Fig. 1
The core SMRT/NCoR complex is recruited to chromatin through the ligand-binding domains of unliganded-nuclear receptors to repress transcription. The SMRT/NCoR complex transiently assembles with chromatin modifying enzymes and other factors to form a large protein complex that regulates gene expression
SMRT and NCoR are large proteins (~ 2500 residues) that interact with many nuclear receptors, other transcription factors, histone deacetylases and other scaffold proteins. The amino-terminus of SMRT (residues 168–725) is the most structured region of the protein; is highly conserved between SMRT and NCoR (68 % identity) and forms the core of the repression complex. In contrast, the carboxy-terminal region of the protein (c. 1700 residues) contains almost no predicted secondary structure and in large part is predicted to be intrinsically disordered (i.e. lacks an intrinsically fixed structure) (Fig. 2).
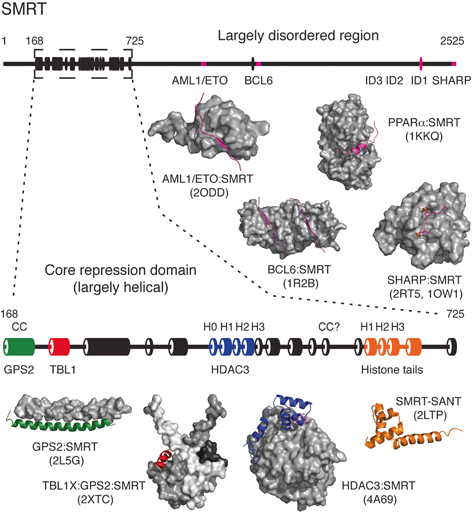
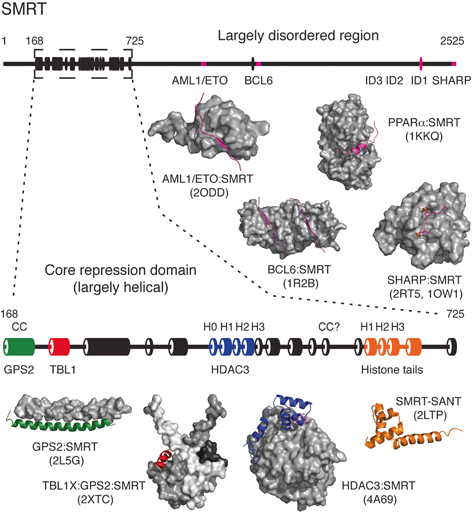
Fig. 2
The nuclear receptor corepressor SMRT is largely intrinsically disordered except for the core repression domain found towards the amino-terminus of the protein (residues 168–725). The secondary structure prediction of SMRT is shown with α-helices depicted as cylinders and with the core repression domain enlarged for clarity (prediction made using http://bioinf.cs.ucl.ac.uk/psipred). Structural characterisation of SMRT has been most successful through the study of SMRT fragments in complex with other proteins from the SMRT corepressor complex. Structures are illustrated with SMRT coloured to match the secondary structure prediction and shown as cartoon
Within the amino-terminal region, there are two structured SANT domains. The amino-terminal domain has been shown to be essential for recruitment of histone deacetylase 3 (Wen et al. 2000; Guenther et al. 2001). In contrast the second SANT domain has been reported to mediate interactions with histones (Yu et al. 2003; Hartman et al. 2005). Given that the two SANT domains are 36 % identical and 69 % similar, it seems likely that they arose by domain duplication followed by functional divergence (Boyer et al. 2004). Interestingly, both domains have a basic charged surface suggesting that if the second SANT domain is mediating interactions with histones, it may also be interacting with negatively charged DNA wrapped around the histone octamer.
The region of SMRT and NCoR amino-terminal to the first SANT domain contains the region that has been shown to be responsible for the recruitment of the proteins GPS2 and TBL1X which form the core scaffold of the repression complex (Guenther et al. 2000; Zhang et al. 2002).
Throughout the apparently unstructured carboxy-terminal region of the corepressors there are short stretches of residues that are conserved between SMRT and NCoR. Several of these have been shown to act as interaction motifs for transcription factors and other proteins including unliganded or antagonist-bound nuclear receptors. These sequence motifs seem to become structured upon forming a complex with their respective partner proteins (see below).
