Fig. 20.1
Measurement of endometrial thickness
An important strategy to facilitate ART success is to increase the number of mature oocytes at the time of ovum pickup (OPU). Therefore, follicular maturation and timing of oocyte development and retrieval must be geared to maximize the mature oocyte yield, thus providing the best chance of an in vitro fertilization (IVF) success. US monitoring plays a crucial role in this process, even in the event of unanticipated difficulties encountered during embryo transfer. Following ART and successful implantation, US is necessary to monitor the course of pregnancy: (1) to confirm fetal heartbeat, (2) to detect fetal growth restriction, and (3) to detect fetal abnormalities such as anencephaly, spina bifida, or cardiac defects.
Regarding the course and outcome, there are major differences between a natural cycle and a hormonally induced, controlled ovarian hyperstimulation (COH) with multifollicular growth with ART. Usually, in a natural menstrual cycle, an average of 10 follicles compete for dominance; however, ultimately only one follicle prevails and only one oocyte becomes mature; the other follicles degenerate. Within COH, the preconditions and course differ completely from a natural cycle.
Although the stimulation protocols might vary, IVF is usually based on the administration of gonadotropins, which are glycoprotein hormones such as follicle-stimulating hormone (FSH), luteinizing hormone (LH), and chorionic gonadotropin. Basically, three different protocols of stimulation are used with major or minor modifications (gonadotropin-releasing hormone (GnRH) agonist (long or short protocol) and GnRH antagonist protocol). The most commonly used stimulation protocol is the long protocol; it was first described almost 30 years ago in 1984 [1]. Even though several infertility clinics apply generally the two other protocols, short and antagonist protocols were designed for distinct medical indications such as low responders or patients at high risk for ovarian hyperstimulation syndrome (OHSS) syndrome [2, 3].
Before initiating follicular growth using the long protocol, the first step after the medical examination is the downregulation of the pituitary gland by supraphysiological doses of GnRH agonists. These pharmaceutical peptides (i.e., triptorelin, ganirelix, cetrorelix, and buserelin) prevent a premature LH surge. Indeed, in almost one-fourth of all cycles, such an LH surge occurs only when gonadotropins are used [4]. Provided the menstrual cycle is regular, the initiation of GnRH agonists in the long protocol is normally between days 18 and 22 of the menstrual cycle. GNRH agonists are usually administered via a subcutaneous injection. These agonists do not quickly dissociate from the GnRH receptor and block the GnRH function by reversibly binding to the GnRH receptor of the pituitary gland (also known as competitive inhibition). As a result, the release of FSH and LH from the pituitary gland is suppressed.
This physiological state, also called downregulation, is usually confirmed by two main criteria: (1) the determination of blood hormone levels (serum estradiol <200 pmol/l) by a clinical laboratory and (2) transvaginal ultrasound (TVUS) by a physician. The pituitary downregulation should be confirmed by a hypoestrogenic state, and additionally, the endometrial thickness (ETS) should be <5 mm. Measurement of the ETS diameter performed by 3D TVUS can be a highly predictive method to determine the state of hypoestrogenism [5]. It should also be noted that an endometrial morphology examination is also necessary to determine whether the embryos can be transferred in a fresh cycle or it is advisable to cryopreserve them in order to perform a transfer in a subsequent cryo-cycle (this will be further discussed later in this chapter). Examinations of the endometrial volume and the endometrial morphology are crucial to estimate the implantation success, the risk of spontaneous abortion, or other risks.
In a subsequent step, ovarian stimulation is performed by FSH (i.e., Puregon®, Gonal-F®, Altermon®, or Bravelle®) or combinations of LH and FSH (i.e., Pergoveris®, Merional®, or Menopur®); these are administered by subcutaneous or intramuscular injections. The administration of such high, ultra-physiological doses of human FSH triggers the stimulation, growth, and retrieval of multiple follicles, thus increasing the number of mature oocytes [6]. The retrieval of good quality oocytes increases the likelihood of a high fertilization rate and an adequate number of high-quality embryos. Before initiating ovarian stimulation, an US is a prerequisite for planning the IVF therapy in detail; it can estimate the ovarian reserve, which can be done most accurately by an antral follicle count.
To date, as previously mentioned, there are many applications of US for gynecology and reproductive biology; furthermore, a variety of US equipment and techniques are available to perform US scans. However, as a matter of principle, US techniques can be differentiated by 2- or 3-dimensional techniques. Very recently, 4D US has emerged. With 4D US, only 3D data can be acquired in real-time mode.
Conventional 2D US instruments are based on cross-sectional scans, where only a single focus is visualized. In contrast, 3D US is based on a series of 2D images, received either by manual or automatic systems; the 3D image is then calculated and generated. In 3D mode, several scans are assembled into a 3-dimensional structure; therefore, the limitation of 3D US quality depends on the quality of the initial 2D imaging. Thus, it must be kept in mind that when the original 2D quality is low, there might be a loss of spatial resolution in the reconstructed 3D image also.
