Fig. 1
Osteoporotic thoracic spine fractures in an 86-year-old female. Unenhanced CT in (a) axial and (b) sagittal plane
In a study including 155,031 women in the United States, aged between 50 and 99 years, a reported rib fracture was related to a 5.4-fold raised risk of a future rib fracture, while the risk of any future clinical fracture was 2.4-fold increased (Sajjan et al. 2012).
In patients with osteoporosis or those receiving a high-dose steroid therapy, even severe cough can result in rib fractures (Katrancioglu et al. 2015). According to the findings of Pluskiewicz et al., a prior osteoporotic fracture may be the main prognostic factor for the incidence of future fractures (Pluskiewicz et al. 2011). According to Neuerburg et al., osteoporosis is evident in most men over 60 (59 %) and women over 50 (56.2 %), who present to hospital with an osseous fracture. As a consequence, osteoporosis should generally be evaluated in the management of trauma patients with the diagnosis of a fracture over 60, respectively, 50 years of age and treated if necessary to prevent future fractures (Neuerburg et al. 2015). Furthermore, the consideration whether the trauma mechanism was adequately to result in the fracture the patient presents could be a sign for a possibly impaired bone density (Sajjan et al. 2012; Wuermser et al. 2011; Palvanen et al. 2004; Barrett-Connor et al. 2010; Watts et al. 2008; Mirvis et al. 2014, p. 163).
4 Radiologic Equipment and Techniques
4.1 Ultrasound
Portable, non-invasive ultrasound (US) diagnostic can be performed at any time, in any place and on any patient and is thus very suitable for emergency situations (Reissig et al. 2011). The focused assessment with sonography for trauma (FAST), a scan for free abdominal fluid, is most widely accepted (Fleming et al. 2012; Rothlin et al. 1993; Chung et al. 2014) and was extended (EFAST) to detect pleural pathologies (Fig. 2) (Kirkpatrick et al. 2004; Matsushima and Frankel 2011).
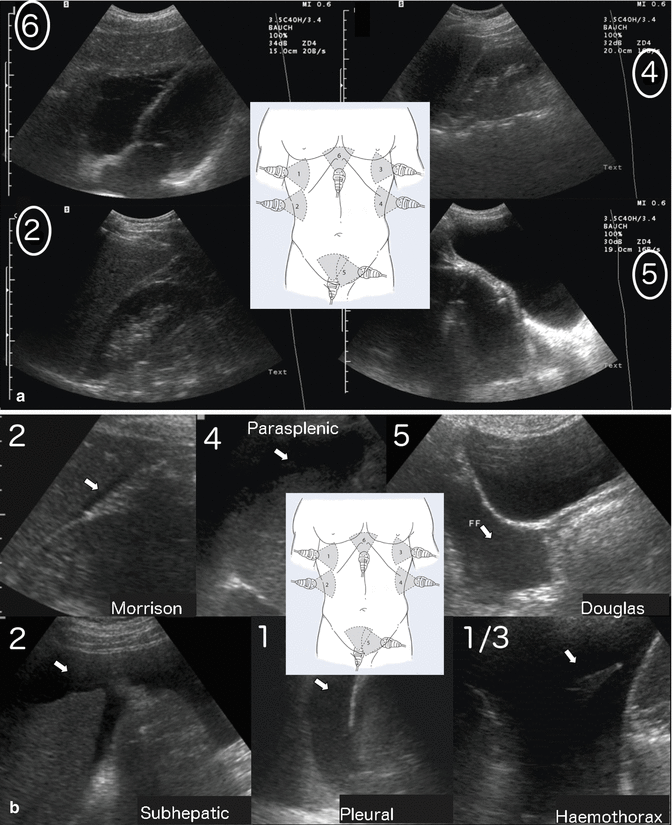
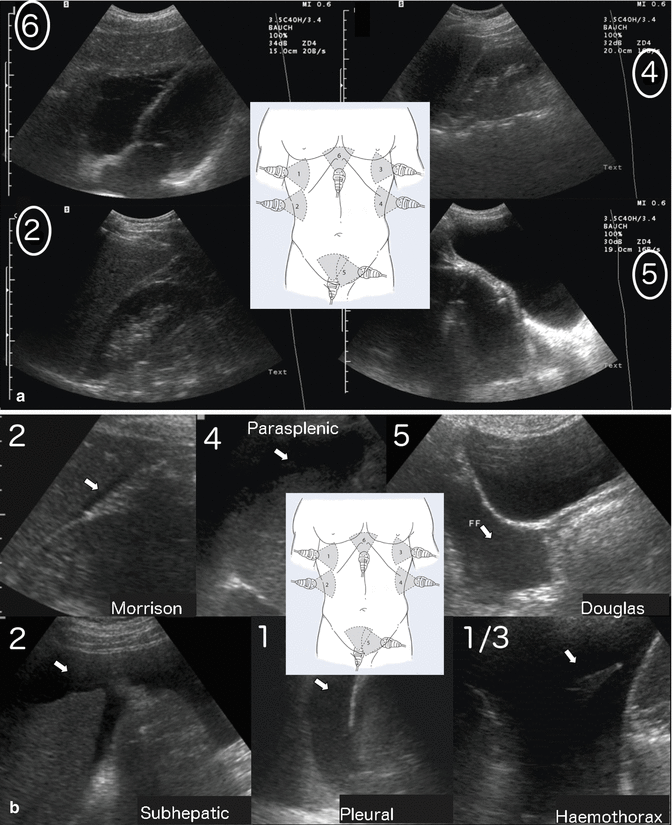
Fig. 2
EFAST: rapid ultrasound scan for free intra-abdominal and intrapleural fluids. The numbers on the left side of the top of the US pictures correspond to the positions of the Ultrasound Transducer as depicted in the central illustration. (a) Normal findings of the heart (6), the Morrison (2) pouch, the Koller (4) pouch and the Douglas space (5), here in sagittal orientation. (b) Corresponding labelling of examples with findings of free abdominal fluid as marked by arrows (central illustration from springer, der anaesthesist)