Although SMRT and NCoR are the best characterised of the nuclear receptor corepressors, a great many other proteins have been implicated in mediating transcriptional repression by nuclear receptors. Some of these, such as RIP140, Hairless and LCoR, act as repressors of agonist bound nuclear receptors and probably serve to attenuate activation (Cavaillès et al. 1995; Potter et al. 2001; Fernandes et al. 2003). Like SMRT and NCoR, these proteins are in large part intrinsically disordered suggesting that this is a functionally important characteristic of this family of proteins.
3 The Core SMRT/NCoR Repression Complex
When SMRT or NCoR are purified from cells, three proteins (GPS2, TBL1X and HDAC3) invariably co-purify as a complex with the corepressor (Guenther et al. 2000; Li et al. 2000). This complex shows resistance to dissociation and is stable in the presence of high salt, moderate sodium dodecyl sulfate and non-ionic or ionic detergents suggesting that it acts as a stable core to the repression complex (Zhang et al. 2002). HDAC3 is a histone deacetylase with a well-established role in nuclear receptor mediated transcriptional repression (Yang et al. 1997; Dangond et al. 1998). GPS2 is a G-protein signalling regulator, initially discovered in a yeast pheromone response pathway, and shown to be involved in MAP kinase cascades (Spain et al. 1996). GPS2 has been shown to have an important role in hepatic bile acid synthesis and promotes adipose tissue inflammation in obese subjects (Sanyal et al. 2007; Toubal et al. 2013). Mutations in GPS2 have also been linked to medulloblastoma (Pugh et al. 2012).
TBL1X (and its closely related homologues TBL1Y and TBL1XR1) are WD40 repeat-containing proteins that have been shown to be involved in human hearing; loss-of-function mutations in TBL1X have been linked to deafness (Bassi et al. 1999). TBL1X has been shown be important for maintaining a healthy liver fat content through interaction with PPARα; TBL1X deficiency results in fatty liver development and further metabolic syndromes such as steatosis and hypertriglyceridemia (Kulozik et al. 2011).
HDAC3, GPS2 and TBL1X interact with the highly conserved core-region of SMRT (168–725). Residues 168–297 are sufficient to bind to both GPS2 and TBL1X, whereas residues 409–475 are required for the recruitment of HDAC3 (Oberoi et al. 2011). In between these two interaction domains there are three predicted α-helices that may also contribute to the interaction with the TBL1X and/or HDAC3. Importantly it has been shown that there is a three-way complex between SMRT, GPS2 and TBL1X, such that SMRT and GPS2 interact directly with each other as well as both interacting with TBL1X, thus forming a tight three-way complex (Oberoi et al. 2011).
Structural studies have demonstrated that residues 167–207 of SMRT form an anti-parallel coiled-coil with residues 53–90 of GPS2 (Fig. 3) (Oberoi et al. 2011). The anti-parallel orientation positions the two regions that bind to TBL1X at one end of the coiled coil. Residues 227–297 of SMRT and 1–52 of GPS2 interact with the amino-terminal domain of TBL1X . Modelling, together with interaction mapping studies, has shown that the interaction regions in SMRT and GPS2 form short helical structures that bind in grooves on either side of a TBL1X dimer.