A 3D US examination comprises four steps: (1) data acquisition, (2) volume calculation, (3) image animation, and (4) data storage and transfer. In regard to the mode of data acquisition, US scans can be obtained either freehand, by manual movement through the region of interest (ROI), or automatically, by sweeping through the ROI. It is indisputable that the former modality is more subjective and is more susceptible to inter- and intraobserver reliability, while the latter is more standardized and accurate. However, it should be noted that 3D US needs post-processing of the received data. Data can be stored and visualized in various displays such as multi-planar with navigation through the planes or surface rendering mode. The 3D US technique allows for an easy volume calculation of the examined objects, which is one of its greatest fortitudes in contrast to 2D imaging; furthermore, the determination of the mean diameter of the analyzed object can be determined. Additionally, post-processing software allows the presentation of 3-dimensional structures of the examined object in the so-called inversion mode [7]. According to differences in density of tissues or structures, there are differences in ultrasound reflection and scattering, which is also referred as echogenicity. Normally, the degree of echogenicity can be visualized by a gray scale. Minor echogenicity is represented by black voxels, while regions of high echogenicity are pictured by white voxels. The gray-scale voxels of volume data sets can be inverted; this sometimes allows a better illustration of anechoic structures such as cysts. Inversion mode can be used for a better determination of tissue boundaries and efficient volume calculation. Some software programs also picture the captured objects in different colors and, therefore, enable a clear overview. This is needed when many objects are scanned and counted (i.e., follicles).
One of the major advantages of US is that it is a noninvasive, painless, and harmless procedure. In contrast to X-ray, US scans are not hazardous either to the patients or to the follicles; furthermore, US radiation does not impair the developmental potential of implanted embryos [8, 9]. Portable magnetic resonance tomography (MRT) instruments are now available; however, the advantage of US over other imaging techniques such as MRT is that US equipment is less expensive and easier to operate. Examination with MRT is more time-consuming than US; furthermore, it requires contrast agents. Moreover, regarding imaging and velocity, 3D Doppler sonography is the only established imaging method for ART that can display liquid flow such as blood circulation; this is accomplished by calculating the frequency shift within the analyzed sample volume. Due to these various options, Doppler sonography is applicable to a broad field of diagnostic applications.
Interestingly, the principle of 3D US is not a new innovation; the technique itself has been in existence for almost three decades [10]. This fact might be quite astonishing; however, it must be kept in mind that in the past its application was limited because of the restricted calculation and memory capacity of computer systems at that time. With the tremendous acceleration of technical advancements in this field, instruments also became available at an affordable price for medical applications. Fast computers now enable 3D ultrasound picture construction, and the Digital Imaging and Communications in Medicine (DICOM) specification has facilitated the full integration of ultrasound into the picture archiving and communication system (PACS).
Use of 3D Ultrasound of the Female Reproductive System Before and During IVF in Regard to Endometrial Receptivity
On occasion, ultrasound can help determine factors involved in female infertility. Three-dimensional TVUS allows evaluation of the pelvic organs, and it is useful for the detection of pelvic pathologies such as myomata, polyps, or cysts, which can have detrimental effects on a patient’s health, fertility, and pregnancy outcome. US aids in the identification of malformations of the uterus such as unicornuate uterus, bicornuate uterus, or uterine septae. Patients with these malformations require intense pregnancy surveillance because pregnancy loss, premature birth, and other complications are more common [11–14]. Furthermore, approximately about one-fourth of patients with recurrent pregnancy loss may have uterine anomalies [15]. The use of 3D ultrasound techniques is superior to other modalities for the detection of uterine malformations. The correct diagnosis of malformations is crucial for deciding whether to correct them i.e., by hysteroscopy or to refrain from surgical intervention.
Although 3D US can confirm the status of a woman’s reproductive system, the medical literature contains only a handful of studies in this regard. A prospective study encompassing 284 women demonstrated the reliability of 3D US for the detection of Müllerian anomalies [16]. The accuracy was verified by endoscopy, and the authors noted that the scan could be performed in a brief period of time. Their findings confirmed previous studies by other investigators [17, 18]. Furthermore, the high intra- and interobserver reliability for 3D sonography has been reported [15]. In view of this, it must be kept in mind that 2D US of the pelvic organs cannot achieve this degree of accuracy. Rosendahl et al. demonstrated this feature by comparing the true ovarian volume and the volume determined by 2D US; they reported a discrepancy of approximately 30 % [19].
