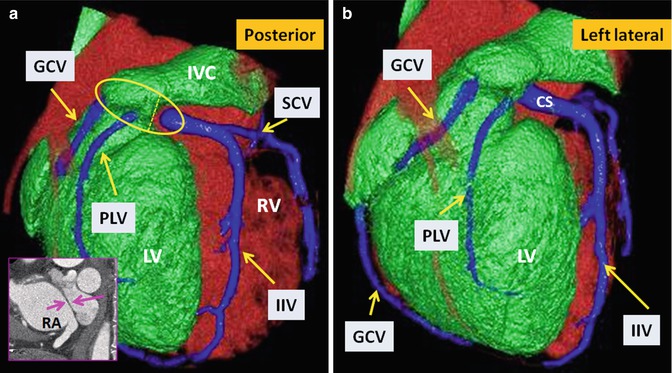
Fig. 10.15
TGA status post Mustard operation. Posterior (a) and left lateral (b) views of the heart show anatomic distribution of the coronary veins. The coronary sinus (within the yellow circle) appears divided into two parts by the intra-atrial baffle (pink arrows
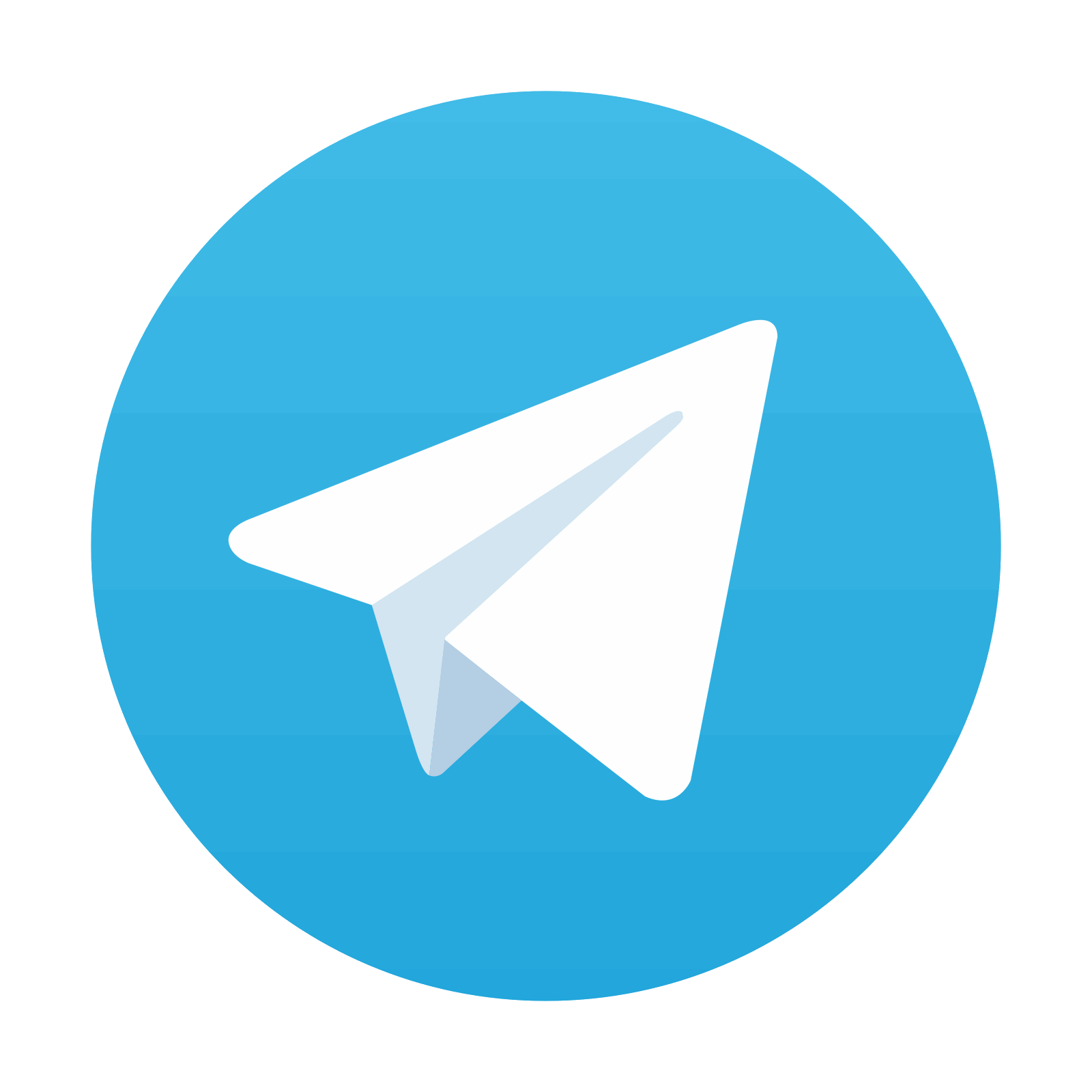
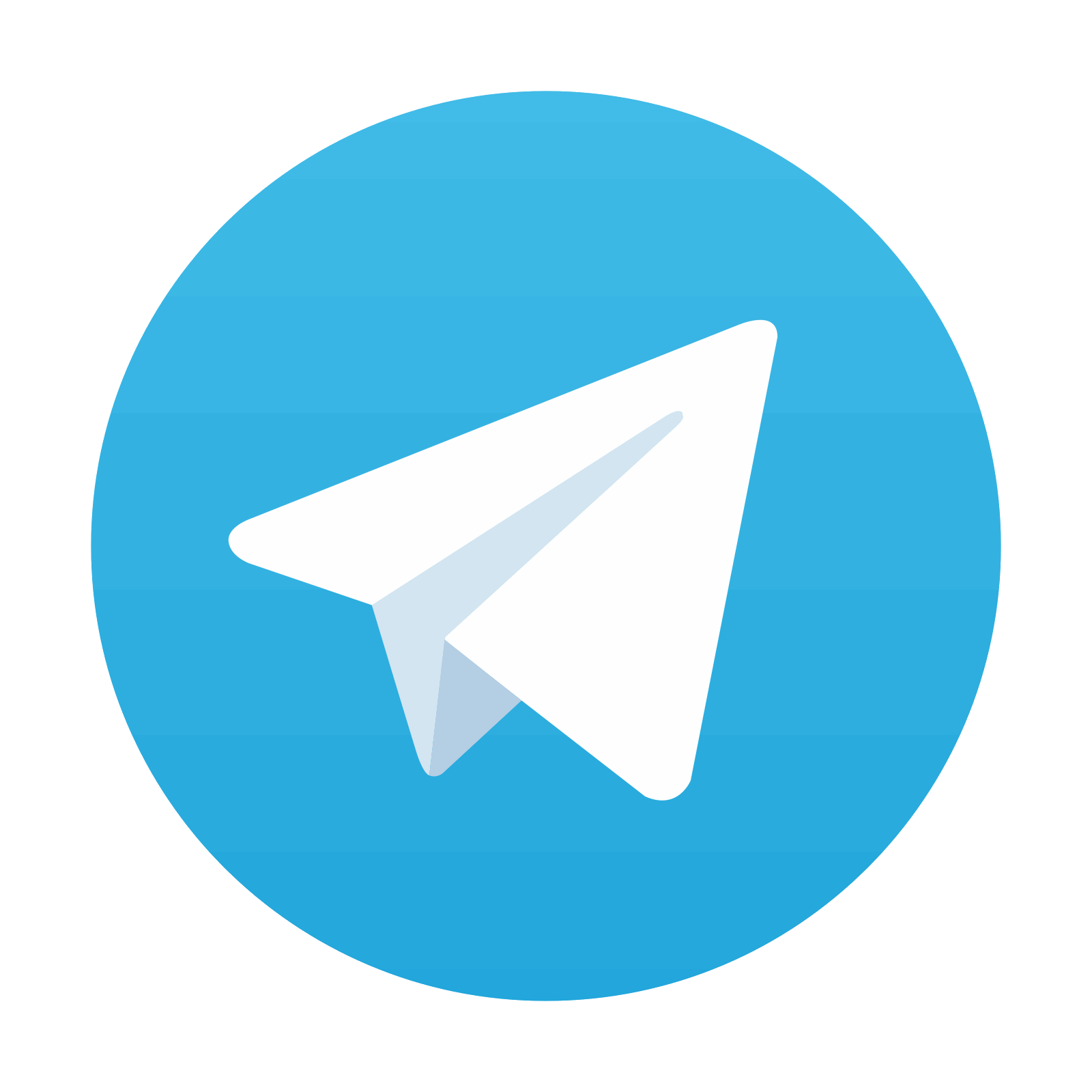
Stay updated, free articles. Join our Telegram channel

Full access? Get Clinical Tree
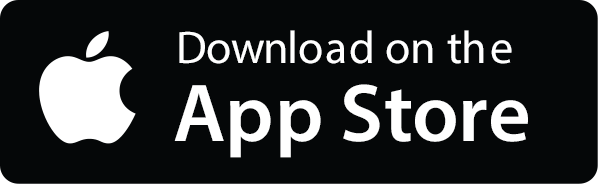
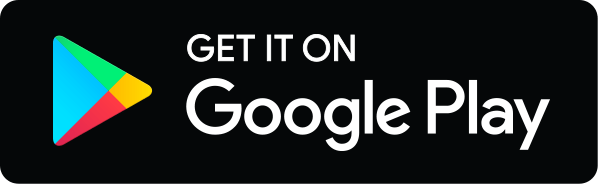
