7 Ultrasonography
High-frequency probes (7.5–15 MHz) provide excellent imaging of the soft tissues of the hand, especially in the carpal tunnel. New developments, such as tissue-harmonic imaging, computed ultrasonography (US), extended-view techniques, and three-dimensional US, have considerably increased imaging possibilities in the small parts of the hand. Present diagnostic indications are: (1) injuries to the tendons, collateral and anular ligaments, (2) pathologic processes of the tendon sheaths, (3) nerve-compression syndromes, (4) confirmation of joint effusion and inflammatory joint diseases, (5) detection and staging of soft-tissue tumors and other space-occupying lesions, and (6) evaluation of vascular pathologies with color-coded duplex US. Although considerably limited in visualization of bone abnormalities, US offers the advantages of wide availability and ability to record functional movement patterns.
Physical Principle
Definition: Ultrasonic waves are periodic oscillations of particles around their resting positions that spread spatially in elastic waves. Medical US uses acoustic frequencies from 1 to 15 MHz that are beyond the range of human hearing, which is about 1–20 kHz. The conduction velocity of ultrasonic waves depends on the density of the material being examined: in water it is 1490 m/sec, in parenchymal organs (liver, spleen, kidneys, etc.) and muscle about 1580 m/sec, and in bone 3360 m/sec.
Acoustic impedance and interface reactions: The decisive physical property for imaging is the acoustic impedance, since interfaces between two adjacent tissues with different conductivity can change direction and quality of the wave. Physically, the acoustic impedance Z is the product of the density of the material σ and the acoustic-wave velocity c (Z = σ×c). At acoustic interfaces, reflection, refraction, diffraction, diffusion, and absorption take place ( Fig. 7.1 ).
Since only the portion of acoustic waves reflected back to the probe without deviation from the plane of incidence can be used for diagnosis, the energy at the acoustic-wave receiver is less than 1% of the transmitted energy.
Producing, transmitting, and receiving: The production of acousticwaves is achieved by means of the piezoelectric effect. The energy of a high-frequency electric alternating current applied to a quartz crystal causes rhythmic changes in the crystal volume that are transmitted into the surroundings in the form of acoustic ultrasonic waves. The reception of acoustic waves functions in reverse as a “negative piezoelectric effect.” The energy of an acoustic wave (acoustic wave pressure) is taken up by the quartz crystal, causing a change in the electric charge on the surface. In ultrasonographic imaging, these electric impulses are fed into a computer system, which, with the aid of a mathematical algorithm, computes scans in real time.

B-scan Ultrasonography
Image origin: In B-scan procedures (B for brightness), two-dimensional scans are produced in real-time mode and depicted as an image in various shades of gray corresponding to the wave intensity. A US probe contains numerous crystals that are arranged next to one another and alternately serve as transmitter and receiver. In the echo-impulse procedure, short wave impulses lasting 0.000001 second are transmitted in rapid succession. In comparison, the reception time for the reflected echo is very long: the transmission time constitutes only 0.1%, and the reception time 99.9% of the total time required to produce an image.
The image matrix is determined by the lateral and axial position encoding, as well as by the amplitude of the reflected acousticwaves. Lateral position encoding is achieved by identifying the receiving piezoelectric crystal. For depth localization (axial position encoding), the echo delay (sum of the transmission and reception times) correlates with the position of the point (ultrasound reflection) on the monitor. This time-position calculation causes brief echo-delay times to appear close to the probe in the upper portion of the image, whereas long echo-delay times are represented far from the probe in the lower section of the image. The echo intensity is determined by the amplitude of the acoustic wave. High amplitudes produce a bright reflex point, and low amplitudes produce a dark point.
Depth compensation: The transmitted acoustic waves weaken with increasing distance within the body (i.e., the wave amplitude decreases), and the reflected echo energy becomes correspondingly weaker. The reduction in intensity increases exponentially with the depth of penetration. To achieve diagnostically assessable ultrasonographic information, compensation for the signal far from the probe must be made in the form of electronic enhancement of the echo signal at the receiver site ( Fig. 7.2 ). Artificial brightness enhancement (DGC = depth gain compensation) of echoes received from deep tissue layers can be performed automatically or manually by the examiner.