Although some of these conditions are beyond the scope of EFAST, multiple thoracic medical conditions, such as pulmonary embolism, pneumothorax, haemothorax, pleural effusion, cardiac injuries and rib fractures, and sometimes even pneumonia can be detected with the help of US (Reissig et al. 2011). It may be surprising that US has proven to be even superior to supine chest radiography in ruling out suspected pneumothorax (sensitivity 48.8 % vs. 20.9 %; specificity 99.6 % vs. 98.7 %) (Kirkpatrick et al. 2004). This was confirmed for sensitivity in a meta-analysis from 2010 (86–98 % vs. 28–75 %), while both modalities where comparably specific (97–100 % vs. 100 %) (Wilkerson and Stone 2010). US may even be superior to chest radiography in the detection of rib fractures, but so far clear evidence is missing and further studies with a well-established gold standard have yet to be executed (Lalande and Wylie 2014).
4.2 Chest Radiography
Chest radiography is often a common part of the standard trauma workup, even if computed tomography (CT) is to be performed later on. Even mobile C-arm fluoroscopy or radiography can verify tube and line positioning (Fig. 3). In many cases pneumothorax, tension pneumothorax, haemothorax, extrapleural haematoma and injuries of the aorta (Fig. 4), heart, trachea, oesophagus, great thoracic vessels, bony chest wall and lungs can be detected, helping with patient triage without losing much time during initial emergency room care. However, in a trauma setting, patients are seldom able to stand and perform a full inspiration resulting in both limitation of chest radiographs to AP direction and impaired significance to additional motion artefacts and overlying materials (Chung et al. 2014; Kaewlai et al. 2008, pp. 1556–1557). Therefore, it is not surprising that about half of the pneumothoraces and rib fractures detected by CT are occult on AP chest radiography. Consequently, experts advise against over-reliance on chest radiography in patients with high-energy blunt thorax trauma (Ball et al. 2005; Barrios et al. 2010; Chung et al. 2014). In summary it can be stated that, although chest radiography is less accurate in case of blunt chest trauma than CT, it can still provide important information and is often faster available in the trauma bay. Thus, it can be concluded that the utilisation of chest radiography is justified in case of minor trauma or in case of high-energy trauma if CT is not available in time.
