7 Ultrasound Contrast Agents and Contrast-Enhanced Ultrasonography Imaging the internal organs is crucially important for identifying diseases and for treatment and follow-up. Diagnostic imaging has made tremendous technological progress in recent years, making possible ever more advanced and more detailed examinations of anatomy and physiology. In addition to techniques such as multislice computed tomography (CT), whole-body magnetic resonance imaging (MRI), and positron-emission tomography (PET), which require large and expensive machines, there is still a substantial need for easily available, patient-friendly, and inexpensive methods such as ultrasonography. However, the technique needs to fully meet the standards of modern diagnostic imaging in all respects. These requirements have become substantially more demanding over the years. Following the introduction of contrast agents, the original simple imaging of anatomical structures was extended to imaging of the blood supply (vessels and perfusion) and, most recently, the imaging of molecular structures (molecular imaging) with the aid of tissue-specific contrast agents. All such investigations can in principle be performed with sonography as well. This chapter provides an overview of the contrast agents currently available, with their most important properties, and of contrast-specific sonographic techniques that allow selective imaging of contrast agent signals. The basis of all sonographic examinations is B-mode sonography, which uses differences in echogenicity in tissue to produce detailed high-resolution images of anatomic structures. In addition, Doppler sonography allows imaging of the blood flow in vessels and the derivation of spectra for the quantitative determination of blood flow over time. However, Doppler sonography has serious limitations: it only functions at fairly high flow rates (greater than the wall motion), for flow in a defined direction toward or away from the transducer, and for adequate flow volumes. Imaging of capillary flow (small volumes, very slow speeds), particularly in tumors with complex vascular architecture, is not possible using this technique. For this purpose, contrast agents that allow the imaging of blood on the basis of specific parts of the contrast signal are required. Ultrasound contrast agents were originally developed to improve the contrast of weak (noisy) echo signals, but a combination with contrast-specific scanner techniques made a completely new application available: B-mode imaging of very small quantities of contrast agent (equivalent to blood volumes) in tissue (parenchyma). This requires sensitive detection (right down to the individual microbubbles) and selective detection (to distinguish between tissue signals and noise) of the contrast agent, making it possible to image the blood supply in tissue. This type of contrast-specific sonography firstly allows the imaging of vascularity and vessel geometry in organs (capillary volume); and secondly, can image and quantify the passage of a contrast agent bolus through the vascular system in real time (capillary flow). Capillary volume and capillary flow are the determinants of parenchymal perfusion. The principle of ultrasound contrast agents has been known for more than 30years, since Gramiak and Shah observed strong ultrasound echo signals in blood following the injection of indocyanine green.1 The signals were formed as a result of air bubbles that were co-administered during the rapid bolus injection. Since that time, the intentional creation of air or gas bubbles of this type has been used to produce echogenic solutions that make the hypoechoic blood visible using ultrasound. Home-made ultrasound contrast agents of this type were prepared, for example, through vigorous shaking of physiological saline or viscous infusion solutions, or by sonicating radiographic contrast agents. As their lack of stability inhibited pulmonary passage, they were mainly used for shunt diagnosis2,3 or to image myocardial perfusion after intracoronary administration.4,5 Since 1991, standardized ultrasound contrast agents have been available for right-heart diagnosis (Echovist) and, since 1995, ultrasound contrast agents that are stable on passage through the lungs (Levovist) have been commercially available. Since 2001, second-generation contrast agents containing gases with low solubility in water have been on the market (SonoVue) and have substantially increased the stability and duration of the contrast. Classification of Ultrasound Contrast Agents The original objective in developing standardized ultrasound contrast agents was to obtain products that pass through the lungs, making contrast imaging of the entire vascular system possible after intravenous injection (blood-pool agents). Most products available or in development today fall into this category. Ideally, blood-pool contrast agents should be transported freely in the bloodstream without leaving the vascular bed or accumulating in specific tissues (Table 7.1). Some of these contrast agents do not move perfectly freely with the bloodstream, but have some tissue-specific affinity. This means that they accumulate in specific tissues—e.g., the reticuloendothelial cells in the liver and spleen—at the end of the vascular phase. This effect has been described for Levovist6,7 and Sonazoid8,9 and can be used diagnostically to identify functional liver tissue in the late, liver-specific, phase (i.e., after the end of the vascular phase). Care needs to be taken to ensure that the late-phase examination is not recorded too soon, to avoid overlap with