Transducer technology: The probe converts electrical energy into mechanical vibrations and vice versa by means of piezoelectric crystals. The form of the ultrasonic beam is essentially determined by the probe design. Mechanical probes use oscillating or rotating transducers with a sector-shaped acoustic beam or anular-array constructions with ultrasonic elements arranged in concentric circles. Electronic-array probes have numerous ultrasonic elements arranged in a plane, and these elements can be controlled electronically. A linear or curved array of the transducer surface produces rectangular or sector-shaped acoustic fields. Sector scanning is also possible with phased-array probes in which the form of the sound beam can be achieved through time-offset excitation of the ultrasonic elements.

Doppler Ultrasonography and Color-coded Doppler Ultrasonography
The Doppler effect: If sound waves are reflected onto a moving interface (as are, for example, erythrocytes in flowing blood), the frequency increases when the interface is approaching the probe and decreases when it is retreating from the sound source. When the velocity of the reflecting interface is considerably lower than that of the sound waves, the difference between the transmitting and receiving frequencies is where ƒ is the transmission frequency, c is the sound velocity, v is the velocity of the interface, and α is the angle between the movement vector of the reflecting structure and the effective direction of the ultrasonic beam. At a sound transmission frequency of 3 MHz and a sound velocity of 1500 m/sec, one can assume, for example, from a Doppler shift ƒ D of 4 kHz, a blood-flow velocity v of 1 m/sec.
The continuous-wave (CW) technique is the simplest method of recording Doppler spectral curves and is based on the continuous transmission of ultrasound waves in the body. The probes have separate ultrasonic elements for transmitting and receiving sound waves.
Pulsed Doppler (PD) techniques: Selection of an electronic window for the reception of reflected sound waves makes possible delay-time-dependent depth selection; that is, the examiner can arbitrarily choose a Doppler sample volume along the ultrasonic beam. The pulse-repetition frequency is then determined by the required depth penetration and the maximal measurable flow velocity. Both methods have the disadvantage that the measurement of the Doppler spectrum must occur without imaging support.
Duplex US combines B-scan technique with PD to achieve an image-supported choice of the Doppler sample volume. While the scan movements of the transducer must be mechanically held stationary in the desired position to enable recording of the Doppler signal, with electronic array probes a nearly simultaneous recording of the B-image and the Doppler spectral curve is possible.
Color-coded duplex US uses real-time gray-scale images over which two-dimensional images showing color-coded blood flow can be superimposed. The image can be either frequency-coded or amplitudecoded (power Doppler). Although the frequency-coded image contains information about velocity and direction of blood flow, the power Doppler mode, which does not depend on the ultrasonic angle, offers optimal visualization of the vascular architecture.
Ultrasonographic contrast agents based on microbubbles, i.e., microscopically small gas bubbles ranging in size from 2 to 8 μm, present one possibility for enhancing the US signal. This characteristic is based on the nearly complete reflection of ultrasonic waves from their surfaces and, even more, on their resonance. The phenomenon can be used in the form of second-harmonic imaging, which employs only the overtones, i.e., ultrasonic waves with a frequency double that of the transmitted frequency, resulting in an improved signal-to-noise ratio. Unlike contrast-enhanced techniques in angiography, CT, and MRI, duplex US cannot provide plain and echo-contrast-enhanced images of the capillary bed in normal and abnormal tissues, as the flow velocity in the capillaries is too slow for this purpose. One possibility for overcoming this limitation involves imaging by bursting the gas bubbles with an ultrasonic beam of high-amplitude echoes (stimulated acoustic emission, SAE).
Special Prerequisites for Ultrasonographic Examination of the Small Parts of the Hand
Because of their anatomic characteristics, examination of the soft tissues of the hand places especially high demands on the spatial and contrast resolution of modern ultrasonographic equipment.
