Tumor Vasculature: Structure and Function
Janice A. Nagy
Amjad Husain
Shou-Ching Jaminet
Ann M. Dvorak
Harold F. Dvorak
Tumors Have Long Been Known To Induce Their Own Blood Supply, And The Vasculature They Generate Is Heterogeneous And Highly Abnormal, Differing From That of Normal Tissues With Respect To Organization, Structure, And Function. It Had Long Been Suspected That Tumor Blood Vessels Were Induced By Tumor-Secreted Products, Entities That Judah Folkman1 Referred To As “Tumor Angiogenesis Factors.” This Postulate Was Ultimately Proved Correct With The Discovery of Vascular Endothelial Growth Factor-A (Vegf-A), And With That Discovery Came The Recognition That Most Tumors Generate New Blood Vessels By A Common Mechanism (I.E., By Secreting Vegf-A).2,3 Vegf-A Was Originally Discovered As A Potent Vascular Permeabilizing Factor (Vascular Permeability Factor),4,5 And It Is Not Surprising, Therefore, That Tumor Blood Vessels Are Hyperpermeable To Plasma And Plasma Proteins. Coupled With A Lack of Efficient Lymphatic Drainage, The “Leakiness” of The Tumor Vasculature Leads To Extravascular Clotting And Accumulation of Interstitial Fluid (Edema) With Important Consequences For Generating Tumor Stroma.4,6,7,8 Subsequently, It Became Possible To Generate Surrogates of The Various Types of Tumor Blood Vessels In Mice Using An Adenovirus Engineered To Express Vegf-A, Thus Allowing Their Study Without The Necessity (And Complication) of Tumor Cells.9 This Advance Also Made Possible A Classification of Tumor Blood Vessels That Has Clinical Significance (Table 55.1), A Finding of Particular Importance Now That Anti-Vegf Therapy Has Been Included As A Therapeutic Regimen.
This Chapter Reviews The Structural And Functional Properties of Tumor Blood Vessels From The Points of View of Vascular Biology And Pathophysiology. Included Is A Discussion of The Steps And Mechanisms By Which The Several Types of Tumor Blood Vessels Form, The Differences That Distinguish Each of Them From The Blood Vessels of Normal Tissues, And The Importance of These Distinctions For Cancer Therapy With Agents Directed Against Vegf-A Or Its Receptors. It Is Hoped That This Information Will Provide A Useful Perspective For Investigative Radiologists, Supplementing With An Independent Approach And At Higher Resolution The Information They Currently Obtain In Cancer Patients With Dynamic Contrast Imaging Techniques.
Normal Microvasculature
The normal vasculature is the appropriate reference standard for evaluating the tumor vasculature. In normal tissues, blood from the heart proceeds through arteries, arterioles, and, thereafter, with a few exceptions, through capillaries, postcapillary venules, and veins. Although part of a continuum with some degree of overlap, each of these vessel types has a characteristic structure and function. Arteries are large vessels lined by a single layer of endothelial cells and coated with varying layers of elastic tissue and smooth muscle cells. Arterioles have a structure similar to nonelastic muscular arteries, but are smaller in size, typically 10 to 20 μm in diameter with a simpler smooth muscle cell coating. Tissue blood flow is exquisitely regulated, primarily at the arteriolar level, by
autonomic innervation and by local, endothelial cell-generated nitrogen oxide.
autonomic innervation and by local, endothelial cell-generated nitrogen oxide.
TABLE 55.1 TYPES OF TUMOR/TUMOR SURROGATE BLOOD VESSELS AND THEIR PROPERTIES | ||||||||||||||||||
---|---|---|---|---|---|---|---|---|---|---|---|---|---|---|---|---|---|---|
|
Capillaries are small vessels, typically 4 to 9 μm in diameter, that are lined by a thin, flattened, but, in most tissues, continuous endothelium and are enveloped by basement membrane and a variable coating of pericytes. Caveolae, vesicles approximately 70 nm in diameter,10 are prominent both in the cytoplasm and attached to the luminal and abluminal plasma membranes. In the central nervous system, caveolae are reduced in number and astrocytes contribute to a barrier that renders brain microvessels highly resistant to the nonspecific passage of plasma solutes. In some tissues (e.g., kidneys, endocrine glands), the endothelium is fenestrated rather than continuous. Fenestrae are 50 to 150 nm in diameter circular zones in which the normal trilaminar unit plasma membrane is replaced by extremely thin diaphragms of as yet incompletely determined composition.11,12 Capillaries are normally spaced at intervals of approximately 100 to 200 μm (i.e., distances corresponding to the effective diffusion range of oxygen). This distribution is important because capillaries are the principal “exchange vessels” responsible for tissue nutrition and waste disposal.