Ultrasound not only allows the imaging of the major organs but also the detection of small histological abnormalities. For example, a recent study evaluated 275 consecutive women undergoing an IVF cycle in which TVUS was applied; it showed that the clinical and ongoing pregnancy rates drastically decreased when women had adenomyosis [20]. The authors pointed out that the diagnosis of adenomyosis could be promptly made with high-resolution transvaginal ultrasound. The finding that adenomyosis can impair pregnancy is also supported by a former retrospective study of 748 IVF patients who underwent a TVUS to identify possible pelvic pathology before starting IVF therapy with the GnRH antagonist protocol. The investigators found that the clinical pregnancy rate was reduced by 50 % in patients with adenomyosis, compared to women without the condition [21]. Nevertheless, these authors correctly stated that “there is no consensus regarding the impact of adenomyosis on implantation potential.” This finding is quite remarkable because the worldwide IVF success rates are still unsatisfactorily low and assisted reproduction facilities are still facing the challenge of pregnancy rate improvement. This situation might be explained by the fact that US advancements, especially the 3D techniques, have only recently appeared; thus, IVF pregnancy rates may increase in the near future.
The endometrium is the innermost glandular layer of the uterus and the location for embryo implantation. Morphology and thickness (volume) of the endometrium can be visualized by US. This technique is widely used because pathological alternations of the endometrium can drastically impair female fertility. Endometrial polyps might affect embryo implantation (depending on their size, position, and number). Therefore, detection of these polyps is relevant for IVF success. Either subsequent removal by polypectomy or the application of IVF cryo-cycles is the method of choice. Nevertheless, to date only limited data is available, and only a few studies have demonstrated the superiority of 3D techniques in the diagnosis of endometrial polyps compared to 2D US [22]. A study of 103 patients with postmenopausal bleeding revealed the advantages of 3D techniques for the diagnosis of endometrial pathologies [23]. Therefore, the application of 3D US allows a conceivably better discrimination between benign and malignant endometrial pathologies with less false-positive results; furthermore, endometrial volume calculation with 3D US was found to be superior to 2D US for the measurement of endometrial thickness. The advantage of 3D US to discriminate between different uterine anomalies was also demonstrated by two other studies [17, 18].
In addition to correctly diagnosing endometrial pathology, a second crucial point is that follicular maturation needs to be synchronized with endometrial receptivity in order to achieve and sustain a pregnancy. Endometrial morphology, thickness, and perfusion are subjected to hormonal alterations, which are reflected by a shift in morphology and function. Beginning in the 1990s, the relationship between serum estradiol levels and endometrial thickness during downregulation has been demonstrated by multiple studies; however, the first study of the new 3D US that imaged the endometrium was published by Yaman et al. [5]. They first examined endometrial volume by 3D ultrasound in the case of pituitary downregulation. They found that 3D measurements of the endometrial volume were highly accurate; however, the authors reported no additional benefits of the endometrial volume measurement. The endometrial receptivity for embryo implantation is characterized by certain morphological and biochemical alternations and is also called the “window of implantation.” These alternations include an increase in the proliferation and thickness of the endometrium.
According to the application of high, supraphysiological hormone dosages during stimulation, it is sometimes difficult to simultaneously achieve both perfect development of multiple follicles and optimal endometrium buildup. Therefore, characteristics of the human endometrium, including thickness (volume), morphology, endometrial blood flow, and vascularization, can be readily and noninvasively monitored by US monitoring. Nevertheless, a direct correlation between endometrium buildup and implantation rates as well as pregnancy rates is still to be confirmed. Although endometrial patterns have been reported to correlate with endometrial stages for the past 15 years [24], large discrepancies have been reported in regard to correlations between the foregoing and endometrial thickness necessary for successful implantation. Some studies have reported correlations between endometrial thickness, endometrial morphology, and pregnancy outcome; however, others do not address those factors [25, 26].
Currently, there is still no consensus regarding the endometrial thickness and endometrial volume necessary for successful implantation. However, most physicians agree that a certain degree of buildup is crucial. Several studies have suggested that pregnancy rates dramatically decrease when the endometrial thickness is <5–7 mm or the endometrial volume is <2.5 or 1 ml, respectively [5, 27–30].
In a 2001 study, it was reported that implantation is unlikely when the endometrial thickness is <5 mm [31]. Other investigators report no correlation of endometrial thickness and patterns to implantation rates [32]. The reason for these discrepancies might be based on the application of different stimulation protocols, the various sonographic instruments and techniques (primarily 2D US) employed, and especially on the different patient subgroups analyzed. Therefore, the outcome cannot be directly compared. It must be noted, however, that there are application limitations of 2D US for this procedure. Measuring the endometrial volume by this technique yields limited accuracy and requires significant time. Even for endometrial thickness, there is currently no general consensus of a cutoff value [30]. However, some authors have proposed a minimum of 5–8 mm. Despite this situation, there might be a higher consensus to recommend embryo cryopreservation in cases of thin and non-trilaminar endometrium because the likelihood of implantation is low with this finding.