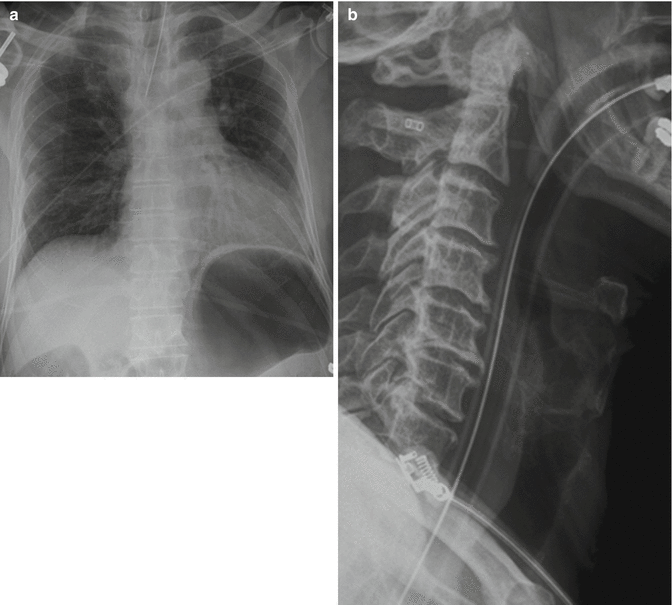
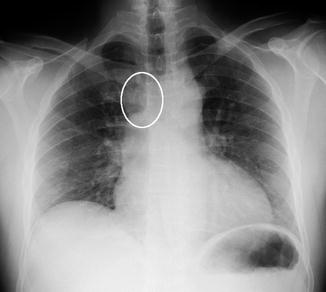
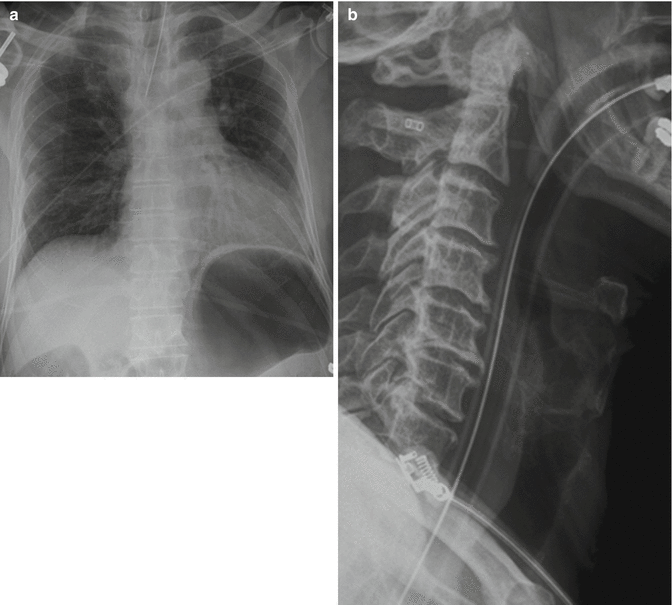
Fig. 3
Misplaced endotracheal tube. Notice huge amount of air in projection of the stomach as well as the endotracheal tube crossing the left tracheal border in AP chest projection (a) and the sagittal neck view (b) proofing the malpositioning
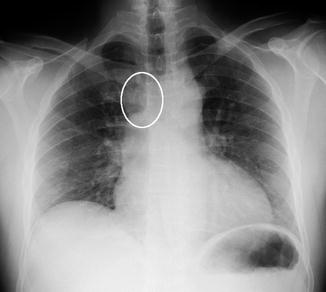
Fig. 4
Unrecognised Stanford A dissection of the aorta with secondary fatal haemopericardium. Chest radiography (p.a.) of a 46-year-old patient with mild pain after weight lifting 4 h ago. The thickening and hampered transmission of the anterior mediastinum as well as the buckling in projection of the border of the aortic root (white circle) was missed. As no apical cap sign was visible and there was only mild pain, the patient went home, where he died another 4 h later. CT was not performed. Autopsy revealed a massive aortic dissection
If CT is available for high-risk patients, the CT scout (i.e. topogram or scanogram) (Fig. 5) provides relevant information (about conditions that require intervention before CT) comparable to radiography but with less total time consumption (Wirth et al. 2009). However, the appropriate modality to exclude or diagnose relevant findings like pneumothorax may depend on various circumstances, such as urgency, patient condition, local conditions and others (Ball et al. 2005; Barrios et al. 2010; Chung et al. 2014; Kaewlai et al. 2008, pp. 1556–1557; Henry et al. 2014; Traub et al. 2007).