Venules are larger than capillaries, typically 20 μm or larger, and are lined by a cuboidal endothelium whose cytoplasm contains caveolae as well as a characteristic collection of interconnecting vesicles and vacuoles, the vesiculo-vacuolar organelle (VVO).13 Venules have key roles in both humoral and cellular inflammation and in tumor angiogenesis.
Tumor Blood Vessels: Organization, Structure, and Function
Organization
Whereas the normal microvasculature forms a hierarchy of evenly spaced, well-differentiated arterioles, capillaries and venules, tumor microvessels exhibit a chaotic pattern of hierarchical disorganization.14 A characteristic feature is spatial heterogeneity, uneven distribution of blood vessels with zones of both increased and sparse vascular density. Tumor vessels commonly exhibit a serpentine course, branch irregularly, and form arteriovenous shunts. Vessels are often most abundant at the tumor–host interface, where they often form a prominent, circumferential mantle. Internal portions of solid tumors are typically less well vascularized.15 Blood flow through the tumor vasculature does not follow a consistent, unidirectional path. Rather, the tumor vasculature consists of a maze of interconnected vessels through which blood flows haphazardly and irregularly. Not all open vessels are perfused continuously, and, over short periods of time, blood flow may follow different paths and actually reverse direction.16
The tumor vasculature is also heterogeneous over longer timeframes.14 Vascular volume, length, and surface area all increase during early stages of tumor growth. As a result, small tumors tend to be relatively well vascularized. Nevertheless, as tumors grow, the vasculature fails to keep pace, and the tumor blood supply becomes progressively deficient. Thus, tumors are said to outgrow their blood supply or, put in other words, the developing vasculature and other stromal elements fail to keep pace with tumor cell growth.
Structure
Surprisingly little has been written about the structure of tumor blood vessels, although it has long been recognized that they differ extensively from their normal counterparts. Writing in the late 1970s, Warren14 described eight or more distinct types of tumor vessel, not all of which were found in any one tumor. Although this classification has been superseded (Table 55.1), it was useful at the time because it called attention to the fact that tumor blood vessels are heterogeneous and not of a single type. Warren concluded that an important difference between the tumor and normal vasculature was that in the former, capillaries and veins became tortuous and dilated. In fact, the notion that tumor vessels are “dilated” is a misconception that is still widely voiced. Dilatation is correctly understood as vessel enlargement engorgement that results from the relaxation of vascular smooth muscle cells, as, for example, in the response of normal arterioles to nitric oxide. Instead, the enlarged size of many tumor blood vessels reflects fundamental abnormalities in their generation, not smooth muscle relaxation.
Function
It was supposed at one time that tumors had a blood supply superior to that of normal tissues. However, in now classic studies, Gullino17 demonstrated that blood flow in animal tumors was significantly lower than in normal tissues, reflecting reduced overall vascular density that was reduced further as tumors increased in size. Not unexpectedly, a combination of abnormal tumor vessel organization, structure, and reduced blood flow leads to poor tumor perfusion. The spatial heterogeneity of tumor blood vessels results in uneven delivery of oxygen and nutrients and uneven clearance of waste products; together these lead to zones of metabolic insufficiency, ischemia, and necrosis. Also, the increased average diameter of tumor vessels results in an altered surface area to volume ratio that further impairs tissue nutrition. As a result, and also because of arteriovenous shunts, tumors fail to take up nutrients efficiently, as is manifest by the higher than normal oxygen content of the venous blood draining tumors. Poor clearance of carbon dioxide and other metabolites,
coupled with high tumor cell glycolysis, result in overall tumor acidity (pH ∼ 7.2 vs. pH ∼ 7.4, reviewed in Dvorak18).
coupled with high tumor cell glycolysis, result in overall tumor acidity (pH ∼ 7.2 vs. pH ∼ 7.4, reviewed in Dvorak18).