One study directly compared 2D and 3D US applications in US scans of the endometrium [30]; in addition, several studies have reported the advantages of 3D US in analyzing the endometrium. The major advantage of 3D US over 2D US is that it is a simple method of measuring the endometrial volume. The morphology of the endometrium can be readily determined when it appears as trilaminar (with a central echogenic line, inner hypoechoic regions, and hyperechogenic outer walls) or non-trilaminar (as a homogenous layer). Additionally the advantage of 3D US for calculation of the endometrial volume is its low deviation of inter- and intraobserver reliability [33, 34]. However, these parameters might not in themselves be adequate for the prediction of successful implantation [35, 36]; thus, other factors that can influence the success rate have to be considered.
US Monitoring of Polycystic Ovary Syndrome (PCOS) Patients
Polycystic ovary syndrome (PCOS) is one of the most common endocrine disorders impairing female fertility; it has been reported to occur in about 20 % of the general female population and in up to 50 % of women undergoing IVF therapy [37–39].
A number of symptoms have been associated with PCOS that are subject to controversy; however, four defined criteria have broad acceptance as symptomatic of the disease: (1) chronic irregular ovulation or anovulation (therefore oligomenorrhea, might be an early clinical symptom for PCOS), (2) hyperandrogenism diagnosed clinically (expressed by alopecia, hirsutism, and/or acne) or by laboratory findings (serum testosterone >1.4 nmol/l), and (3) the exclusion of other endocrine disorders. The fourth criterion can only be defined by ultrasound examination. Polycystic ovaries are defined as those which contain ten or more cysts with a maximum diameter of 10 mm arranged either peripherally around a dense core of stroma and/or scattered throughout an increased amount of stroma [40]. Moreover, PCOS patients are more likely to have larger ovarian volumes (>10 cm3) and ovarian stroma with increased volume and increased numbers of antral follicles (12 or more follicles, according to the Rotterdam criteria) [41]. Therefore, US scans play a crucial role in diagnosing this disease. The new automated 3D US techniques facilitate the exclusion of a false-positive PCOS diagnosis and reflect pathophysiological changes in these patients in a more accurate manner. An early detection of PCOS is highly recommended for women undergoing IVF treatment, due to the elevated risk for OHSS. Unfortunately, because 3D ultrasound is a relatively new imaging modality, the Rotterdam criteria only take 2D US sonography into account.
At present, only a few studies have evaluated the use of 3D US for PCOS patients [42]; furthermore, to date, only one study has addressed automated 3D US (SONO-AVC) [43]. In a retrospective cohort study, Allemand et al. analyzed 29 normoandrogenic, ovulatory women with tubal or male factor infertility and 10 PCOS women with chronic anovulation and clinical or biochemical hyperandrogenism [42]. Mean follicle number/ovary (FNPO) as well as the maximal number of follicles in a single sonographic plane (FSSP) was determined by 3D TVUS; simultaneously, the ovarian volume was determined by 2D TVUS. Interestingly, the authors postulated a considerably higher threshold of antral follicles (20 or more) for PCOS patients, which is a considerably higher threshold than that of the Rotterdam criteria. The authors explained this discrepancy by the identification of more follicles by 3D US. The weakness of this study is the small number of patients, the missing direct comparison to 2D US, and the low sensitivity (true positive rate) using receiver operating characteristics (ROCs). According to most publications dealing with 3D US in PCOS patients, there is a broad agreement about increased ovarian volume of polycystic ovaries [43–48]. Nevertheless there is still disagreement regarding ovarian vascularity, stromal changes, and cutoff values for antral follicles [41, 44, 48, 49].
In most PCOS studies where 3D techniques were applied, the numbers of analyzed patients were low; therefore, further studies are necessary. However, it should be noted that a major advantage of 3D US imaging of female pelvic organs when a hydrosalpinx or cyst is noted is the aforementioned inversion mode. Such structures might not be displayed by a single slice; however, they may be recognized at full scale by viewing multiple planes. In 2006, Benaceraf [50] noted that the transformation of voxels within a volume to echogenic structures and voxels of solid structures to radiolucent images is one method that can facilitate the acquisition of the total volume of a hydrosalpinx or cyst without being hampered by the gray-scale portion of the image.
Ultrasound in Estimation of the Ovarian Reserve
Before initiating an ART cycle, it is crucial to estimate the ovarian response to stimulation. The latest time for this estimation should be the cycle prior controlled ovarian hyperstimulation (COH) cycle; moreover, it is the last possible opportunity to determine any pelvic organ abnormalities before stimulation.
