Fig. 6.1
(a) Left upper jugular lymph node metastasis (white arrow) from nasopharyngeal cancer was noted on CT before IMRT. (b) On the 25th day of IMRT (after delivery of 36 Gy in 18 fractions), the metastatic lymph node had shifted 1 cm medially because of body weight loss and tumor shrinkage
6.2 Concept of ART and Image-Guided RT
Tumors and normal tissues move with time, and this movement may be clinically significant from second to second, day to day, week to week, or longer, and the variation in their position can be considered to take place intra (within)- or inter (between)-treatment sessions. Each tumor site has its own movement characteristics. For example, thoracic tumors are predominantly affected by periodic respiratory motion, whereas head and neck tumors are affected more by gradual tumor shrinkage over time (Fig. 6.1). The position of prostate tumors can change from day to day owing to differences in bowel and bladder fillings, although additional momentary and irregular changes can also result from peristalsis. However, no tumor is immune from change in position, size, or movement, whether momentary or more gradual.
Substantial effort has been devoted in RT in reducing the effect of variations related to the treatment process, such as set-up error and geometric variations in volumes of targets and critical normal organs. To compensate for these variations (also called uncertainties), the use of predefined uniform margins around the CTV has been suggested. The term planning target volume (PTV) denotes a CTV that is volumetrically expanded to compensate for treatment set-up uncertainties [14]. Such expansions often present dosimetric trade-offs because they often overlap geographically with adjacent organs at risk. A favorable therapeutic ratio relies on accurate knowledge of set-up uncertainties, normal tissue dose tolerances, and delineation of treatment targets to safely minimize PTV expansions. However, radiation treatment planning with compensation strategies has not been customized to account for variations in individual patients over the course of treatment.
ART is an approach to correct for daily or weekly variations in tumor and normal tissue positioning or volume through online or offline modification of original RT plans [13, 15–17]. ART has two components: (1) means of detecting such changes and (2) means of intervention. Successful implementation of each of these components determines the overall success of the clinical application of ART. The first component of ART, detecting changes, is also known as image-guided RT (IGRT). Obtaining images from patients while they are in the treatment room can provide extensive datasets documenting movements and anatomic change of targets during and between RT sessions. The use of computed tomography (CT) in the treatment room can depict geometrically accurate soft tissue targets. In-room CT scanners, tomotherapy-based megavoltage CT, and gantry-mounted cone-beam CT are now all available to provide in-room three-dimensional (3D) imaging [16, 17]. Such imaging can reveal two types of variation: systematic, in which the mean of the observed positions is offset from the prescribed position, or random, which can be measured as daily changes from the mean. Systematic uncertainties are amenable to offline analyses of images acquired at treatment. However, random errors can be addressed only with daily online imaging and modification. Thus, IGRT can reduce set-up uncertainties and improve the management of organ motion, consequently allowing dose escalation, an improved therapeutic ratio, or both. Because the goal of IGRT is to correct for set-up errors and minimize the PTV margin, image guidance does not typically involve modification of the original treatment plan but rather may involve repositioning treatment fields (Fig. 6.2).
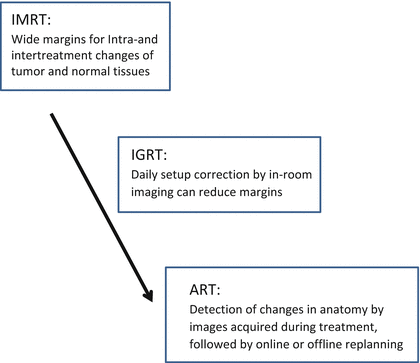
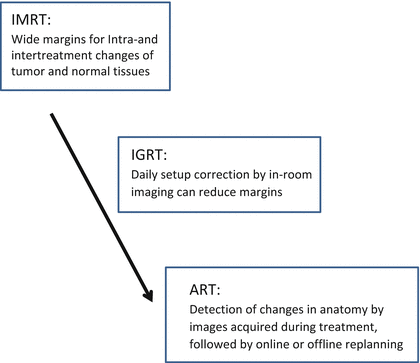
Fig. 6.2
The concept of ART in comparison with IMRT and IGRT
In contrast to IGRT, the intent of ART is to appropriately modify a radiation treatment plan to account for temporal changes in anatomy (Fig. 6.2). In theory, ART can occur on three different timescales: offline between fractions, online immediately before a fraction, and in real time during a treatment fraction [16, 17]. ART is closely linked to image-guidance processes because any volumetric images acquired for the IGRT procedure could also be used to monitor changes in anatomy and to design new plans. Ideally, in-room volumetric images could be sent to a treatment-planning system, where a new treatment plan based on current anatomy could be generated via automated deformable image registration software and sent back to the therapy machine for delivery. The adapted plan could be either deployed immediately (online correction) or used for future treatments (offline correction).