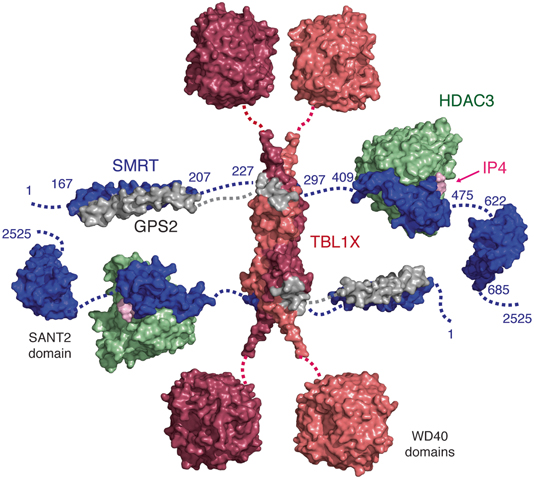
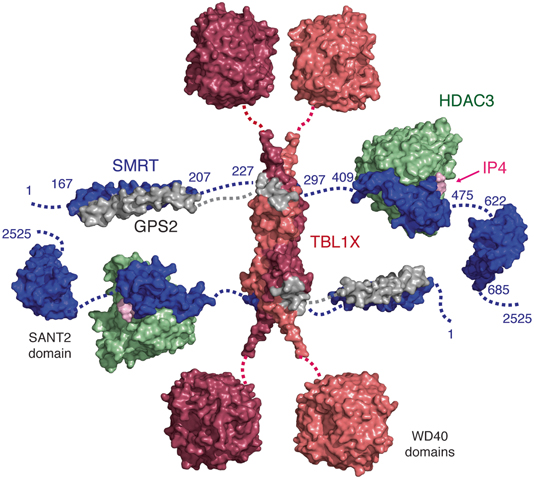
Fig. 3
A schematic model of the core repression domain of SMRT (blue) showing the characterised interactions with HDAC3 (green), IP4 (pink), GPS2 (grey) and TBL1X (salmon and red). SMRT is arranged in a linear format for simplicity but may fold to a more compact arrangement in solution. SMRT:GPS2 (pdbcode 2LG5), TBL1X tetramer (pdbcode 2XTC), WD40 domains of TBLXR1(pdbcode 4LG9), HDAC3:SMRT-SANT1 (pdbcode 4A69, 1XC5), SMRT-SANT2 (pdbcode 2LTP). Dotted lines indicate regions of SMRT that have not been structurally characterised
TBL1X consists of a LisH domain and a WD40 domain. The LisH domain of TBL1X forms a homodimer with an antiparallel four-helix bundle stabilised by polar and non-polar contacts. Two additional helices cross over to form an X-shaped structure that rests on the four-helix bundle. Two TBL1X dimers interact through one surface of the four-helix bundle so as to form a tetramer (Oberoi et al. 2011). The similarity between TBL1X, TBL1Y and TBL1XR1 is such that it would be expected that a TBL1 tetramer could be formed from any combination of these closely related proteins.
An eight-bladed WD40 domain is located carboxy-terminal to the TBL1X tetramerisation domain. Thus the tetramerisation of TBL1X amino-terminal domain draws together four WD40 domains into close proximity (which may have implications for chromatin targeting). The WD40 domain structure of TBLR1X has been solved (PDBcode 4LG9) and resembles the WD40 domain from WDR5 that is known to mediate interactions with chromatin (Wysocka et al. 2005; Couture et al. 2006; Ruthenburg et al. 2006) . It is possible that the WD40 domains in TBL1X will serve a similar role.
The tetramerisation of TBL1X also implies that the whole complex will contain 2 copies of SMRT or NCoR, 2 copies of HDAC3 and 2 of GPS2 along with the TBL1X tetramer. This would equate to a total molecular weight of approximate 1 MDa that fits well with the reported size of the complex when purified from nuclear extracts (Guenther et al. 2000; Li et al. 2000; Varlakhanova et al. 2011).
4 Assembly of HDAC3 in the SMRT/NCoR Complex
As mentioned earlier, the first of the two SANT domains in SMRT and NCoR has been shown to be responsible for the recruitment of HDAC3. Surprisingly, it was also found that enzymatic activity of HDAC3 was very significantly enhanced through interaction with this domain which was named accordingly: “the deacetylase activation domain” (DAD) (Guenther et al. 2001). The SMRT-DAD is in fact an extended SANT domain which was shown by NMR to fold into a compact four-helical structure composed of a canonical three-helix bundle SANT domain, and amino-terminal helix termed H0 (Codina et al. 2005). Structure-guided mutagenesis was used to determine the residues that were required for binding and those that were essential for activating HDAC3. The molecular detail of this interaction was clarified through the crystal structure of the SMRT-DAD bound to HDAC3 (Watson et al. 2012). The structure shows that the isolated SMRT-DAD must undergo a major structural rearrangement on binding. Helix H0 unfolds to expose an HDAC3 binding surface, and both helix H0 and the SANT domain make extensive intermolecular interactions with the surface of HDAC3. Whether this unfolding transition occurs on binding HDAC3 or whether the complex is assembled directly after synthesis is uncertain.