The ovaries contain several subtypes of follicles: the primordial follicles (≤0.05 mm diameter), primary follicles, secondary follicles, pre-antral follicles, and antral follicles (>2 mm diameter). However, only a small number of ovarian follicles are highly responsive to FSH [51]. The number of these so-called antral (or Graafian) follicles represents the “for stimulation selectable follicles.” The antral follicle count (AFC) reflects the ovarian reserve and is predictive of the IVF outcome in regard to the number of yielded oocytes in response to hormonal stimulation [45, 52, 53]. This allows the classification from very low (≤6) to high responding patients (≥12 up to 30), the calculation of risks for ovarian hyperstimulation syndrome or polycystic ovaries (PCO), and, most importantly, the adaption of gonadotropin dosage during the stimulation schedule [41, 54–57]. Inappropriate stimulation with subsequent excessive response increases the risk of OHSS; however, inadequate stimulation might result in cancellation of an IVF cycle. It should also be noted that antral follicles of different sizes respond differently to gonadotropin stimulation. Furthermore, follicles that are >9 mm are more likely to become atretic (apoptosis-induced degeneration and the following reabsorption process) [58]. The accurate assessment of the ovarian reserve is therefore a prerequisite for planning the optimal therapy for each patient and an individualized management protocol. Various clinical, endocrinologic, and other parameters (i.e., estradiol, the basal FSH, the declining levels of anti-Müllerian hormone (AMH), or inhibin B levels) have been suggested as predictive indicators for ovarian reserve and COH response [59–64]; however, their validity is extremely limited, and hormonal assays are currently expensive [65, 66]. Additionally, there are several markers that significantly correlate with the ovarian reserve such as age or ovarian volume; however, these markers are less predictive [67, 68]. It is widely accepted that age is strongly associated with ovarian reserve; however, there is a high variability even among women of the same age [69].
The estimation of antral follicle count and antral follicle size performed by TVUS is currently the most reliable method and gives the best correlation with retrieved oocytes [64, 68, 70, 71]; moreover, it is easy to perform and is noninvasive. Therefore, the AFC determined by ultrasound, notably by 3D techniques, is the best predictor for poor ovarian response, OHSS, oocytes collected, and live birth rates [72]. It should be noted that it is imperative to distinguish between the total antral follicle count (TAFC), including follicles >6 mm in diameter and the number of small antral follicles which better reflects the ovarian reserve [73] and predicts the COH response [74].
AFC can even be obtained on day 2 of the menstrual cycle [71]; however, the best time for estimation of antral follicles is the middle to late phase of folliculogenesis. Currently, the AFC is determined by 2D as well as 3D US, with predominance of the 2-dimensional techniques. In regard to 3D ultrasound, we must distinguish between manual and automatic systems (such as Voluson e in combination with SONO-AVC software); this will be discussed later in this chapter. Nevertheless, there is the question regarding the reliability of AFC determined by different US techniques as well as which current methods are the best. Of note, only a handful of studies compare the accuracy of ultrasound in regard to AFC and inter- /intraobserver reliability. Broekmans et al. described the value of AFC determined by AVC needed for the improvement of standardization and presented a tutorial on clinical and technical requirements [52].
Standard AFC assessment was, and still is, performed primarily with 2D US imaging. Although this modality might be sufficient in some cases, there might be some uncertainties and disadvantages. One disadvantage of 2D involves inter- and intraobserver reproducibility. Scheffer et al. [75] compared healthy volunteers with proven fertility to patients visiting an infertility clinic. For each patient, 2D or 3D TVS was conducted for AFC (2–10 mm), and interobserver reliability was calculated. Both techniques were adequate when only a few follicles were present; however, when higher AFCs occurred, the reproducibility decreased with the 2D technique. In addition to this report, studies by the group of Raine-Fenning [76–80] demonstrated an improvement of interobserver/intraobserver reliability by the application of 3D methods (in particular by automated systems, such as SONO-AVC). One of the disadvantages of manual 3D US AVC is the greater examination time that occurred in a study comprising 55 women [78]. Nevertheless, this finding was contradicted by the same researchers in a second study, in which they measured the examination time instead of including time for post-processing of the US scans [79].
Interestingly, in several studies the TAFC was found to differ between 2D and 3D techniques [78, 79]. This might be explained by the fact that when the follicle number increases, the accuracy of 2D techniques decreases because of losing track of counted follicles and multiple counting of the same follicles [81, 82]. In conclusion AFC is highly predictive for the ovarian reserve and strongly associated with the serum AMH level [74]. The estimation of the ovarian response by US is simple and noninvasive; thus, it is an advisable procedure. The reliability of this method is probably increased with 3D instruments.