the portal venous phase. These are contrast agents that have a high affinity to specific tissues or molecular structures and which therefore accumulate specifically in these tissues. In principle, this includes the above blood-pool contrast agents with liver-specific late phases. In the narrower sense, however, it is taken to mean contrast agents with a shell containing structures with high molecular affinities (e.g., antibody fragments). Currently, no tissue-specific contrast agents are available for use in humans, but several substances are in preclinical development.10–14 Contrast agents of this type make it possible to detect neoangiogenesis, intravascular thrombi, plaques, or inflammation, for example. The shells of ultrasound contrast agents can be used not only for the transport of gases or air, but can also transport drugs in the body. The release of these drugs then takes place locally, either through nonspecific rupture of the shell after specific binding to the target tissue (target-specific microbubbles) or by selective local rupture of the freely circulating microbubbles in the target area (e.g., by exposure to ultrasound). Systems of this type can be used for the transport of conventional drugs or even of DNA or RNA fragments (e.g., antisense molecules).15,16 After initial in vitro and in vivo experiments with stains and marker DNA, studies are now underway with functional DNA and have already resulted in measurable clinical effects in animal studies. It is helpful that ultrasound can be used not just to release the active substance, but also allows penetration into the tissue (sonoporation). Products of this type are already in preclinical testing. These were generally developed as blood-pool contrast agents; however, the administration route is not intravenous, but rather by catheter or needle into a body cavity. Established indications are contrast hysterosalpingosonography to assess the patency of the tubes when investigating fertility,17 and voiding urosonography to investigate vesicoureterorenal reflux, particularly in children.18 These are actually negative contrast agents, as they do not produce echogenicity, but eliminate the interfering echogenicity in the gastrointestinal tract caused by the presence of air. At present, the sole product approved for this purpose is only available in the United States (SonoRx). The drinkable liquid contains cellulose fibers that adsorb the air that causes interference, thus allowing overlap-free examination of organs behind the gastrointestinal tract.19 Mode of Action of Ultrasound Contrast Agents The basic principle of ultrasound contrast agents is the creation of many small interfaces with high echogenicity. This is ideally achieved using gaseous microbubbles. To increase the stability of microbubbles in the blood and achieve a standardized size, the bubbles are surrounded by a shell. There are products with hard shells (e.g., galactose microparticles, denatured albumin) and products with flexible shell membranes (e.g., phospholipid shells). In terms of the gas, a distinction is made between products containing air (first-generation products) and those with low-solubility gases (second-generation products) (Fig. 7.1). The latter produce longer-lasting contrast, as the gas they contain only dissolves slowly in the surrounding blood. The microbubbles usually have a diameter of 2–10 pm, which is about the size of the red blood cells. Unlike common CT and MRI contrast agents, therefore, they do not pass into the interstitial fluid, but remain confined to the vascular system (blood-pool contrast agents). This substantially simplifies the assessment of tissue perfusion, as the contrast agent distribution can be considered equivalent to the distribution of the blood. Luckily, the resonance frequency of microbubbles of this size is in the region of the sound frequencies used for diagnostic imaging, which makes it possible to generate a harmonic response. When sound waves strike the microbubbles, they are reflected from the surface. More precisely, the process is known as backscattering. The backscattered sound waves have the same wavelength as the emitted sound waves. This backscattering behavior is referred to as the linear behavior of the microbubbles. The microbubbles in ultrasound contrast agents are very effective backscatterers. They increase the signal intensity by more than 30 dB,20 which corresponds to a factor of 1000 in the received sound intensity. Fig. 7.1a, b Composition of an ultrasound contrast agent micro-bubble. Microscopic image (a) and schematic configuration (b) of SonoVue microbubbles. A flexible outer shell of phospholipids surrounds the enclosed SF6 gas. The phospholipids form a monolayer, with the lipid side facing inward (toward the gas) and the hydrophilic side pointing outward (toward the blood). However, as the sound pressure increases, nonlinear behavior of the microbubbles becomes increasingly prominent. The bubbles first start to oscillate, sending out harmonic oscillations,21,22 and then, as the sound pressure increases further, the microbubbles become unstable, start to split up, and finally break down. In the process, they send out a brief high-energy signal (stimulated acoustic emission, SAE)23,24 (Fig. 7.2). In principle, all ultrasound contrast agents show this behavior, but the absolute level of sound energy at which the harmonic response and the rupture of the microbubbles sets in varies from one contrast agent to another. Microbubbles with flexible shells start to oscillate at low sound energies and show pronounced harmonic behavior. Hard-shelled