5 HDAC Activity Is Regulated by Inositol Phosphates
The structure of the HDAC3:SMRT complex led to the surprising finding that there was an inositol tetrakisphosphate molecule (Ins(1,4,5,6)P4) located at the interface of HDAC and the SANT domain of the corepressor (Watson et al. 2012) . The inositol phosphate co-purified with the complex that had been expressed in HEK293 cells. Subsequent deacetylase assays revealed that the IP4 can be washed out of the HDAC3:SMRT complex using high salt resulting in an enzymatically inert complex (Millard et al. 2013). Addition of inositol phosphates leads to the restoration of full deacetylase activity suggesting that inositol phosphates might be bona fide regulators of HDAC3 activity. The concentrations of these small signalling molecules in the cell has been shown to be sufficiently high to make them physiologically relevant regulators of HDAC activity (Barker et al. 2004). Whilst the exact mechanism of activation by inositol phosphate binding has yet to be fully determined there is some evidence that this involves the stabilisation of the active site channel (Watson et al. 2012; Arrar et al. 2013) . The biological rationale for regulation of HDAC3 by inositol phosphates remains to be established.
6 The SMRT/NCoR Complex Is A Paradigm for Other Class I HDAC Complexes
Intriguingly, several other corepressor proteins that recruit HDACs 1&2 contain very similar SANT domains to that of the SMRT-DAD. These include the MTA proteins from the NuRD complex (nucleosome remodelling and deacetylase complex) and RCOR proteins from the CoREST complex (cofactor of REST). In both these corepressors, the SANT domain is preceded by an ELM2 domain that has been shown to be important for HDAC recruitment (Toh et al. 2000; Lee et al. 2006). Although, not as firmly established as for the SMRT/NCoR complex, there is evidence that both the NuRD and CoREST complexes associate with nuclear receptors. MTA1 directly binds estrogen receptor-α via an nuclear receptor binding motif found in a naturally occurring short form of the corepressor protein (Kumar et al. 2002). LSD1 (part of the CoREST complex) associates with the androgen receptor (Metzger et al. 2005).
The structure of MTA1 bound to HDAC1 shows that the MTA1-SANT domain binds to the HDAC1 in a very similar fashion to the SMRT-DAD domain binding to HDAC3 (Millard et al. 2013) . As was observed for the HDAC3:SMRT complex, there is a basic inositol binding pocket formed at the interface between HDAC1 and the MTA1-SANT domain. Biochemical assays confirm that inositol phosphates also regulate the HDAC1 activity in this complex.
The ELM2 domain of MTA1 is also present in the HDAC1:MTA1 structure and is shown to wrap completely around the catalytic domain of HDAC1 in an extended groove. This positions the amino-terminus of the ELM2 domain and carboxy-terminus of the SANT domain on either side of the active site. Interaction studies with HDAC3 and SMRT show that, although there is only limited sequence conservation, a region amino-terminal to the SANT domain in SMRT also contributes to interaction with HDAC3 (Millard et al. 2013). This is likely to mimic the ELM2 domain, and so wrap around HDAC3, drawing TBL1 and GPS2 closer to the histone deacetylase. This extensive interface would correlate well with the observed stability of the HDAC3:SMRT complex.
HDACs 1&2 are highly similar (83 % identical) and are recruited interchangeably to the same repression complexes including the NuRD, CoREST and Sin3A complexes (Laherty et al. 1997; Xue et al. 1998; Lee et al. 2005). In contrast, whilst HDAC3 is also similar to HDACs 1&2 (53 % identical), it is recruited uniquely to the SMRT/NCoR complex. Careful comparison of the interactions of MTA1 and SMRT with HDACs 1&3, respectively, reveal a series of subtle but sufficient differences to result in the HDACs being recruited their cognate partners. In particular, there are two distinct regions in that contribute primarily to the specificity of assembling these highly related HDAC:corepressor complexes (Fig. 4) (Millard et al. 2013).