Follicle Tracking During Controlled Ovarian Hyperstimulation
The consistent aim of controlled ovarian hyperstimulation during an IVF is to obtain the maximum number of mature and good quality oocytes. This increases the chance for having an adequate number of high-quality embryos, which rises the likelihood of achieving a viable pregnancy. Therefore, oocyte maturity is a critical factor. The cytoplasmic as well as nuclear maturity, defined as oocytes that have completed their first meiotic division and reside in metaphase II (MII), is required for a high fertilization rate and optimal embryo development. According to the classical IVF protocols, GnRH analogues and hCG are administered to trigger the ovulation process when the dominant follicle reaches a diameter between 18 and 20 mm or the follicle volume has reached 3–4 ml [83]. The timing for hCG administration is the most critical step of an IVF cycle. During COH, follicle growth occurs at different rates; thus, follicles of varying sizes are present. Therefore, the best method to obtain a maximal yield of MII oocytes is to obtain a maximal number of follicles of large diameter or volume. Nevertheless it should be also considered that in the case of an extended stimulation period, follicles might become atretic and oocyte quality might be markedly compromised. In addition, a possible danger of OHSS and chromosomal abnormalities in the oocytes due to excessive hormonal stimulation is discussed. Currently, follicle growth is monitored with serial ultrasound examinations at regular intervals. In these examinations, the total number of follicles in each ovary is counted, and each follicle is measured by ultrasound. Thus, it is likely that these regular examination intervals can result in a maximal error rate for intra- and interobserver reliability.
Ovarian size, follicle growth rate, and follicular volume were considered to be important indicators of oocyte maturity and regarded to be measurements of therapy success [84]. This was not always the case. A main stimulator of follicle growth is 17β-estradiol (E2); it was and currently is used as indicator of follicle growth during COH. Quigley et al. [85] reported that they administered hCG on the sixth day of E2 rise. They stated that “the use of E2-rise days proved to be a simple, successful technique for the timing of hCG administration in an IVF treatment program.” This viewpoint was also assumed by other researchers [86]. Nevertheless, follicle growth and oocyte maturation are regulated by protein and peptide hormones, growth factors, steroids, and many other factors; thus, these factors must be take into account when performing IVF. Moreover, one must keep in mind that only the overall E2 value can be measured. However, this quantitative hormone determination does not take into account the E2 production of the individual follicles.
Casper et al. [87] pointed out the problems occurring with a spontaneous LH surge during mid-cycle and the possibility of ovulation, thus demonstrating the incalculability of follicle maturation monitoring by E2. In 1985, Nilsson et al. [88] postulated that ultrasound scanning should be the sole index of monitoring follicle maturity. Actually, growth rate and follicular volume are the only applicable factors, because the estimation of hormonal levels for follicular maturation such as the serum E2 value alone for prediction of follicular maturity was reported to be unsatisfactory [89]. US technology is the best modality to determine an accurate strategy of ovulation and to determine the date of OPU.
Human chorionic gonadotropin mimics the endogenous LH surge and induces the nuclear maturation and the germinal vesicle breakdown. For decades, it has been used as the universal trigger of final oocyte maturation. Therefore, the state of follicular maturity reflects the developmental potential of oocytes and embryos as well as the ART outcome.
The induction of multiple follicle maturation requires the determination of the best time point for hCG administration to induce final oocyte maturation. This time point was and still is determined by US. In a retrospective study of 2,429 oocytes from 215 patients, the fertilization rate of all oocytes showed a positive linear correlation with increasing follicle diameter regardless of the morphological type of oocyte-cumulus-corona complex [90].
Although 3D US systems had been developed and patented at the end of the 1980s [91], there is still a broad debate on the benefits of 3D US in follicle monitoring during COH. Moreover, there are still no uniform standards for follicle monitoring via US in ART. The conventional two-dimensional (2D) transvaginal ultrasound (US) techniques are used in many centers; however, they completely neglect the third follicular diameter (z-diameter). Therefore, the follicular volume is calculated by the formula for spherical volume V = (4/3) r 3 π. Some observers rely on a single “best” estimate, while others use one or more planes. Although this might hold more or less true for follicles of a natural cycle, the induction of multiple follicular growth during hormonal stimulation results in undesirable side effects. Of note, especially when multiple follicles are present, the follicles almost never exhibit a spherical shape, which can result in an overestimation of the mean follicular diameter. Almost two decades ago, it was demonstrated by a prospective clinical trial that the mean diameter estimated by 2D US reflects the follicle volume if the follicles have a round or polygonal shape. In contrast, for elliptical follicles, the volumes could not be predicted and were over- or underestimated; this was shown by a prospective clinical study comprised of 14 patients and 96 follicles [84]. This was also observed in a prospective clinical study of Kyei-Mensah and colleagues [92], where they demonstrated that the follicle volume measured by 3D US reflects a more accurate follicular volume than 2D measurements. With 3D US, they found discrepancies of up to 1 ml of the true volume. In contrast, with 2D US an overestimation of 3.5 ml or underestimation of 2.5 ml of the true volume was observed; thus, 2D US is inferior to 3D for follicular volume measurement. According to our own observations, the follicle size is typically underestimated for small follicles and overestimated for the larger ones. The total number of follicles cannot be determined the classical 2D US. In cases of ovarian hyperstimulation syndrome, the stimulation response is likely to be high, which means an antral follicle count >30. In these cases, it is often difficult to ensure that every follicle is accounted for and often the qualification regarding the follicle size and quantification is unreliable.