A number of additional factors contribute to the relatively poor blood flow in tumors compared with normal tissues. In effect, all of the variables affecting blood flow are altered in tumors (reviewed in Jain19). Blood flow is proportional to the drop in blood pressure across a vascular bed and inversely proportional to blood viscosity and extrinsic geometric resistance, a complex function of vascular morphology dependent on vessel number and types and their branching pattern, diameter, and length. Whereas pressures in the arteries supplying normal and tumor vessels are quite similar, microvascular pressures within tumors are actually elevated due to venous compression, whereas pressures in draining veins are significantly reduced.20 Also, tumor blood vessels exhibit greater resistance to flow than do the vessels supplying normal tissues. Because of their serpentine course, thin walls, exposure to increased interstitial pressure, and other local factors, the extrinsic geometric resistance may be increased in experimental tumors by more than 10-fold. Finally, the viscosity of the blood within tumor vessels is increased because vascular hyperpermeability, a characteristic feature of the tumor vasculature, results in plasma leakage with consequent increased hematocrit, rouleaux formation, and a resulting increase in shear rate.
Attempts have been made to manipulate tumor blood flow with vasoactive drugs, but the response has been inconsistent.21 In general, the vascular beds of most tumors behave as rigid tubes that are in a state of near-maximal diameter; thus, there is little ability to increase flow in response to increasing vascular pressure. One reason for this is that many tumor vessels lack normal coatings of smooth muscle cells (see below). Another reason may be that, unlike normal vessels, tumor blood vessels lack innervation and therefore their smooth muscle cells are not subject to autonomic regulation. The result is low maximum perfusion capacity (i.e., high vascular resistance) compared with that of most normal tissues.
Vascular Endothelial Growth Factor-A and Tumor Blood Vessel Generation
Very early in their growth, at very late stages of malignant progression, and following treatment, some tumors can satisfy their nutritional and waste removal needs by coopting the normal vasculature. Sometimes this becomes dramatically manifest as tumor cells form cuffs that surround pre-existing normal blood vessels.22 Nevertheless, for the most part, tumors must generate new blood vessels if they are to grow beyond minimal size. Although both endothelial and smooth muscle cells participate in tumor blood vessel generation, it is endothelial cells and their replication that have attracted the most attention. A lengthy search for a selective endothelial cell mitogen culminated in the discovery of VEGF-A. Nevertheless, the significance of VEGF-A lies not only, or even principally, in its endothelial cell mitogenicity (it is a selective but relatively weak endothelial cell mitogen). A number of other activities appear to be equally or more important. These include the ability of VEGF-A to induce vascular permeability, to reprogram the endothelial cell gene expression pattern, and to maintain endothelial cell health and survival (reviewed in Dvorak2,18).
A considerable body of evidence attests the importance of VEGF-A in tumor blood vessel generation (as reviewed elsewhere18,23,24,25,26). Nearly all malignant tumors express VEGF-A at high levels and exhibit increased vascular permeability; premalignant tumors of several types (e.g., cervix, breast) express VEGF-A at low levels and at progressively higher levels as they become invasive; several other growth factors, as well as oncogenes and inactivation of tumor suppressor genes, act in part to upregulate VEGF-A expression. Blocking VEGF-A or its receptors (VEGFR-2, KDR) can effectively inhibit the growth of many animal tumors and is of benefit in treating some human cancers.