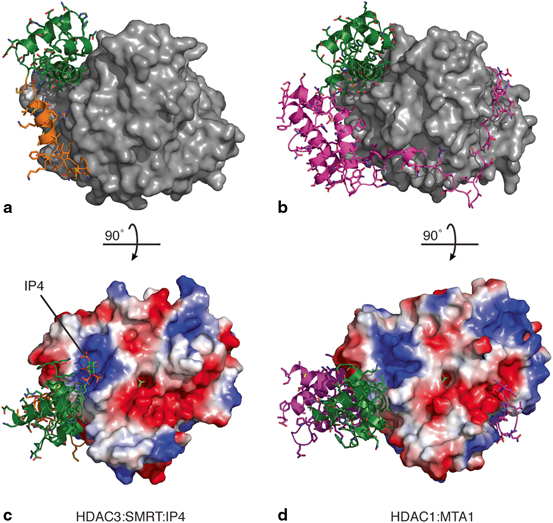
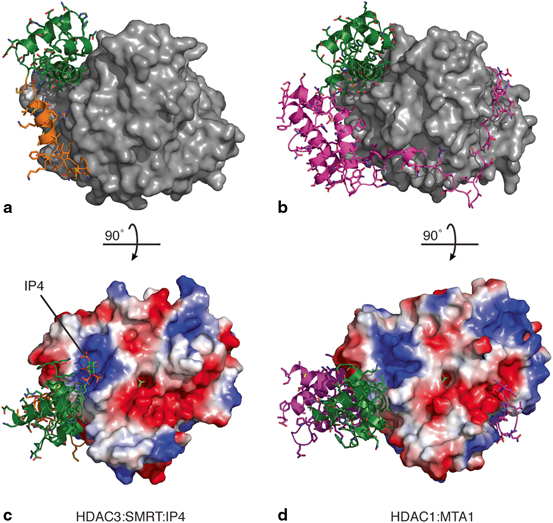
Fig. 4
Structures of a HDAC3:SMRT and b HDAC1:MTA1 corepressor complexes. The HDACs are illustrated as surfaces (grey) with the bound corepressors are shown as cartoons. These are coloured to highlight the SANT domains (green), helix H0 of SMRT (orange) and the ELM2 domain of MTA1 (magenta). The HDAC active sites are located at the top of each panel. Electrostatic surface profiles of c HDAC3 and d HDAC1 with their cognate corepressors following a 90° rotation. An acetate molecule can be seen in the HDAC active sites (green). Inositol phosphate (green and orange) is bound to the HDAC3:SMRT in a basic binding pocket at the interface between the molecules. A similar basic pocket is formed at the interface between HDAC1 and MTA1 and could accommodate an inositol phosphate molecule . HDAC3:SMRT (pdbcode 4A69) and HDAC1:MTA1 (pdbcode 4BKX)
7 Recruitment of Repression Complexes to Nuclear Receptors
Corepressor proteins are recruited to specific genomic loci through interactions with the ligand-binding domains (LBDs) of unliganded nuclear receptors. There are 48 unique receptors and many of their LBDs have been structurally characterised by crystallography (reviewed in (Rastinejad et al. 2013)). The LBD has a three-layered α-helical sandwich fold and the ligand-binding pocket is found within the middle layer (Bourguet et al. 1995; Renaud et al. 1995; Wagner et al. 1995). The ligand is shielded from the external environment when bound within this hydrophobic pocket. A carboxy-terminal helix (known as helix 12 or AF2 helix) lies across this pocket and can make direct contact with the ligand.
Before exploring how repression complexes are recruited to nuclear receptors , it is useful to consider how coactivators are recruited to ligand-bound nuclear receptors. Sequence alignment of short activating fragments from RIP140, SRC1 and CBP identified a highly conserved consensus motif LxxLL (NR box) that was sufficient for binding (Heery et al. 1997; Darimont et al. 1998). The molecular detail of NR box recruitment has been revealed through structural studies of isolated LBDs bound to short coactivator peptides (Nolte et al. 1998; Watkins et al. 2003). The LxxLL motif adopts a helical structure on binding to the surface of the LBD and makes contact along a hydrophobic binding groove formed by helices 3, 4, 5 and 12. Helix 12 is promoted to the “active” conformation on ligand binding and is essential to support coactivator binding (reviewed in (Nagy and Schwabe 2004)).
More recently, full-length nuclear receptors have been characterised bound to coactivator peptides (Chandra et al. 2008; Chandra et al. 2013; Lou et al. 2014). The structures of full-length heterodimer PPARγ-RXRα, HNF4α homodimer, and RXRα-LXRβbound to DNA provide insight into the relative positioning of the DNA-binding domain with respect to the LBD. Peptide binding to the full-length receptor is largely similar to that seen in the isolated LBDs, and since binding is some distance from the DNA-binding domain, it is suggested that the other domains do not directly modulate coactivator binding.
