6 Skeletal Scintigraphy
Three-phase skeletal scintigraphy (TPS) of the hand makes it possible to evaluate the regional circulation and bone metabolism in a single examination. Pathologic factors that can exert an influence are osseous hyperemia, ischemia, callus development following fracture, inflammation of the bones and joints, inactivity osteoporosis, and trophic disorders. Skeletal scintigraphy is primarily employed to exclude pathologic osseous processes, to identify fractures, and to provide further differentiation when radiographic results are inconclusive. In such cases, scintigraphy often plays a decisive role.
Physical-Technical Foundations
In comparison to radiologic procedures, diagnosis in nuclear medicine is more functionally oriented. It is based on the tracer method. A radioactive substance in a pharmacologically ineffective dose is administered to the metabolic system of interest. The spatial distribution of the radiopharmaceutical substance can be measured at the patient’s body surface as a result of its emission of gamma radiation. At present the most commonly used isotope is technetium-99m (99mTc), which, depending on the clinical objective, is linked to various carrier substances. It is obtained from a molybdenum-99 generator in a technically uncomplicated process, so that it is routinely available. Technetium-99m gives off monoenergetic gamma radiation (electromagnetic radiation) with an energy of 140.5 keV. Owing to its brief half-life of 6.03 hours and lack of corpuscular radiation, this isotope has ideal characteristics with regard to radiation exposure as well as imaging quality. The current standard instrument is the digital wide-field gamma camera with an effective field of view of about 40 cm × 55cm.
Three-phase scintigraphy demonstrates arteriovenous circulation, the early distribution phase in tissue, and the late distribution of the tracer in the skeletal system. The standard examination protocol is given in Table 6.1 .
Evaluation and documentation of data can be carried out in different ways ( Fig. 6.1 ). For quantification, the region-of-interest technique (ROI technique) is generally used. Although the ROI areas should be generously allotted for the blood circulation curves (Fig. 6.1b, c), in the late scintigram the area of pathologic uptake should be kept small (Fig. 6.1d) so as not to falsify the quotients by including normal tissue. In a very small ROI (like the carpal bones), it can be useful to set a considerably larger reference ROI, since a low counting rate in few pixels in normal bone can lead to statistical problems.
The reproducibility of results is good; the coefficient of variation is less than 5%. In contrast to radiographic diagnosis, plaster casts up to 1 cm in thickness do not influence optical interpretation and quantitative assessment with scintigraphy.
Positioning of the hand:
|
Bolus injection of 99mTc-labeled diphosphonate
|
First phase: Arteriovenous circulation
|
Second phase: Early-phase scintigram
|
Third phase: Late-phase scintigram
|
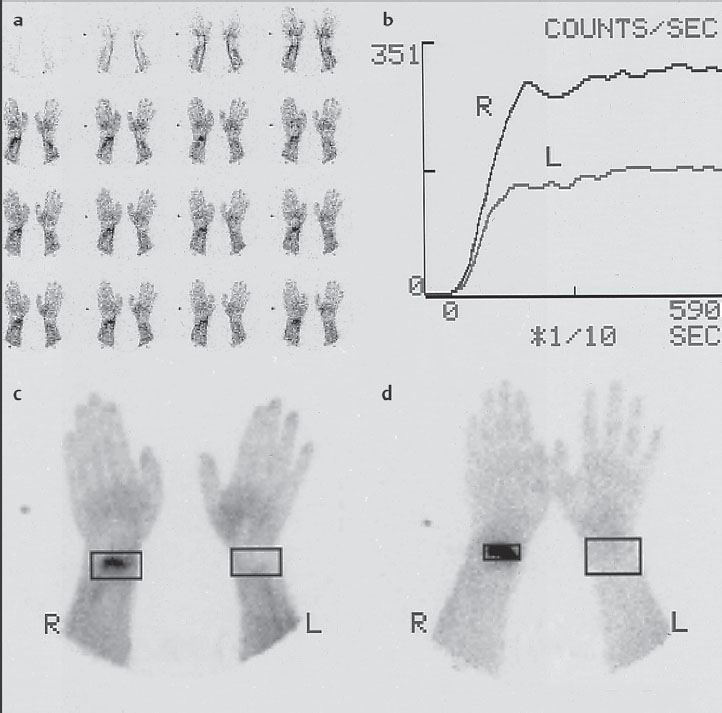
Biological Foundations
Knowledge of the local differences in bone perfusion is important for the interpretation of skeletal scintigrams. A well-developed network of vascular collaterals makes the blood supply in periarticular areas considerably higher than that in the shafts of tubular bones. Their blood supply comes mainly from the bone marrow and is much more likely to be disturbed by dislocated fractures.