Blood vessels’ formation is difficult to study in tumors because they comprise only a minority of the tumor mass and because of secondary complications such as necrosis and inflammatory cell infiltration. It became desirable, therefore, to look for a means of generating surrogate tumor blood vessels in the absence of tumor cells. Because of the prominence of VEGF-A as a tumor angiogenesis factor, it seemed possible that the various types of blood vessels found in tumors could be induced by overexpressing VEGF-A in normal tissues without the need for tumor cells. In fact, this approach is feasible. Local overexpression of VEGF-A by a variety of methods (implants of VEGF-A protein in Matrigel [BD Bioscience, San Jose, CA] or collagen, viral vectors, etc.) leads to the formation of abnormal vessels that closely resemble those found in tumors.2,9,27 Adenoviral vectors expressing VEGF-A164 (the mouse equivalent of human VEGF-A165) have proven to be especially useful for this purpose because they infect normal, nondividing cells and do not require introduction of a foreign matrix. A caveat is that these studies must be performed in immunodeficient mice to avoid the strong immune reaction directed against the adenoviral vector. Also, the response induced by adenoviral VEGF-A164 (Ad-VEGF-A164) differs from that in tumors in an important respect. Whereas tumors express VEGF-A continuously in large amounts over long periods of time, expression of Ad-VEGF-A164 is transient. Adenoviruses are not incorporated into the genomes of the cells they infect and therefore direct new VEGF-A164 synthesis for only a limited period of time—days to weeks—before being discarded. As a result, Ad-VEGF-A164 effectively generates only a single round of new blood vessel formation. Nevertheless, this property has proved to be advantageous
in that it has facilitated an understanding of the sequential steps by which VEGF-A164 induces new blood vessel formation.
in that it has facilitated an understanding of the sequential steps by which VEGF-A164 induces new blood vessel formation.
Surrogate Tumor Blood Vessel Formation in Mice with Ad-VEGF-A164
When injected into immunodeficient mouse tissues, Ad-VEGF-A164 infects normal host cells, which then become local factories that synthesize and secrete VEGF-A164 in tumor-like quantities for a limited period of time. VEGF-A164 initiates a cascade of events that generates all of the six different blood vessel types found in human and animal tumors (Fig. 55.1) (Table 55.1).2,9,28 Four of these result from angiogenesis (i.e., the generation of new blood vessels from microvessels, primarily venules and capillaries): mother vessels (MVs); capillaries; glomeruloid microvascular proliferations (GMPs); and vascular malformations (VMs). Two other vessel types (feeding arteries [FAs] and draining veins [DVs]) result from the enlargement and remodeling of pre-existing normal arteries and veins (i.e., they arise by a process of arterio-venogenesis). Like tumor vessels, which show limited tissue specificity, the new blood vessels induced by Ad-VEGF-A164 are largely independent of the tissues in which they arise, that is, similar vessel types form in response to VEGF-A164 and with similar kinetics in a wide variety of normal mouse and rat tissues, including skin, subcutaneous space, fat, skeletal and heart muscle, and brain.2,9,29 Ad-VEGF-A164 also induces abnormal lymphangiogenesis, leading to the formation of greatly enlarged, poorly functional lymphatics.30 The extent to which new lymphatics are induced in tumors and their significance for tumor metastasis is the subject of considerable debate but is beyond the scope of this chapter. The properties of the types of tumor surrogate blood vessels induced by Ad-VEGF-A164 are described below and in Table 55.1.
Mother Vessels
The term mother vessel was initially proposed by Paku and Paweletz to describe the first type of new blood vessel to form in experimental tumors in mice.18 MV are also the first type of new blood vessel to develop in response to Ad-VEGF-A164 and are the blood vessel subtype that is primarily responsible for the well-known hyperpermeability of tumor blood vessels (Figs. 55.2A–C and 55.3). Because of their hyperpermeability, MV have little retained plasma, and their lumens are packed with red blood cells; as a result, blood viscosity is increased, flow is sluggish, and thrombosis not uncommon.