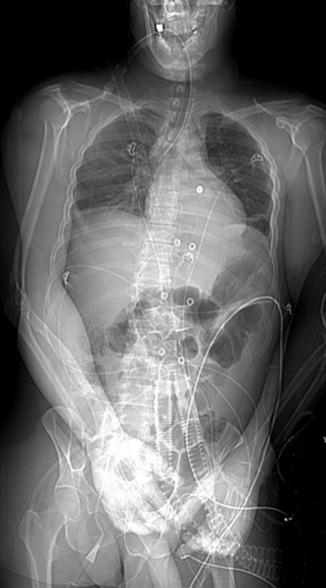
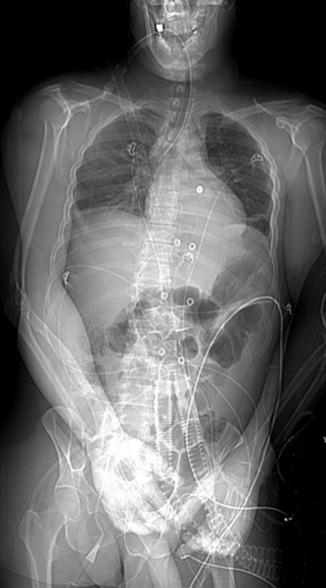
Fig. 5
Topogram of a polytrauma patient for planning the exact positioning of CT scanning. Additionally, it holds relevant information about serious patient conditions and may give hints for extension of scan regions
4.3 Computed Tomography
The invention of CT and CT angiography has revolutionised the blunt trauma management, allowing rapid diagnosis of complex injuries and consequently early therapeutic management like embolisation of arterial bleeding and utilisation of stents in case of vascular dissection or transection (Tillou et al. 2009). In trauma centres CT is usually 24/7 available and able to provide fast and high-quality whole body 3D cross-sectional imaging (WB-CT). This results in the highest sensitivity (80–100 %) (Magu et al. 2009; Bier et al. 2015) among the techniques for imaging of rib fractures. Furthermore, it provides information of associated injuries. According to Traub et al., CT can reveal significant injury in about 38 % of patients with bland initial radiographs (including fractures of ribs (14.9 %, p < 0.001), sternums (7.1 %, p < 0.001), scapulae (4.9 %, p = 0.016) and vertebrae (15.5 %, p < 0.001)) or can possibly exclude suspected injury in patients allowing an earlier discharge (Traub et al. 2007). CT can even be performed during cardiopulmonary resuscitation (Fig. 6) (Wirth et al. 2009). Counterarguments for the utilisation of CT may be, depending on the particular setting, higher time consumption compared to radiography, higher radiation exposure (chest: average effective CT dose = 2.86–7.4 mSv vs. 0.1 mSv) and higher costs (Mettler et al. 2008; Tonkopi and Ross 2016).
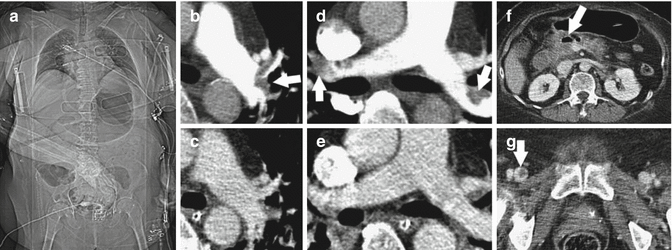
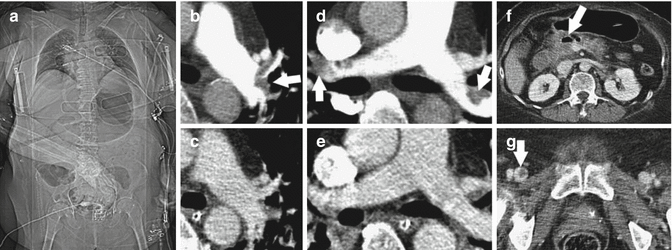
Fig. 6
CT images of a 62-year-old obese female patient with LUCAS baseplate. The patient was admitted under mechanical CPR with LUCAS. Because she did not fit into the bore of the CT gantry with the whole LUCAS system, the compression unit was lifted off for each CT data acquisition. (a) Planning scout for chest/abdomen and pelvis. (b, d) Thorax: CT revealed PE obstructing lobar arteries on both sides (arrows, 50 s delay). (f, g) Abdomen/pelvis: Because images of the abdomen/pelvis with a delay of 120 s had insufficient venous contrast enhancement (not shown), the CT acquisition was repeated with a delay of 210 s. Images depicted free peripancreatical air surrounded by small areas of contrast media (arrow in f) as well as thrombosis of the right deep femoral vein (arrow in g). (c, e) Control study of the thorax after return of spontaneous circulation confirmed successful fibrinolysis. Note the differing distribution of contrast media even though all scan parameters were identical to those of the first study shown in b and d
As specified in the section “Epidemiology and Aetiology”, a major part of trauma victims are of a young age, where the discussion about potentially harmful radiation effects is particularly relevant. This discussion should consider that it is known that we are able to save one-fourth of polytrauma patient’s lives with the utilisation of whole body CT when comparing with ‘dose saving’ organ-based CT imaging (Huber-Wagner et al. 2009). Thus, it is of outmost importance to decide very quickly whether acute life-threatening conditions are given in a patient or not. In cases where this is given, the risk of harmful application of radiation can be expected to be far beyond the possible benefit. In addition, a polytrauma is a once in a lifetime experience for most patients – a situation that decides about living or dying. However, for children and young women, it should remain a very carefully taken case-to-case decision, depending on the very individual constellation (Mueck et al. 2016).
CT characterises pleural fluids with determination of their attenuation values, where fresh haemorrhage may have an attenuation as low as 20/30–45 Hounsfield units (HU) (Mirvis et al. 2014, p. 178; Feeman 2010). During the clotting process, the density of blood increases to up to 50–90 HU (Mirvis et al. 2014, p. 178). To distinguish blood from other fluids, the reader should routinely perform a measurement of pleural fluid attenuation in chest trauma patients (Kaewlai et al. 2008, p. 1558; Feeman 2010; Chung et al. 2014; Brink et al. 2008; Traub et al. 2007; Tillou et al. 2009; Mirvis et al. 2014, p. 178).