contrast agents, on the other hand, give a very good SAE signal on rupture. Fig. 7.2 Behavior of microbubbles as a function of insonation pressure. The behavior of microbubbles in the acoustic field depends primarily on the insonation pressure. If the acoustic pressure is very low, passive backscattering of the input signal occurs. At somewhat higher acoustic pressures, the microbubbles start to oscillate at their resonance frequency. If the acoustic pressure is too high, the microbubbles break down. LOC, loss of correlation; MI, mechanical index. Fig. 7.3 Oscillation of microbubbles in a sound field. Compression of the microbubbles during the high-pressure phase (against the internal gas pressure) is less than expansion in the low-pressure phase. The fluctuation in the diameter of the microbubbles is therefore asymmetrical and is not a linear function of pressure fluctuation—a nonlinear response. During oscillation, the microbubbles produce a nonlinear echo signal.25 This is because the compression of the microbubbles against the pressure of the inner gas content is less than the expansion. This leads to asymmetrical fluctuation of the bubble diameter, which is no longer linearly dependent on the sound pressure (Fig. 7.3). Ultrasound contrast agents are usually administered by intravenous bolus injection. To achieve the fastest and most complete possible wash-in of the contrast agent bolus, flushing with 5–10 mL of physiological saline is recommended.26 The injection should ideally be given in a large-volume arm vein, with the syringe connected to the indwelling cannula directly or via the straight part of a Tconnector. If there are compelling reasons for interposing an infusion tube, it must be as short as possible and the inner lumen should not be too tight. Particularly for measuring perfusion profiles (e.g., to characterize lesions), the injection has to be given rapidly to obtain sharp temporal separation. Fig. 7.4 Course of the microbubble concentration in blood after bolus administration. After bolus injection, there is a rapid increase in the contrast-agent concentration up to a maximum (peak intensity), followed by a somewhat slower wash-out phase. If the attenuation threshold or even system saturation is reached at the maximum, artifacts such as shadowing or blooming can occur. After the bolus injection, there is a rapid increase in the microbubble concentration, followed by a slow wash-out over several minutes. Attention has to be given to three threshold values (Fig. 7.4): • System saturation. This threshold is frequently reached right at the start of contrast wash-in and gives rise to saturation artifacts and (in color Doppler) blooming effects.
Tissue-Specific Contrast Agents
Product | Concentration | Dose |
Levovist | 200 mg/mL 300mg/mL 400mg/mL | 10–16 mL 5–10mL 5–8 mL |
Optison |
| 0.5–3.0 mL |
SonoVue |
| (0.6-)1.2–2.4 mL |
• Attenuation threshold. Above this threshold, there is a strong echo signal close to the transducer because of the high microbubble concentration; distal to this there is shadowing, like that seen behind hyperechoic tissue structures.
• Detection threshold. Below this threshold, the microbubble concentration is too low and the microbubbles can no longer be detected.
The diagnostic window within which the bubble concentration is optimal for imaging is located between the detection threshold and the attenuation threshold. The absolute level of these thresholds depends on the machine used, the contrast software installed, and the machine settings. Most machines now have presets suitable for contrast agents, allowing rapid selection of the most important parameters for optimal contrast imaging.
The signal strength (gray value or power Doppler intensity) is an approximately linear function of the bubble concentration, allowing quantitative assessment of the perfusion. However, this only applies at contrast agent concentrations that are not too high (below the attenuation threshold).
The optimal dose of contrast agent depends, among other things, on the technique used (B-mode, Doppler, harmonic mode, etc.), the settings and sensitivity of the ultrasound machine, the target organ, and the contrast enhancement required. With the newer second-generation contrast agents, doses of 1–5mL are usually adequate. The quantity of gas they contain is very low (e.g., only 8 pL/mL in the case of SonoVue), which illustrates the high sensitivity of contrast agent imaging. The dosages shown Table 7.2 are recommended as standard values. With the most recent scanner technology, even smaller doses down to less than 1 mL per injection are possible.
If longer contrast times with uniform contrast enhancement are required, a continuous infusion of microbubbles can be given.26,27 The infusion rate has to be adjusted so as to provide uniform contrast without saturation artifacts. For longer infusion times, it should be noted that the microbubbles float toward the top of the carrier liquid over time and become concentrated on the surface of the liquid in the syringe. Regular, or preferably continuous, mixing of the solution is recommended (e.g., with special infusion pumps).
Artifacts Caused by Contrast Agents
The high amplitude of the backscattered signal can lead to saturation of the receiver electronics at high microbubble concentrations. In color Doppler, this takes the form of smearing of the color signal (color blooming), which also occurs at high color amplification or transmitter power.28 This effect often occurs shortly after wash-in of the contrast agent and disappears as the microbubble concentration declines again.