Additionally, follicular asynchrony occurs, which means that the stimulated follicles are a heterogeneous group of varying size with different degrees of maturity. This might be one reason that E2 values are not predictable as they do not, as aforementioned, reflect the individual follicles but overall follicle maturity [93]. Even though there might be broad agreement that follicle size and oocyte maturity correlate with the IVF outcome, there is a considerable dissent when to trigger final oocyte maturation prior to oocyte pickup and what size the leading or the three biggest follicles should be when hCG is administered. This might be due to the small number of studies. Moreover, the available data is conflicting. Although several studies have suggested that human oocytes derived from larger follicles have greater potential than oocytes originating from smaller follicles, the correlation of oocyte competence with follicular size following COH has not been fully evaluated [94
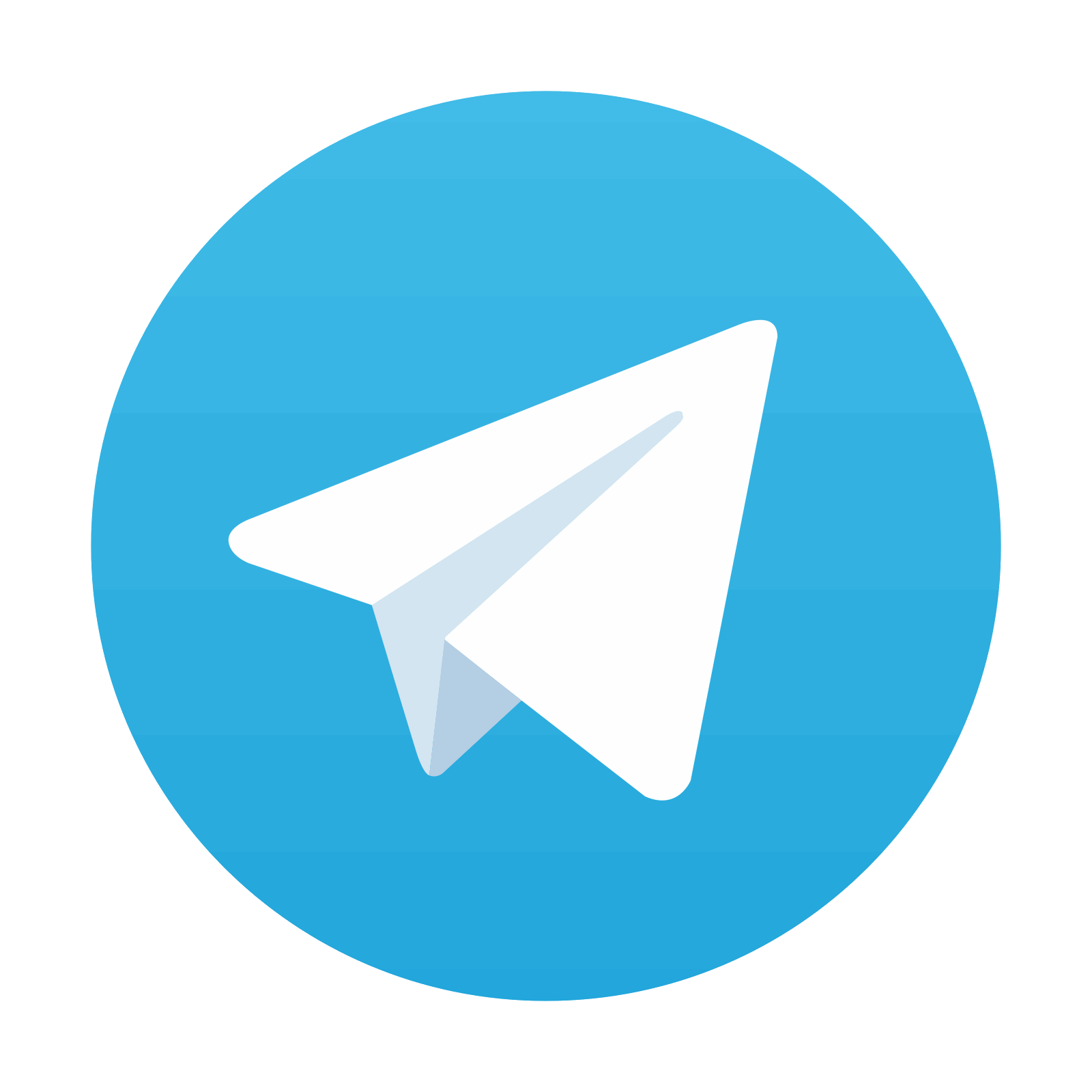
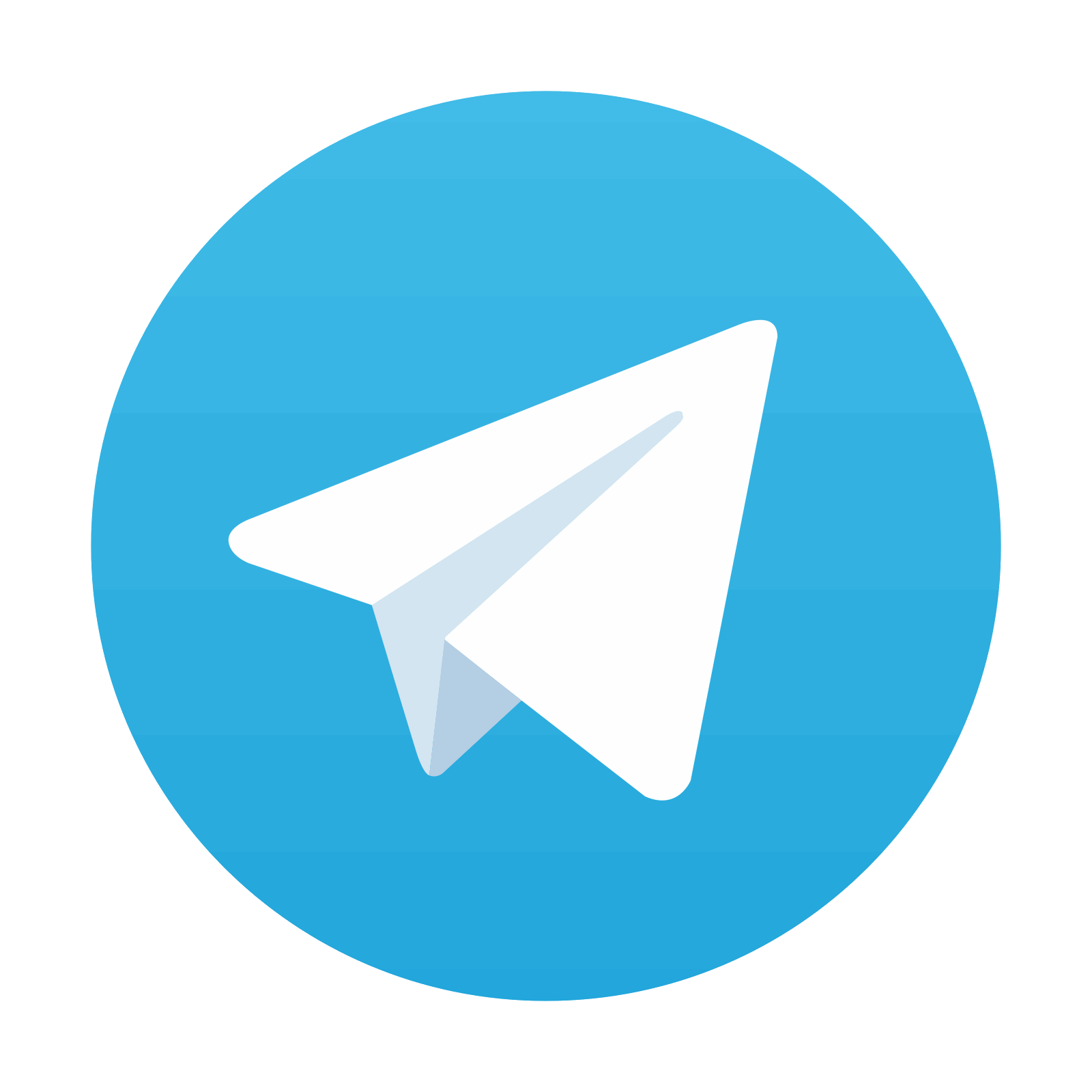
Stay updated, free articles. Join our Telegram channel

Full access? Get Clinical Tree
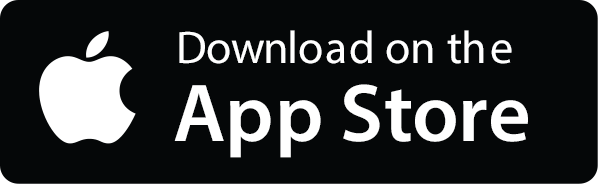
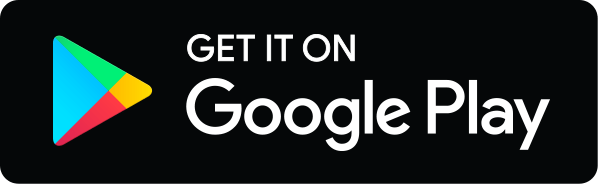