Manual segmentation of treatment-planning images requires too much time and effort on behalf of treating physicians and staff to be practical for the routine use of ART. Deformable image registration for atlas-based autosegmentation is an effective alternative for serial adaptive replanning [18–21]. If the contour exists in one of the reference CT images, then deformable transformation can be used to transform reference contours onto the newly acquired CT images with minimal manual input. This approach is well suited to ART, given that the original plan can serve as the reference for this process. Manual recontouring can take hours, which would not be practical for online ART and would strain resources for the application of offline ART as well.
6.3 Volume and Dosimetric Changes During IMRT
Several studies have demonstrated that anatomic changes take place during IMRT with concurrent chemotherapy for head and neck cancer [7, 9, 20, 22–24]. In one of these studies, Barker et al. [7] evaluated 14 patients with head and neck cancer treated by an integrated CT-linear accelerator system that allows CT imaging at daily RT sessions; these investigators noted rapid weight loss and anatomic change in both tumors and at-risk organs after 3–4 weeks of RT. Specifically, the gross tumor volume (GTV) decreased throughout therapy at a median rate of 0.2 cm3 per treatment day (range, 0.01–1.95 cm3/day), and the GTV decreased at a median rate of 1.8 % per treatment day. The absolute volume loss was larger for large tumors and nodes. By the final day of treatment, the median relative loss of the GTV was approximately 70 % (range, 10–92 %), and the center of the mass was displaced by a median 3.3 mm (range, 0–17.3 mm). The volume of the parotid glands also decreased over time; at the end of treatment, the median loss was 28 % (range, 5.9–53.6 %), and the median medial shift was 3.1 mm (range, −0.3 to 9.9 mm) [7].
Wu et al. [20] retrospectively analyzed 11 patients with locally advanced head and neck cancer who had had one IMRT planning scan and 6 weekly helical CT scans. Each patient had 1–6 repeated plans (“replans”) created based on the weekly helical CTs, and doses were accumulated on the planning CT. Although the cumulative doses to targets or the spinal cord, brainstem, and mandible were unchanged on the replans relative to the original plans, significant increases in parotid doses were observed without replanning. These investigators concluded that replanning would preserve sparing of only the parotid glands.
On the other hand, Hunter et al. [18] evaluated 18 patients with oropharyngeal cancer by daily cone-beam CT with clinical set-up alignment. In that study, differences between planned and delivered doses to the parotid glands were small relative to the standard deviations of the dose–salivary flow function, suggesting that ART is not likely to produce measurable improvements in salivary output in most cases.
Duma et al. [25] reported a dosimetric advantage of ART triggered exclusively by soft tissue changes detected on daily set-up helical megavoltage CT during helical tomotherapy for head and neck cancer. At those authors’ institution, ART is routinely used for patients with head and neck cancer when (1) inspection or palpation reveals a change in soft tissues or the mask was loose or (2) the IGRT CT scan shows a soft tissue change >5 mm. Of 94 patients with head and neck cancer treated with helical tomotherapy, replanning was done for 11 patients (12 %) for whom soft tissue changes were >5 mm as identified by IGRT CT (i.e., none had clinically evident changes); replanning was done mostly at the end of the third week of treatment. In these patients, shrinkage of the body diameter by 1 cm did not affect the coverage of the PTV but translated into a slightly higher dose to the PTV itself and a higher delivered dose to the normal tissues outside the PTV.
At our institution, we adopted a two-step method for IMRT for head and neck cancer and are exploring its use as ART [8, 9, 11]. We obtain two sets of treatment-planning CT scans, the first before IMRT (CT-1) and the second during the third or fourth week of IMRT (CT-2); the second set of plans is used for an IMRT boost dose after 46–50 Gy has been given and the patient fitted with a new thermoplastic mask. We recently analyzed geometric and dosimetric changes between the first and second set of scans in 20 consecutive patients treated with this two-step IMRT process [8]. Twenty consecutive patients with pharyngeal cancer (10 nasopharyngeal, 6 oropharyngeal, and 4 hypopharyngeal) were treated with a two-step IMRT method in which 46–50 Gy was given in 23–25 fractions to the whole neck, followed by a boost dose to the high-risk CTV for a total dose of 60–70 Gy in 30–35 fractions (median dose, 70 Gy); patients also received concurrent cisplatin-based chemotherapy (80 mg/m2 every 3 weeks). The spinal cord and parotid glands were recontoured without margins on both sets of CT scans, and the primary tumors (GTV-p) and the largest metastatic lymph node (GTV-n) were also recontoured without margins. On the CT-2 scans, the mean GTV-p volume had dropped to 37 % ± 24 % of the CT-1 plan (P = 0.002) and the mean GTV-n volume to 48 % ± 37 % of the CT-1 value (P = 0.081). The parotid gland volume also decreased to 82 % ± 12 % of the initial volume (P < 0.0001), and the lateral surface of the parotid glands on CT-2 had shifted medially by an average of 4.2 ± 2.9 mm (P < 0.0001) [8].