Spatial resolution: Spatial limiting resolution, i.e., the smallest size of structures that can be differentiated from one another, is influenced by various factors in US imaging. The resolution in the z direction (axial resolution) depends directly on the pulse duration and, thereby, on the frequency of the transmitted sound waves. Simultaneously, the depth penetration decreases with increasing transmission frequency because the attenuation of the sound waves through absorption, refraction, and scatter is directly proportional to the sound-wave frequency. The resolution in the y direction (lateral resolution) and in the x direction (slice-thickness resolution) is determined by the focusing of the acoustic beam and the position in the acoustic field.
Wide-band frequency probes operate with a wide frequency spectrum, e.g., between 5 MHz and 12 MHz. Whereas conventional probes with a single frequency band always represent a compromise between resolution and depth penetration, state-of-the-art probes combine high axial resolution, good depth penetration, and homogeneity of the B-mode image.
Focusing of the acoustic beam at all depths is a prerequisite for high lateral resolution and reduction of slice thickness artifacts. With mechanical anular-array probes, the exact electronic focus of the transmitted acoustic beam, as well as a dynamic focus of the reflected acoustic beam, can be selected to provide excellent conditions for ultrasonographic examination of the small parts of the hand. Focusing the electronic array probes is technically challenging, and only the most advanced instruments have solved the problem. Multiarray probes consist of acoustic elements arranged in two planes that can be selectively activated to achieve electronic focusing in the x and y directions. Moreover, with modern instruments numerous focus zones can be chosen without a significant reduction in image calculation.
Contrast resolution and the signal-to-noise ratio, along with the frequency spectrum and the focusing of the probe, are strongly influenced by the acoustic and electrical impedance of the acoustic elements, and also by the acoustic characteristics of the tissue being examined. Absorption, diffraction, and refraction of the acousticwaves at acoustic interfaces, as well as reverberation artifacts, lead to a signal-to-noise ratio that worsens with depth. In contrast, the amplitude of overtones of the reflected acoustic waves increases with increasing distance from the skin. Modern wideband probes can selectively receive these overtone acoustic waves, resulting in a significant reduction in image noise (tissue harmonic imaging). Another new possibility for improving the contrast-noise ratio and reducing artifacts is offered by real-time compound imaging (computed US), in which B-scan images from different acoustic directions are combined in real time into a two-dimensional scan. Further improvements in contrast resolution are achieved in modern instruments by means of improved signal enhancement, higher dynamic range, and complex signal processing.
Extended field of view: The limited field of view of probes used in small-part US examinations makes it difficult to assess muscles, tendons, nerves, and blood vessels, which have to be followed along their entire course over long distances. The field of view can be enlarged by computing a compound image composed of numerous slabs of images achieved by moving the probe over the region of interest. The complex correction algorithms for the translational and rotational movements during imaging require a separate processor.
Three-dimensional US: Three-dimensional imaging with image reconstruction in any plane (3D US) provides better visualization of anatomic structures, three-dimensional reconstruction of surfaces and vascular structures, and exact measurement of the volume of space-occupying lesions. Systems available today employ either probes with mechanic volumetric scanning, conventional two-dimensional probes with acoustic or magnetic registration of the probe position during scanning, or electronic matrix probes, which can directly measure volume because of their longitudinal and transverse arrangement of acoustic elements. Three-dimensional US and the extended-fieldof- view technique represent promising methods for improved ultrasonographic assessment of the complex anatomy and pathology of the hand.
The technical specifications shown in Table 7.1 must be fulfilled for examination of the small parts of the hand.