Stage I: Inflammation
|
Stage II: Repair
|
Stage III: Remodeling
|
As a result of the differences in perfusion, 8–10 days after a trauma, for instance, in periarticular areas hyperemia is nearly always present with a relatively higher accumulation of the isotope. A fracture of the shaft also causes reactive hyperemia in the surrounding soft tissues, but not in the affected parts of the bone. Similar mechanisms lead to intense accumulation of the tracer in inflammatory and active degenerative joint diseases.
Furthermore, reactive bone formation after a fracture causes tracer accumulation in scintigrams. Fracture healing can be summarized in three stages ( Table 6.2 ).
According to the transformation law, a reconstruction process also takes place in the final callus.
In the tracer technique, osteotropic radiopharmaceuticals are transported into the osseous metabolism. The scintigraphic image of accumulated tracer in the bone changes according to the different pathophysiologic factors prevailing at different stages of fracture healing.
Finally, the fundamental principles underlying tracer uptake under normal and abnormal conditions of the bone have not been entirely clarified. Arnold’s concept appears suitable to correlate theoretical concepts with empirical clinical data ( Table 6.2 ). A model consisting of two bony compartments, each of which is connected with the blood compartment, offers the following explanation:
One compartment represents weak binding sites on the crystalline surface of the quiescent bone. Tracer clearance from the blood onto the weak binding sites correlates with the intensity of blood flow to the bone.
The second compartment represents strong binding sites, which are formed by amorphous calcium phosphate in newly formed lamellar bone. Tracer clearance in the strong binding sites correlates with new bone formation. The increased tracer clearance during new bone formation is based on an increase in the extraction efficiency. This increase is proportional to the newly-formed lamellar bone, whether callus formation, an osseous tumor, or osteomyelitis. Differentiation can be achieved only with the aid of other imaging procedures and the clinical findings.
Because of the exposure of the patient to ionizing radiation, skeletal scintigraphy demands an appropriate indication. Table 6.3 shows gonadal exposure in comparison to radiographic diagnostic procedures.
Table 6.4 lists the radiation doses for different organs, demonstrating that the urinary bladder is the critical organ because of elimination of the radiopharmaceutical in the urine. Radiation exposure can be reduced by sufficient diuresis and frequent urination.
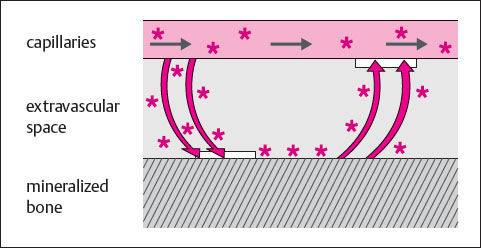
Skeletal scintigraphy, like other nuclear medicine examinations, is contraindicated during pregnancy and lactation. Lactation constitutes only a relative contraindication, though, since nursing can be resumed without danger two days after such examinations.