To analyze dosimetric changes, we created three sets of IMRT plans: Plan-1 was the actual initial IMRT plan (based on the pretreatment CT-1 scans), Plan-2 was the actual boost plan (based on the CT-2 scans), and Plan-3, which involved transferring the initial IMRT plan to the CT-2 scans and matching them for isocenter and bony alignment. Dose distribution plans were then recalculated to obtain dosimetric parameters of the recontoured target volumes and organs at risk, and variables were compared between Plan-3 and Plan-1 to evaluate the effects of anatomic changes on dosimetric outcomes. The replanning effects for dosimetric outcomes were compared for Plan-2 and Plan-3. Dose parameters were calculated for a total prescribed dose of 70 Gy for each plan. The mean doses to the parotid glands (D mean) were 25.4 Gy in Plan-1, 20.0 Gy in Plan-2, and 30.3 Gy in Plan-3 [8]; these differences were significant for Plan-1 vs. Plan-3 (5.0 ± 5.1 Gy, or 120 %; P < 0.0001) and for Plan-2 vs. Plan-3 (10.3 ± 3.6 Gy, or 66 %; P < 0.0001). The corresponding doses to 2 % of the spinal cord (D2) were 37.2 Gy in Plan-1, 36.7 Gy in Plan-2, and 39.1 Gy in Plan-3 (significant difference for Plan-3 vs. Plan-1 [1.9 ± 2.0 Gy or 105 %; P = 0.0003] and marginally significant difference for Plan-2 vs. Plan-3 [2.4 ± 5.2 Gy or 94 %; P = 0.0507]). These findings, plus those from another group showing the value of adaptive replanning for significant changes in the maximum dose to the spinal cord and dose to 50 % of the parotid glands (D50) [22], lead us to conclude that our two-step IMRT method can effectively prevent increases in high-dose exposure of the spinal cord and parotid glands.
Figure 6.3 illustrates two sets of plans, the Plan-1 (lower left) based on the CT-1 scan (upper left) and the Plan-3 (lower right) based on the CT-2 scans (upper right), for a patient with nasopharyngeal cancer. Shrinkage of the neck diameter is evident on CT-2, and the left upper jugular lymph nodes (GTV-n; white arrow) have apparently regressed as well. On Plan-3 (lower right), the parotid glands had shifted so that, in the absence of replanning, they were now included in the 70 % dose region (blue color). Comparing Plan-1 with Plan-3 also indicated a slight (but significant) increase in D mean to the GTV-p (0.6 ± 0.7 Gy, P = 0.0007) and D98 to the GTV-p (0.8 ± 0.6 Gy, P < 0.0001) but no changes in the D mean and D98 of the GTV-n. These findings lead us to conclude that planned doses to GTV-p and GTV-n could be delivered by using the initial IMRT plan, without ART.