MV arise from pre-existing normal venules and capillaries by a process that involves vascular basement membrane (VBM) degradation, pericyte detachment, extensive endothelial cell thinning, and a substantial increase in lumen size. Swayne demonstrated that VBM
are noncompliant (nonelastic) structures that limit microvessel expansion to approximately 30%.18 Therefore, to acquire an increase in cross-sectional area that is four- to fivefold greater than that of the venules and capillaries from which they arise, VBM must be degraded. Recent data have shown that VBM degradation results from increased expression of cathepsin proteases (B > S > L), accompanied by decreased expression of a family of small (11 to 13 kD) proteins called cysteine protease inhibitors (CPI), which are widely expressed in normal tissues and are high-affinity competitive inhibitors of cysteine proteases such as the cathepsins. Pericytes of venules that evolve into MV proved to be the source of increased cathepsin expression and activity,31 whereas expression of CPI was strikingly reduced in both endothelial cells and pericytes. Taken together, these data indicate that MV formation results from an upsetting of the normal cathepsin to CPI balance that regulates vascular size. Increased pericyte cathepsins, relieved of CPI inhibition, degrade VBM, causing pericytes to detach, resulting in the formation of MV, structures that are lined only by endothelial cells.
are noncompliant (nonelastic) structures that limit microvessel expansion to approximately 30%.18 Therefore, to acquire an increase in cross-sectional area that is four- to fivefold greater than that of the venules and capillaries from which they arise, VBM must be degraded. Recent data have shown that VBM degradation results from increased expression of cathepsin proteases (B > S > L), accompanied by decreased expression of a family of small (11 to 13 kD) proteins called cysteine protease inhibitors (CPI), which are widely expressed in normal tissues and are high-affinity competitive inhibitors of cysteine proteases such as the cathepsins. Pericytes of venules that evolve into MV proved to be the source of increased cathepsin expression and activity,31 whereas expression of CPI was strikingly reduced in both endothelial cells and pericytes. Taken together, these data indicate that MV formation results from an upsetting of the normal cathepsin to CPI balance that regulates vascular size. Increased pericyte cathepsins, relieved of CPI inhibition, degrade VBM, causing pericytes to detach, resulting in the formation of MV, structures that are lined only by endothelial cells.
Vascular enlargement, the final step in MV formation, is thought to be driven by centripetal intravascular pressure on endothelial cells that have lost the constraints normally imposed by VBM and pericytes. To accommodate this increase in lumen size, previously cuboidal venular endothelial cells thin extensively and expand to cover a greatly enlarged surface area, a process that requires substantial increases in plasma membrane. Although some membrane may derive from new synthesis, a significant amount comes from the transfer to the cell surface of membrane that is normally stored in the venular endothelial cell cytoplasm in the form of VVOs, which are grape-like clusters of hundreds of uncoated, largely parajunctional cytoplasmic vesicles and vacuoles that together form an organelle that traverses venular endothelial cytoplasm from lumen to albumen.13,32 VVOs also open to the interendothelial cell cleft, either below or above sites of specific junctional (adherens or tight junctions) attachments. VVO vesicles and vacuoles are linked to one another and to the plasma membrane by stomata that are normally closed by thin diaphragms that appear similar to those found in the caveolae found in capillaries. MVs are unstable structures that evolve into several types of “daughter” vessels.2,9
Glomeruloid Microvascular Proliferations
GMPs are poorly organized vascular structures that resemble renal glomeruli macroscopically (hence the name) (Fig. 55.2F, G).9,33 They are found in a wide variety of human tumors, particularly glioblastoma multiforme but also in cancers of the stomach, breast, and others. Recently, GMPs have been reported to correlate with poor prognosis.34 All of the human tumors known to form GMPs express VEGF-A, and tumors such as glioblastoma multiforme that make especially large amounts of VEGF-A are among those that form GMPs most commonly. Like MVs, GMPs are hyperpermeable to plasma and plasma proteins, but, because they are poorly perfused, they contribute relatively little to tumor vessel permeability.