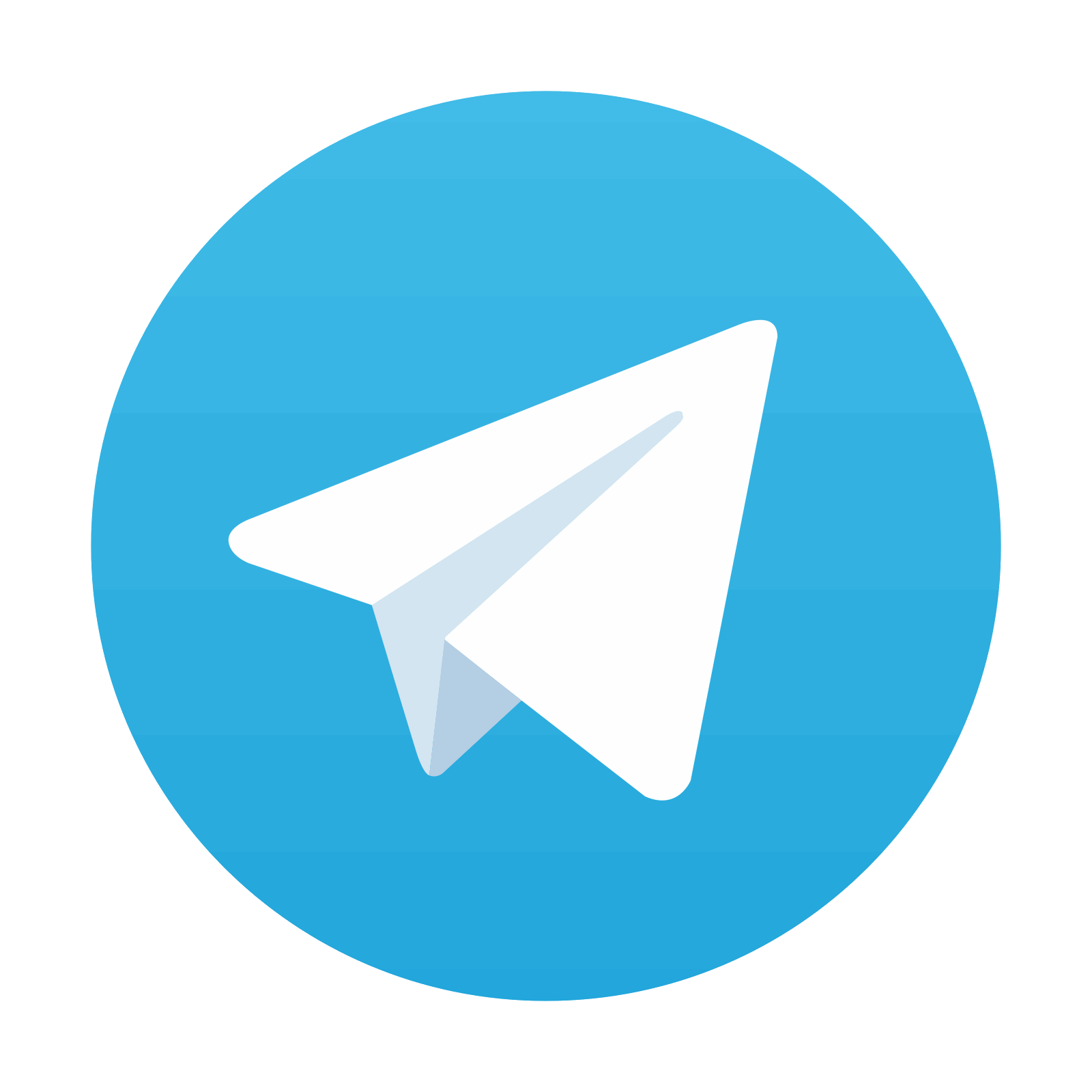
Stay updated, free articles. Join our Telegram channel

Full access? Get Clinical Tree
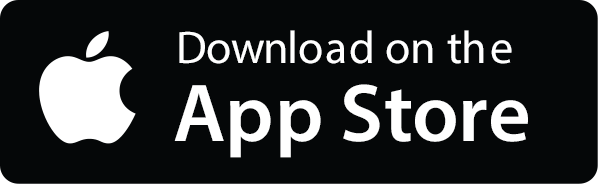
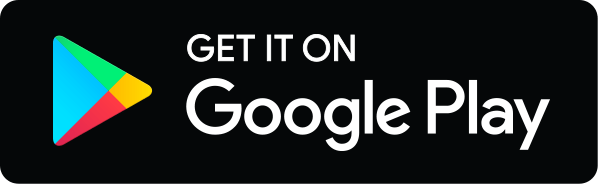