While the utilisation of CT is discussed contentiously in the literature, many authors consider high-energy mechanisms, chest wall tenderness, reduced air entry, abnormal respiratory effort, abnormal chest radiographs, reduced consciousness and being intubated criteria for a selective computed tomography scan (Traub et al. 2007; Tillou et al. 2009; Brink et al. 2008; Kaewlai et al. 2008, pp. 1556–1569; Feeman 2010; Chung et al. 2014; Henry et al. 2014). In a well-structured department, a standard whole body reconstruction set of CT images can be provided in about 20 min, including an in-room time for the patient of less than 10 min. Thus, up to six patients may be served per CT scanner and per hour in a mass casualty incident (Mueck et al. 2016; Wirth 2014).
4.4 Magnetic Resonance Imaging
In contrast to ultrasound, computed tomography and radiography, magnetic resonance imaging (MRI) is highly time consuming (studies may take up to 1 h).
Patients have to remain motionless and treatment options are limited during that period. Thus, the significance of MRI in the emergency workup of severely injured trauma patients is very questionable. Nevertheless, it can be used as a problem-solving tool in case of suspected heart, pericardial and spinal injuries/acute neurologic deficit or other situations especially in pregnant women and paediatric patients (Chung et al. 2014).
For the decision for or against MRI in an emergency situation, it may be helpful to think about the following aspects:
- 1.
Is MRI the only available modality to confirm or rule out the suspected injury, or in case of approximately equal indication, may MRI be favourable due to other reasons (e.g. radiation exposure to paediatric patients)?
- 2.
Would the expected findings lead to alternation of patient management?
- 3.
Would one possible finding alter the patient management to an immediate procedure that is necessary to prevent irreversible harm (e.g. refixation of damaged articular cartilage)?
If all these questions can be answered with ‘yes’ and thus the decision is made to execute an MRI scan, the sequences should be reduced to what is necessary to help solve the clinical problem in order to save time (Wirth 2016, pp. 54–55). Conclusively, it must be said that especially chest trauma patients have a tendency to move (e.g. due to pain) and thus have to be highly compliant in addition to the aspects above to make an MRI feasible.
5 Soft Tissue Contusion and Subcutaneous Emphysema
5.1 Epidemiology of Soft Tissue Contusion and Subcutaneous Emphysema
Blunt chest trauma may result in soft tissue injuries, such as muscle tears, haematoma, cutaneous abrasions, burns, ecchymosis or lacerations (Schnyder and Wintermark 2000, p. 9). These soft tissue injuries may be markers for more severe associated injuries. Lung contusion (Fig. 7) is a frequent injury among blunt chest trauma patients (17–70 %) and serves as a predictor of occult pneumothorax (OR = 3.25; 95 % CI = 1.62–6.54; p = 0.001) (Ball et al. 2005; Skinner et al. 2015; Mirvis et al. 2014, pp. 172–177; Kaewlai et al. 2008, p. 1558). Subcutaneous emphysema in patients after blunt chest trauma (approximately in 15 % of cases) also serves as a marker of occult pneumothorax with a crude risk ratio of 6.78 (95 % CI 2.46–18.66; p < 0.001) and a specificity of 98 % (Ball et al. 2005; Schnyder and Wintermark 2000, pp. 9–10). Ball et al. found subcutaneous emphysema to be present in 7 % of a population of 338 trauma patients with an ISS ≥12 (Ball et al. 2005). Turkalj et al. detected subcutaneous emphysema in 34.4 % out of 61 blunt chest trauma patients (2014).
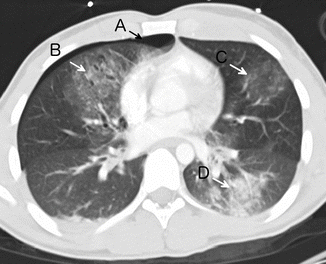
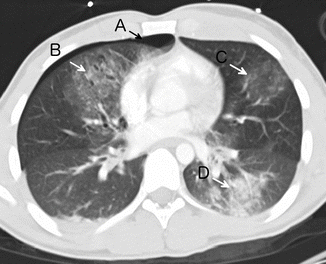
Fig. 7
Pneumothorax (A), pulmonary laceration (B), pulmonary contusion (C) and aspiration (D). Axial image of a contrast-enhanced CT of the chest, lung window settings
The presence of subcutaneous emphysema should also raise concern about osseous injuries. From 18.4 % to over 75 % of patients with subcutaneous emphysema have at least one associated rib fracture (Turkalj et al. 2014). The mortality rates of patients with soft tissue injuries mainly depend on associated injuries. For example, the simultaneous incidence of lung contusion and flail chest more than doubles the mortality from 16 to 42 % (Clark et al. 1988). Flail chest is a clinical finding based on osseous fractures and the breathing mechanism. If three or more contiguous ribs are broken in at least two places, they create a flail segment that moves paradoxically to the remainder of the chest wall while the patient is breathing. Flail chest usually occurs in the anterior and anterolateral regions of the middle and lower ribs, which are less protected by musculature and surrounding tissue. Compliance of the ribs is equally important in the incurrence of flail chest as the force of impact. Severe pain and respiratory insufficiency, due to a decreased vital capacity and ineffective ventilation, may possibly be the consequences. While fractures can be detected by imaging, the paradoxical motion is usually proven by clinical examination (Kaewlai et al. 2008, p. 1567; Bier et al. 2015; Sirmali et al. 2003).