|
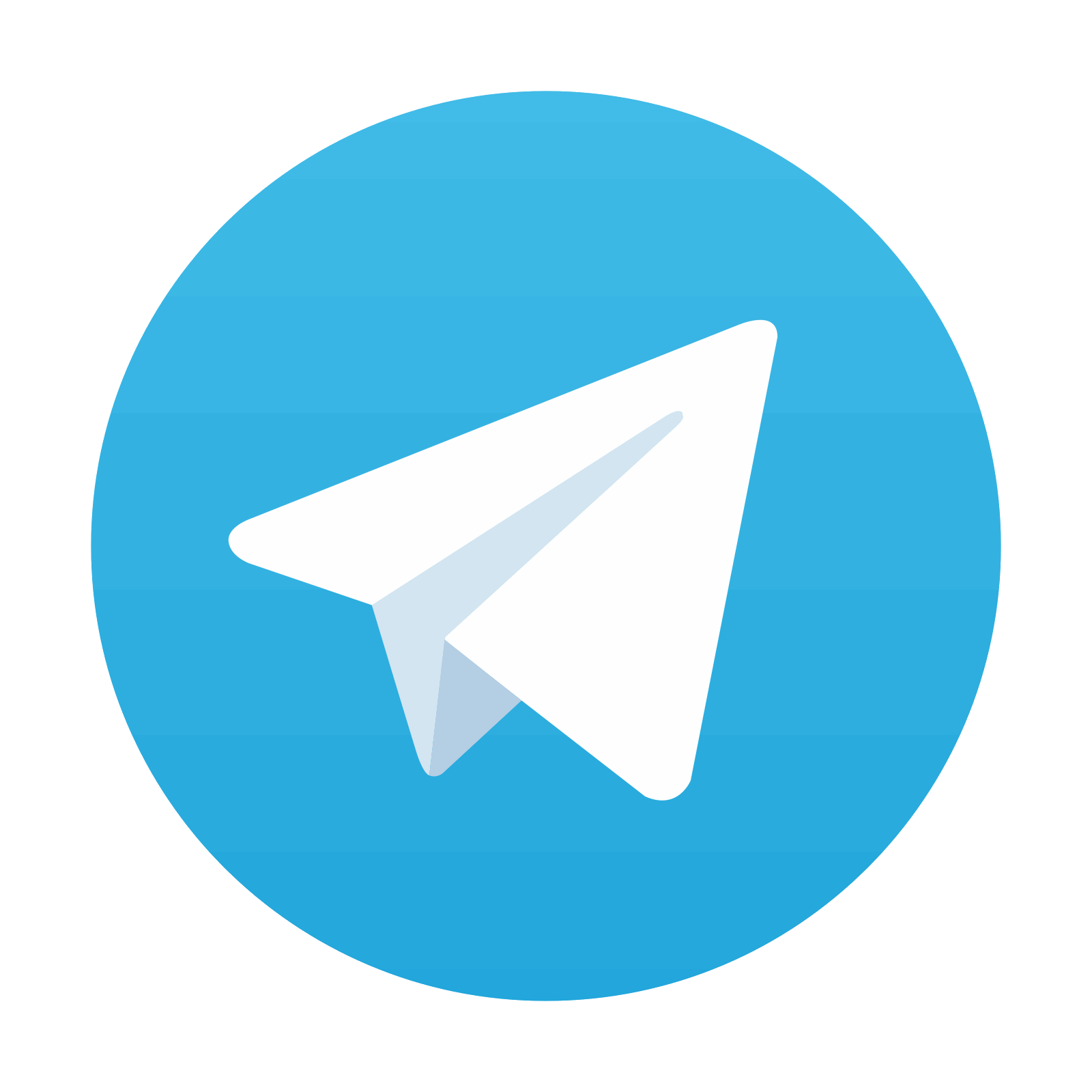
Stay updated, free articles. Join our Telegram channel

Full access? Get Clinical Tree
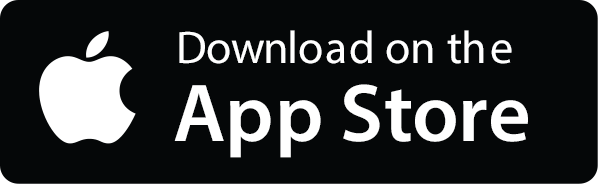
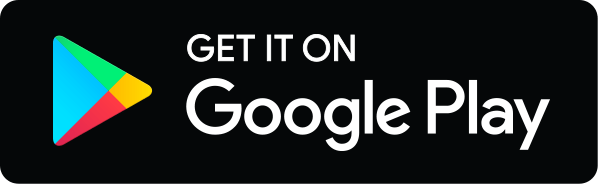