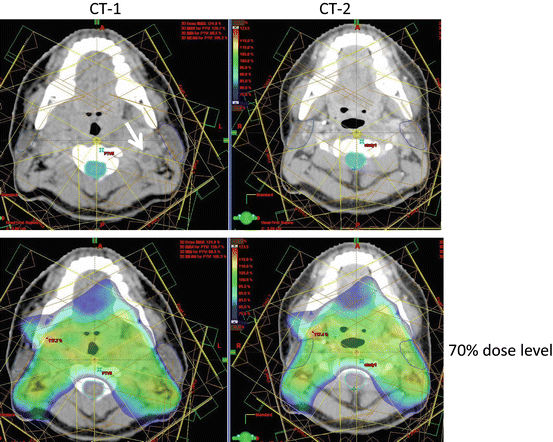
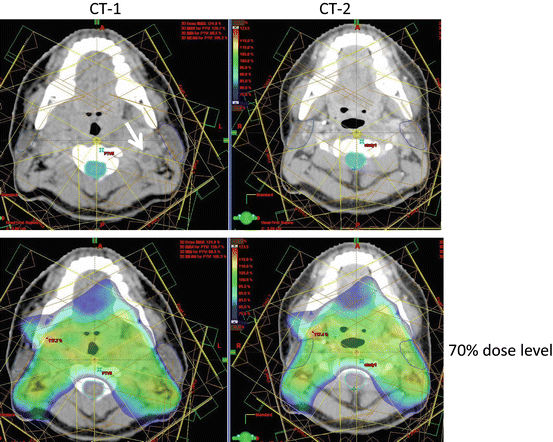
Fig. 6.3
Top row, treatment-planning CT scan obtained before IMRT (CT-1, upper left) and a second CT scan (CT-2, upper right) obtained after 38 Gy in 19 fractions for a patient with nasopharyngeal cancer. Shrinkage of the neck diameter is evident on CT-2, and the left upper jugular lymph nodes (GTV-n; white arrow) have apparently regressed as well. Bottom row, dose distributions on the initial IMRT plan on CT-1 (Plan-1, lower left) and the same plan transferred onto CT-2 (Plan-3, lower right). Most of the bilateral parotid glands would have been included in the 70 % dose level (blue color) in Plan-3
6.4 Clinical Studies of ART
The dosimetric advantage of ART in reducing the dose to various organs at risk such as the parotid glands and spinal cord is apparent. The substantial investments in equipment, staffing, and staff time required for widespread implementation of ART require demonstration that these dosimetric advantages will translate into clinical benefit. However, to date only a few studies have prospectively evaluated the clinical benefit of ART for patients with head and neck cancer.
In one such study, Schwartz et al. [19] described the use of ART for patients with advanced head and neck cancer in which a daily CT-guided setup and deformable image registration were used. Of the 22 patients enrolled, all required at least one round of replanning because of changes in CTV and normal tissues, and eight patients (36 %) required a second replanning. The median trigger point for the first adaptive plan was the 16th treatment fraction. For the eight patients with two replans, the median trigger points for the first replan were the 11th fraction and the 22nd fraction for the second replan. The elapsed interval from triggering in-room CT imaging to subsequent delivery of the prompted ART plan was 1.7 working days (median, 2 days; range, 1–4 days). At a median follow-up time of 31 months, disease control rates at 2 years were 100 % local and 95 % regional. Parotid dose sparing was improved with a single ART replanning by a mean of 0.6 Gy for the contralateral parotid and 1.3 Gy for the ipsilateral parotid relative to standard IGRT; for the eight patients with two replans, these values were 0.8 Gy and 4.1 Gy. Chronic toxicity in this study was considered encouraging, and the locoregional control rate was considered excellent.
Berwouts et al. [26] reported a phase I clinical trial of three-phase ART for head and neck cancer. Ten patients were enrolled, and scans were obtained before treatment, after 8 fractions, after 18 fractions, and at the end of treatment. All patients completed treatment without interruptions or severe acute toxicities. The extent of GTV reduction at the end of treatment was 72 %. At a median follow-up time of 13 months, 9 of 10 patients had no evidence of disease. The authors concluded that their three-phase ART process, in which currently available tools were used, was feasible.
In another clinical study, Zhao et al. [27] retrospectively evaluated the role of replanning in IMRT for nasopharyngeal cancer. Of 175 patients with nasopharyngeal cancer who had been treated with IMRT, 158 showed obvious anatomic changes including tumor shrinkage, nodal shrinkage, or weight loss before 20 fractions had been delivered; 33 of those patients had repeat CT scanning and replanning during the course of treatment, and their outcomes were compared with those of 66 control patients who had not had replanning who were matched in terms of clinical disease stage and type of anatomic changes. IMRT replanning improved the 3-year local progression-free survival rate for patients with T3–T4 tumors and also reduced late effects for patients with large lymph nodes (N2, N3). These investigators concluded that ART is recommended for patients with advanced (T3, T4 or N2, N3) nasopharyngeal cancer. Indeed, because patients with locally advanced nasopharyngeal cancer often present with large lymph nodes in the neck and because both primary tumors and neck lymph nodes regress rapidly with RT, ART is especially desirable for such disease. Lee et al. [5] described a phase I/II study of a two-step, two-plan method involving the use of IMRT with a simultaneous integrated boost (SIB) technique for 20 patients with nasopharyngeal cancer. The results of this study suggested that tumor regression of early-stage pharyngeal tumors may be sufficiently small as to render ART unnecessary; however, even patients with early-stage tumors showed notable changes in body weight and contour when the RT was given with chemotherapy. Therefore, we consider ART to be indicated for head and neck cancer that is to be treated by concurrent chemoradiation.