5.2 Biomechanics of Soft Tissue Contusion and Subcutaneous Emphysema
Soft tissue contusion may lead to emphysema, swelling, pain and hematoma from arterial or venous vessels. While arterial hematoma can enlarge rapidly, venous haematoma is often self-limiting and enlarges rather slowly. In this context, patients under anticoagulation therapy are more likely to develop complications (75 % vs. 41 %) (Ball et al. 2005) with a complication OR of 2.3 (95 % CI 1.1–4.8; p < 0.05) calculated for the pre-injury use of anticoagulants (Mirvis et al. 2014, p. 179; Battle et al. 2013). Fractured ribs may possibly lead to subcutaneous emphysema and pneumothorax due to air leakage by tearing the pleura and pulmonary parenchyma. Tracheobronchial injuries can induce pneumomediastinum and subcutaneous emphysema as well. According to the fact that adipose tissue is not compartmentalised, subcutaneous emphysema of thoracic origin can possibly extend to the abdomen, scrotum, limbs, mediastinum, retroperitoneum or face/skull (Fig. 8) (Schnyder and Wintermark 2000, p. 10).
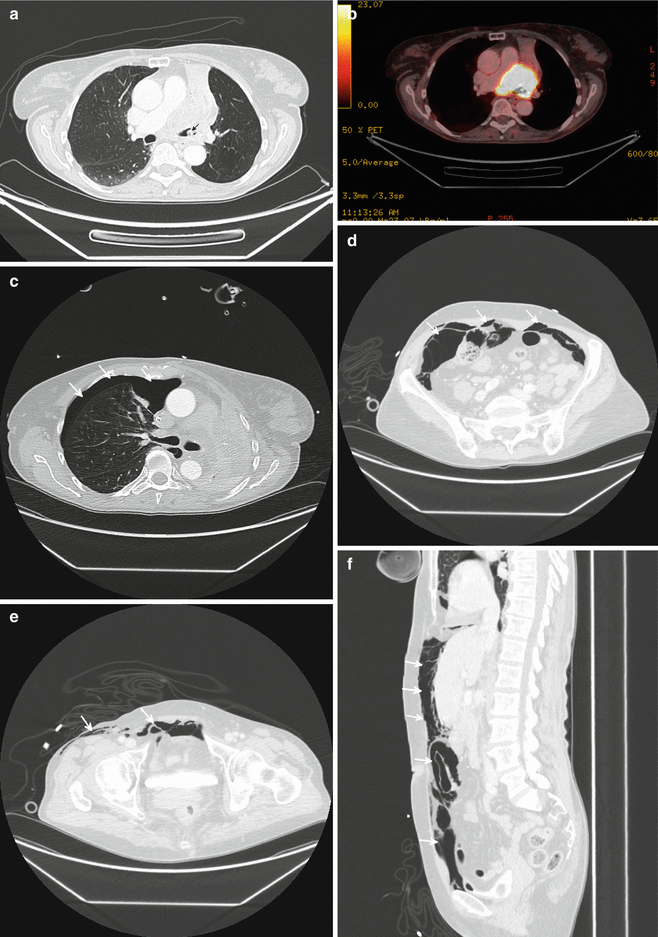
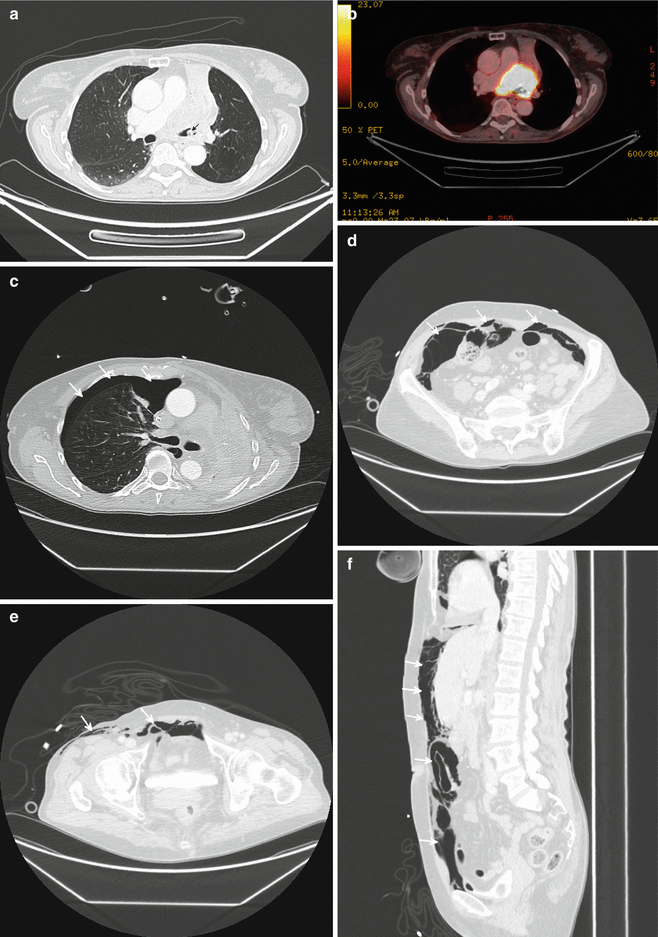
Fig. 8
Tension pneumothorax with air distribution through the abdomen extension to subcutaneous spaces. Bronchial carinoma restricting the left main bronchus (arrow) of a 65-year-old female patient in CE axial CT (a) and FDG-PET-CT (b). One week later the same patient presents with complete atelectasis of the left lung (secondary to complete restriction of the left main bronchus by bronchial carcinoma) and tension pneumothorax on the right side (c, arrows), air distribution to the abdomen (d, arrows) and pelvis (e, right arrow). The sagittal view (f) demonstrates the whole extension of the abdominal emphysema portion feeding subcutaneous compartment through inguinal pathways (e, left arrow)
5.3 Main Part
Minor soft tissue contusion is usually occult on plain chest radiography. Especially, in obese patients, a haematoma or emphysema can be obscured. Increased density and asymmetry of the soft tissue are hints to a contusion (Mirvis et al. 2014, p. 179). Even small haematoma, appearing unimpressive, can be a marker for serious underlying injury. In up to 32 % of the cases, subcutaneous emphysema can be the first sign of an occult pneumothorax (Ball et al. 2005) which itself might become life threatening, especially during aeromedical transport at altitude or when positive pressure mechanical ventilation is used (Kirkpatrick et al. 2004). As Rothlin et al. detected subcutaneous emphysema by US in 17 % of 70 patients with thoracic pathologic entities, US may also be a reasonable screening tool for this condition (1993).