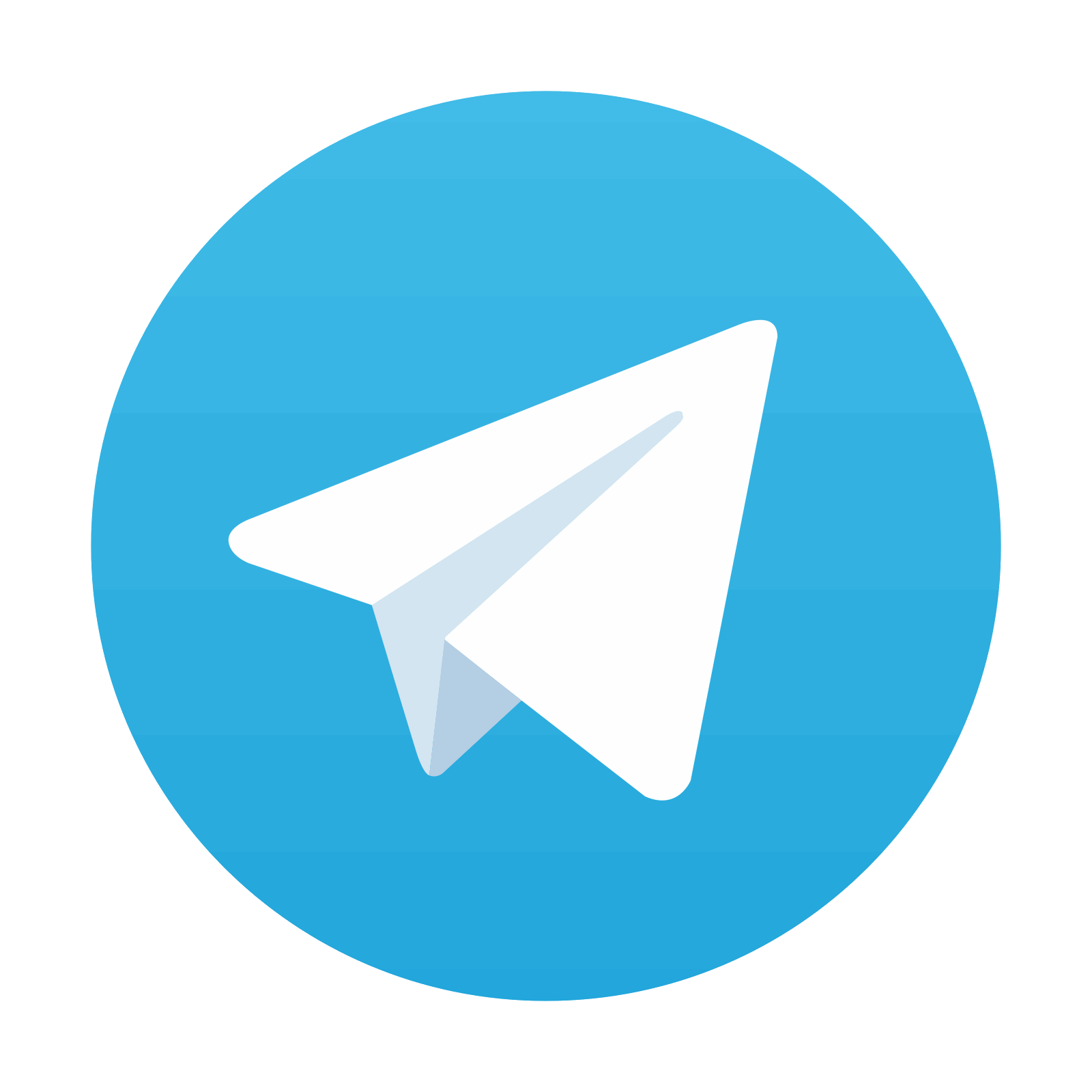
Stay updated, free articles. Join our Telegram channel

Full access? Get Clinical Tree
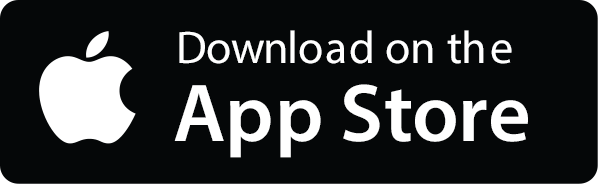
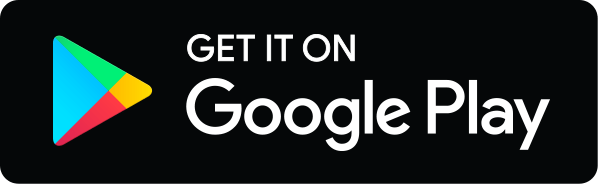