At very high sound pressures, large quantities of micro-bubbles become unstable and finally break down. This gives rise to high energy signals (stimulated acoustic emission). If these signals mix with normal backscattered signals, they lead to an artificial echo image (bubble noise).28 However, when such signals are generated deliberately, they can be used for imaging.
The destruction of microbubbles in regions of high sound pressure (e.g., close to the transducer in the focal plane) can also lead to attenuation phenomena that simulate lack of contrast enhancement. This can be avoided by reducing the sound energy and/or lowering the focal plane.
During sonography of regions with low blood-flow velocities (capillary beds), the section of the image affected only fills up again very slowly after destruction of the microbubbles. This can lead to bleaching of the contrast effect in tissue, which might be misinterpreted as an absence of contrast agent. In such cases, the perfusion can still be imaged by sonography at a reduced imaging rate (intermittent imaging).29,30 Individual images (frames) are recorded and displayed as still images until the next frame is captured. The length of the delay is normally 1–10 seconds (i.e., one frame is recorded every 1–10 seconds), depending on the strength of the blood flow. Recording of the frames can also be synchronized with the cardiac pulse (triggered imaging). However, in more recent procedures, bubble rupture is usually avoided by reducing the transmission power (i.e., using a low mechanical index procedure).
If the microbubble concentration is too high, very strong echo signals are produced close to the transducer, leading to shadowing or attenuation of deeper-lying structures.31 The effect corresponds to the attenuation phenomenon behind very echogenic structures. Increasing the signal intensity by administering higher doses of contrast agent is therefore only possible to a limited extent, so that it is better to use more sensitive, contrast-specific techniques.
Overestimated Flow Rates in Spectral Doppler
If the machine parameters are set correctly (i.e., amplification, wall filter, etc.), correct flow rates can also be measured with contrast-enhanced ultrasonography. If the signal is too strong, the Doppler gain may have to be reduced. There may be differences in the measured values in comparison with ultrasonography without contrast enhancement, if the maximum flow rate (i.e., the upper limit of the Doppler spectral curve) is not detected because the signal strength is too low.28,32 This is often the case for pathological flow in small volumes (e.g., flow in a stenosis, reflux jet, or shunt flow). In this situation, contrast enhancement contributes to more reliable measurement.
Fig. 7.5 The principle of harmonic imaging. In harmonic imaging, contrast-specific parts of the signal are separated from tissue signals using a frequency filter. However, this also suppresses nonlinear signals in the range of the fundamental frequency.
Contrast-Specific Imaging Procedures
Contrast-specific imaging procedures make use of the nonlinear behavior of the contrast-agent microbubbles for selective imaging of the ultrasound contrast agent, and thus of the blood volume.
There are numerous technical approaches to separating the (nonlinear) contrast-agent echo from the (linear) tissue echo and the artifact echoes (noise, speckle, clutter, motion artifacts in Doppler, etc.). The objective of each of these procedures is to optimize the contrast-tissue ratio and the contrast-noise ratio.
The proper separation of contrast-agent signals from background signals is a quality parameter for the contrast-specific imaging modality of the ultrasound scanner. Ideally, the contrast-specific image should be completely black before arrival of the contrast agent. Any background signals (e.g., from tissue) visible at baseline due to insufficient signal separation may be misinterpreted as perfusion.
In frequency filter procedures (harmonic imaging), the nonlinear signals in the region of the second harmonic frequency (i.e., twice the transmitted frequency) are separated out by means of a filter. Thus, only a part of the frequency spectrum that consists exclusively of nonlinear signals is used for imaging. The filter removes those parts of the signal at the fundamental frequency f0, and only those signals in the region of the second harmonic frequency are used for imaging (Fig. 7.5).
The advantage of this procedure is that it reduces the tissue signal (although, because of the formation of harmonics during the propagation of the sound wave, the echoes are not completely suppressed) and provides a clear reduction in noise artifacts (e.g., clutter). The contrast signal is thus more intense in comparison with the tissue (signal-tissue ratio) and noise (signal-clutter ratio), but not completely selective. The disadvantage of harmonic imaging is the need to use narrow-band transducers with lower resolution (to avoid overlapping of the two frequency bands) and the high sound energy required (as the most intense part of the backscattered signal is not used for imaging). Harmonic imaging thus generally ruptures a relatively large proportion of the microbubbles.
Fig. 7.6 The principle of the pulse summation procedure (pulse/phase inversion). In the pulse summation procedure, the contrast-specific parts of the signal are separated from the tissue signal by co-evaluation of several successive pulse sequences. This produces very good suppression of the tissue signal and high sensitivity for contrast-agent signals.