Tracheobronchial injuries are only found in 1–2 % of blunt chest trauma room patients (Welter and Hoffmann 2013, p. 113) because many patients will already have died from associated injuries before reaching the hospital. The overall estimated mortality is 30 % (Burke 1962; Chesterman and Satsangi 1966; Ecker et al. 1971; Kirsh et al. 1976). Deep cervical emphysema (100 %), paratracheal air (94 %) and pneumothorax (36 %) are radiological manifestations of possible tracheobronchial (Fig. 9) or oesophageal (Fig. 10) injuries. Tracheobronchial ruptures typically proceed transversally, parallel to the cartilage rings (74 %) (Welter and Hoffmann 2013, p. 114) and are usually located within a distance of 2.5 cm from the carina (Welter and Hoffmann 2013, p. 114; Kaewlai et al. 2008, pp. 1559–1561). Consequently, the region around the carina should be a particular reading focus. A persistent pneumothorax in a patient with tube placement and suction indicates a possible injury of the tracheobronchial tree, which should be investigated. The ‘fallen lung’sign (Fig. 11), where a lung posterolaterally falls away from the hilum if the patient is supine and inferiorly if the patient is upright, indicates a complete ipsilateral transection of a main bronchus with its accompanying blood vessels. In such cases pneumomediastinum and cervical subcutaneous emphysema are typical additional findings (Ball et al. 2005; Kaewlai et al. 2008, pp. 1559–1561). Furthermore, alveolar ruptures can also produce a pneumomediastinum (Fig. 12) via the Macklin effect (Fig. 13), first described by Macklin and Macklin in 1944, where air dissects into the mediastinum along the bronchovascular sheaths. Streaks of air can be seen along the bronchovascular bundles. Most cases of tracheal laceration can be identified with CT (70–100 %) (Kaewlai et al. 2008, pp. 1559–1561; Welter and Hoffmann 2013, p. 114). However, the diagnosis of a tracheobronchial tree injury should be confirmed by bronchoscopy. With respect to outcome, airway obstruction, bronchiectasis, pneumonia, abscess and empyema are potential complications (Kaewlai et al. 2008, pp. 1559–1561; Welter and Hoffmann 2013, p. 114).