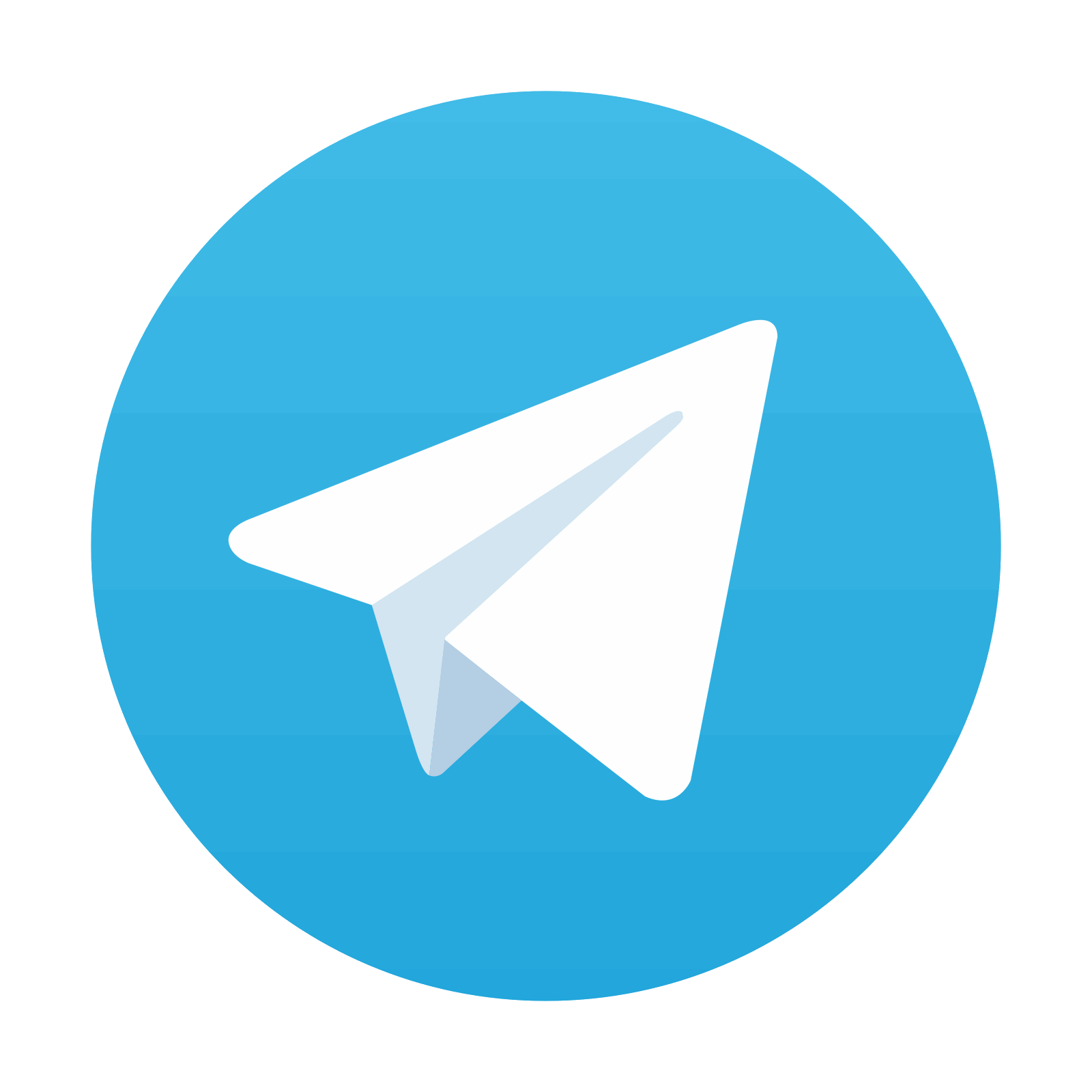
Stay updated, free articles. Join our Telegram channel

Full access? Get Clinical Tree
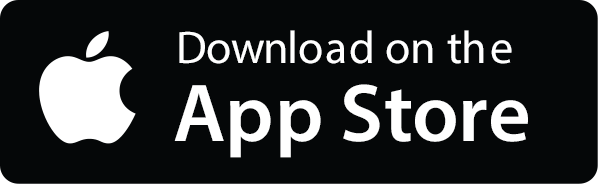
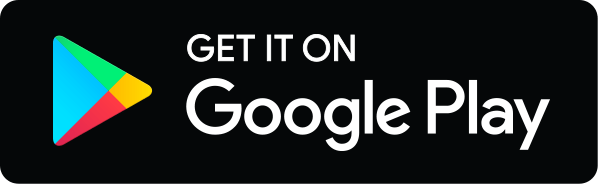