In contrast to coactivator binding, repression complex recruitment to nuclear receptors is more favourable in the absence of ligand. Mapping studies and sequence alignment revealed that recruitment occurs through the consensus motif LxxH/IIxxxI/L (CoRNR box) (Hu and Lazar 1999; Nagy et al. 1999; Perissi et al. 1999). There are three CoRNR box motifs or interaction domains (ID1, ID2 and ID3) that occur in both SMRT and NCoR (Webb et al. 2000) . The first structure of an LBD (PPARa bound to antagonist GW6471) with bound corepressor peptide ID1 (SMRT) showed that the CoRNR box assumes a helical fold and its binding prevents helix 12 from assuming an active conformation (Xu et al. 2002). This crystal structure showed that coactivator and corepressor binding is mutually exclusive as both bind to the same surface of the LBD. The SMRT corepressor peptide has a larger interaction interface with the LBD than that of coactivator motifs and is not dependant on helix 12. Further LBD crystal structures with bound corepressor peptides have been solved, and of note, are the two ligand-free corepressor-bound structures that are now available (structures and relevant references are detailed in Table 1). The first of these structures, Rev-erbα bound to ID2 (NCoR), showed that NCoR forms both the expected α-helix but also an unanticipated antiparallel β-sheet with helix 11 of the LBD (Phelan et al. 2010). This β-structure may be a feature that is specifically tailored for recruitment of Rev-erbα by ID2 (NCoR) but would not occur with ID1 (NCoR) due to sequence differences.
Table 1
A wide range of nuclear receptors have been structurally characterised with bound CoRNR box motifs (LxxH/IIxxxI/L) from NCoR and SMRT, and an NR box motif (LxxLL) from RIP140. Where indicated, the nuclear receptors have been crystallised in the presence of ligand. Numbering of CoRNR box motifs has been inconsistent but here the sites are numbered from the carboxy-terminus
Corepressor | Position | Residues | PDBcode | NR | Ligand | Reference |
---|---|---|---|---|---|---|
NCoR ID3 | 1933–1937 | NFIDVIITRQIAS | – | – | – | |
NCoR ID2 | 2055–2059 | DHICQIITQDFAR | 3N00 | Rev-erbα (NR1D1) | None | (Phelan et al. 2010) |
3KMZ | RARα (NR1B1) | Inverse agonist (BMS493) | (le Maire et al. 2010) | |||
NCoR ID1 | 2263–2267 | LGLEDIIRKALMG | 2OVM | PR (NR3C3) | Antagonist (Asoprisnil) | (Madauss et al. 2007) |
3 H52 | GR (NR3C1) | Antagonist (Mifepristone) | (Schoch et al. 2010) | |||
4II6 | FXR (NR1H4) | Partial agonist (Ivermectin) | (Jin et al. 2013) | |||
SMRT ID3a | Insert at 2049 | TFIDAIIMRQIAH | – | – | – | |
SMRT ID2 | 2147–2151 | QHISEVITQDYTR | – | – | – | |
SMRT ID1 | 2350–2354 | MGLEAIIRKALMG | 1KKQ | PPARα (NR1C1) | Antagonist (GW6471) | (Xu et al. 2002) |
2GPV | ERRγ (NR3B3) | Inverse agonist (4-OHT) | (Wang et al. 2006) | |||
2OVH | PR | Antagonist (Asoprisnil) | (Madauss et al. 2007) | |||
3R29 | RXRα (NR2B1) | None | (Zhang et al. 2011) | |||
3R2A | RXRα | Antagonist (Rhein) | (Zhang et al. 2011) | |||
RIP140 ID5 | 366–390 | NSLLLHLLKS | 2GPP | ERRγ | Agonist (GSK4716) | (Wang et al. 2006) |
2GPO | ERRγ | None | (Wang et al. 2006) |
The second of these structures, RXRα bound to ID1 (SMRT), was solved with the nuclear receptor in both the unliganded and antagonist bound state. The unliganded receptor was shown to be a tetramer in the crystal structure with each monomer binding to one SMRT peptide (Zhang et al. 2011). Interestingly the antagonist displaced the SMRT peptide allowing helix 12 from neighbouring molecules to bind to the vacated corepressor binding site.