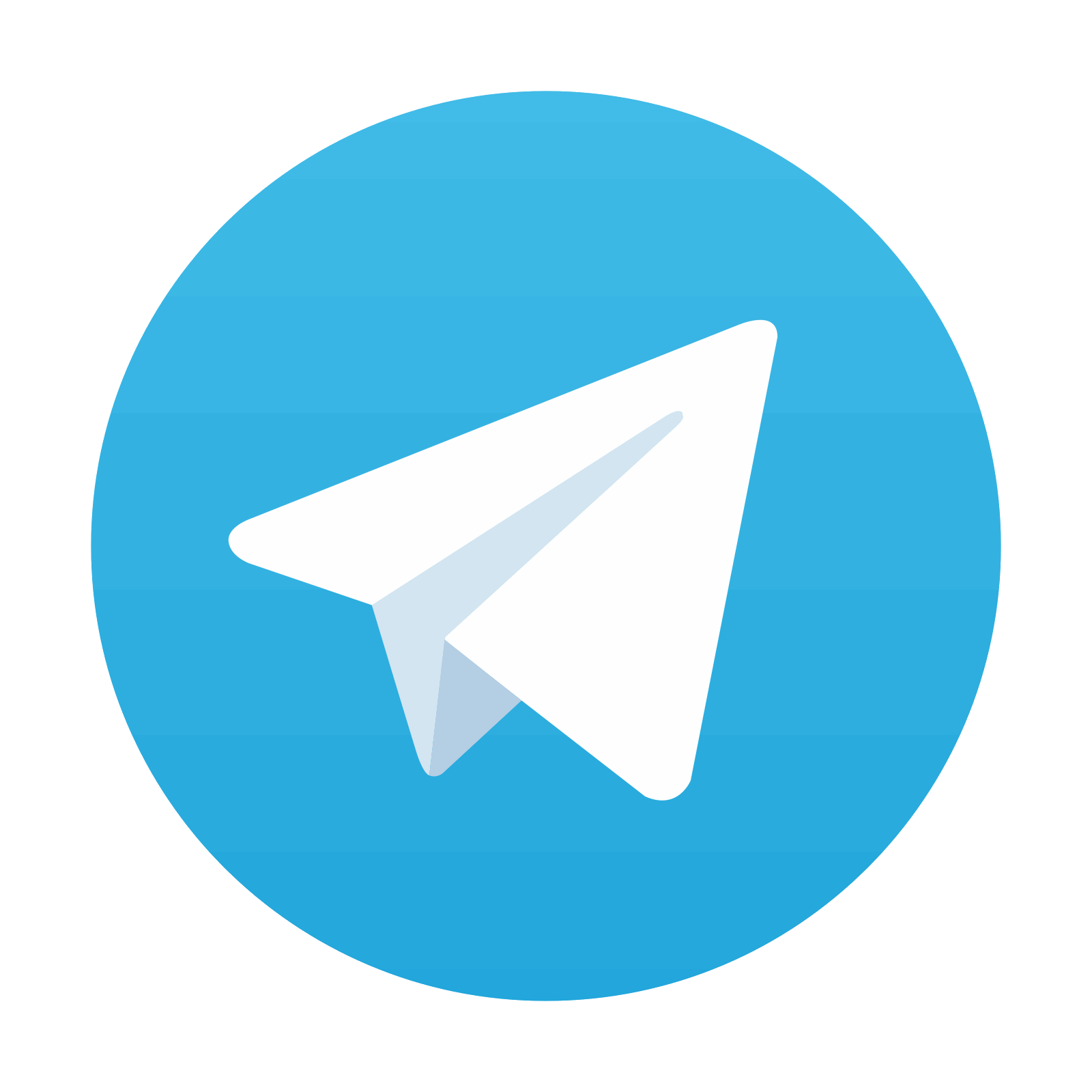
Stay updated, free articles. Join our Telegram channel

Full access? Get Clinical Tree
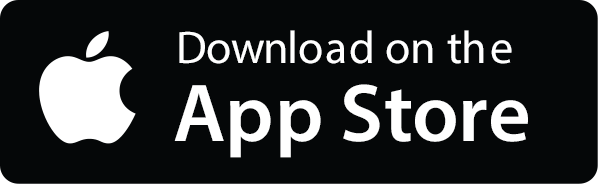
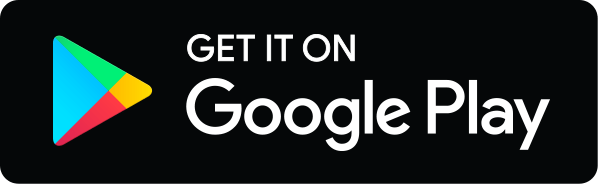