Much work has focused on understanding how the LBD can switch binding preference from coactivator to corepressor. Several lines of evidence suggest that ligand binding promotes the stabilisation of the LBD, and this stabilisation drives coactivator binding, rather than the absolute position of helix 12 relative to the rest of the domain. Examination of the crystallography temperature factors of apo-LBDs, and further NMR mobility studies, suggest that the lower ligand-binding section of the domain is more mobile that the upper portion of the LBD (Nolte et al. 1998; Cronet et al. 2001; Watkins et al. 2003) . Further biochemical studies suggest that ligand binding stabilises the receptor, and this causes the LBD to become more compact and rigid (Keidel et al. 1994; Pissios et al. 2000). In the absence of ligand, the longer CoRNR box motif stabilises the ligand-free LBD. The mobility of helix 12 itself has been studied by fluorescence anisotropy and the helix shows much slower dynamics on ligand binding, suggesting that it associates with the surface of the LBD, presumably in the active position (Kallenberger et al. 2003). Therefore, helix 12 acts as a readout of ligand state, and plays a key role in selectively recruiting either coactivator or corepressor.
In contrast to SMRT and NCoR, RIP140 and LCoR are corepressor proteins that associate with ligand-bound nuclear receptors by means of an LxxLL motif. This motif occurs nine times in RIP140 and a single motif has been identified in LCoR (Heery et al. 1997; Fernandes et al. 2003). The recruitment of corepressors through the LxxLL motif suggests a very different biological rationale for repression since this sequence is most commonly found in coactivator proteins and mediates their recruitment to nuclear receptors (Cavaillès et al. 1995). However, like SMRT and NCoR, RIP140 and LCoR act as corepressors through the recruitment of HDACs complexes (Wei et al. 2000; Fernandes et al. 2003).
The stoichiometry of corepressor binding to dimeric, DNA bound nuclear receptors remains to be fully established. SMRT and other corepressors contain more than one NR or CoRNR box motif and therefore a single corepressor could in principle interact with both nuclear receptors in a homo- or hetero-dimer. Interestingly, several coregulator complexes are dimeric and contain two corepressor proteins that could both make interactions with nuclear receptor ligand binding domains. Although we can only speculate about the assembly of corepressor complexes, recent work has shown that the coactivator PGC-1α is recruited to ERRα and ERRγ asymmetrically such that a single NR box motif efficiently interacts with just one subunit of the homodimeric receptor (Takacs et al. 2013).
8 Recruitment of Other Transcriptional Regulators
SMRT and NCoR have been shown to associate with numerous other proteins beside those already described. In general, these further interactions tend to be relatively transient in comparison, but are nevertheless specific. Some of these interactions have only been loosely mapped to extended regions within SMRT , but others have been mapped in detail, and some of these interaction sites have been characterised through structural studies.
A small peptide from SMRT has been crystallised with the repressive transcription factor BCL6 and is shown to adopt a beta-strand on binding (Ahmad et al. 2003). BCL6 is required for normal B cell maturation, and deregulated expression leads to B-cell non-Hodgkin lymphomas. Residues 1414–1430 of SMRT bind to the homodimeric BTB domain of BCL6, with the amino-terminus of the SMRT peptide contributing a β-strand along an existing β-sheet at the bottom of the dimer, while the rest of the peptide binds in an extended conformation on the surface of the domain. BCL6 has also been crystallised in complex with a 17 residue peptide from BCoR (a non-homologous corepressor), and although it does not show any significant sequence similarity with the SMRT peptide , both peptides bind along the same lateral groove in BCL6 (Ghetu et al. 2008).
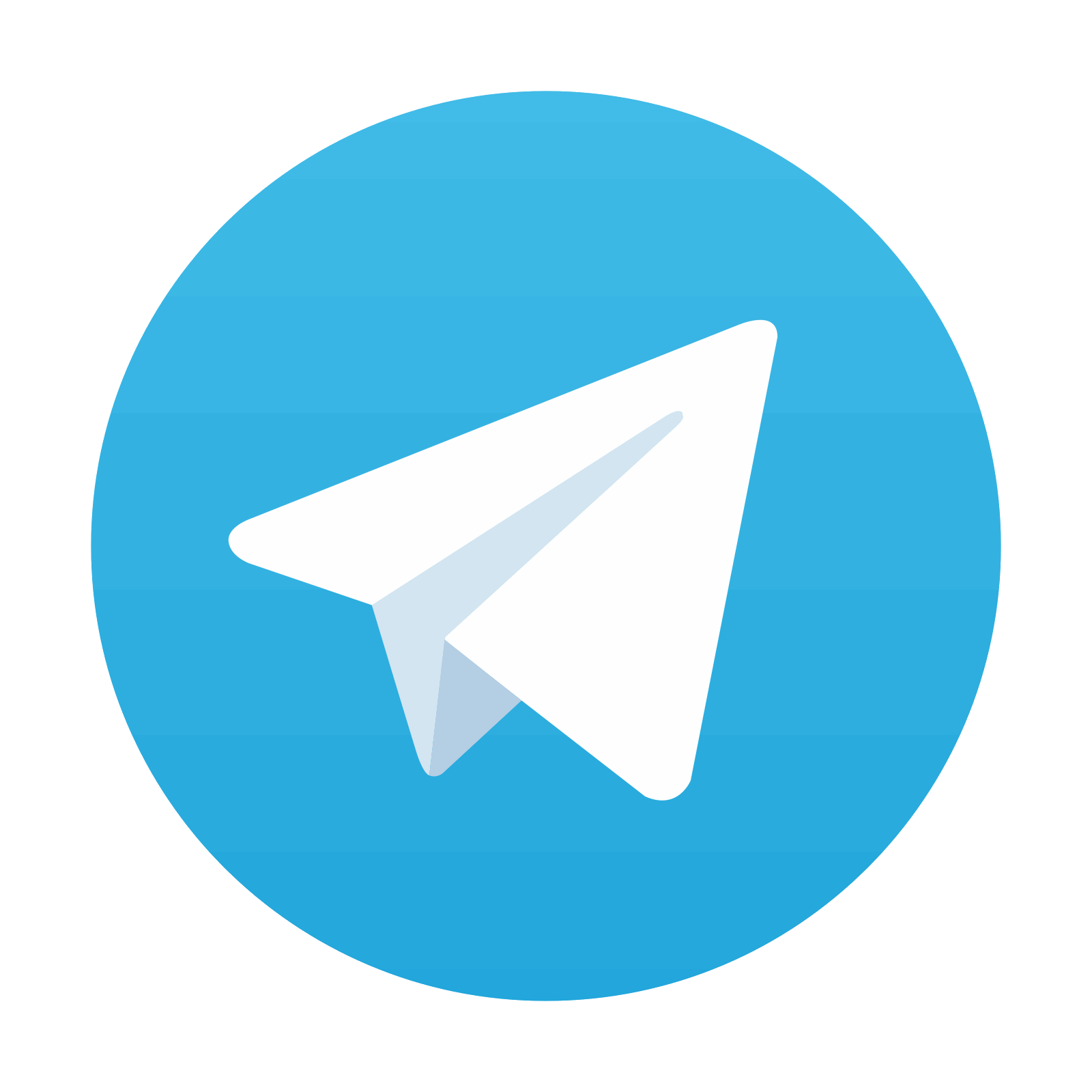
Stay updated, free articles. Join our Telegram channel

Full access? Get Clinical Tree
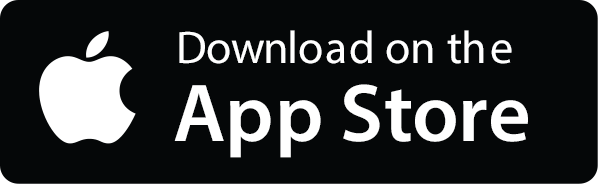
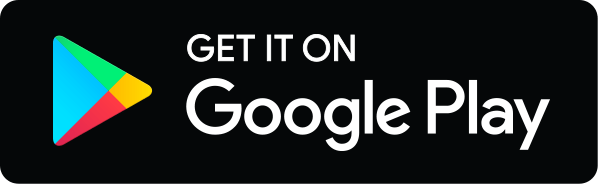