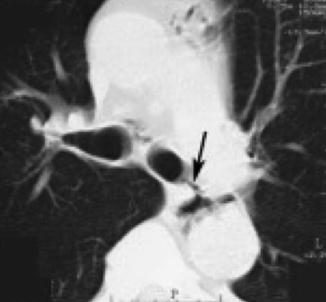
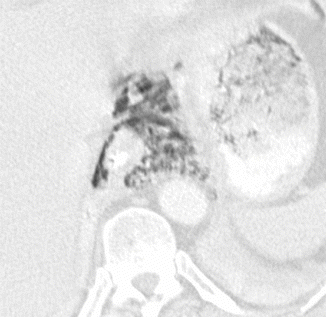
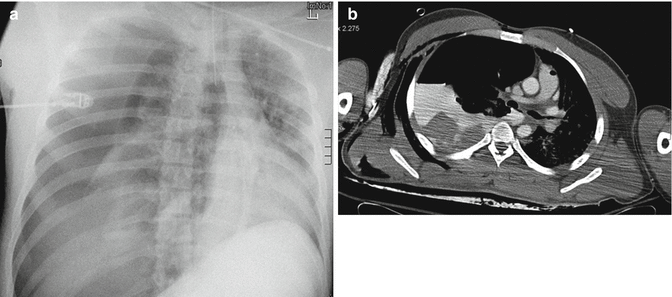
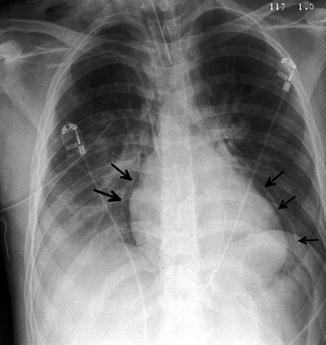
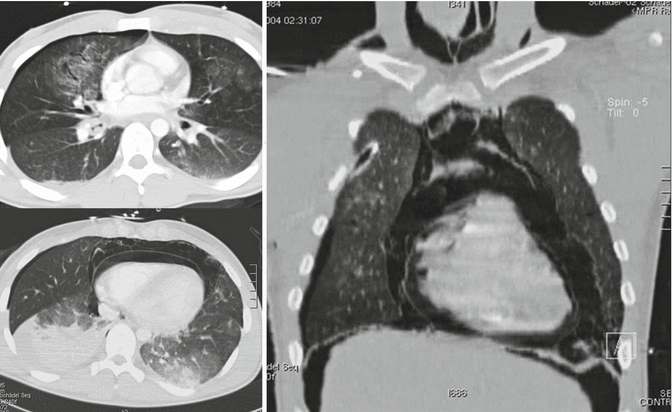
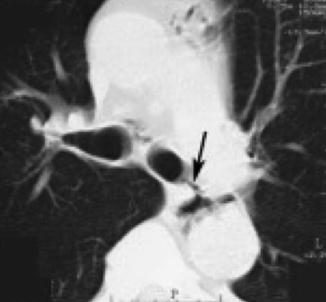
Fig. 9
Bronchial rupture (arrow). Axial CT, lung window
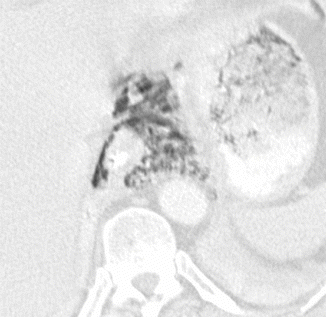
Fig. 10
Oesophagus rupture. Axial CT, lung window
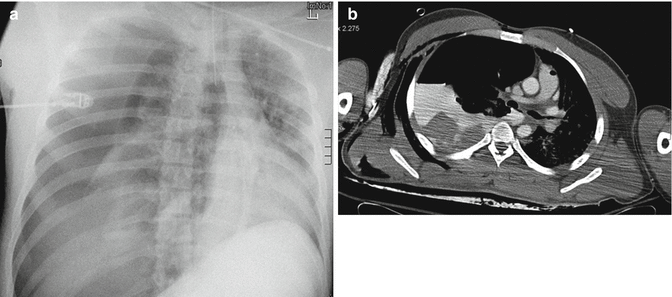
Fig. 11
Fallen lung sign. Polytraumatised patient of unknown age. AP chest radiogram directly after admission (a). Note the dorsal position of the lung and the massive tension component to the left side. Axial CE CT with soft tissue window settings (b) proofing tension haemopneumothorax, massive bleeding due to complete avulsion of the right hilus and subcutaneous emphysema. Efforts had to be terminated
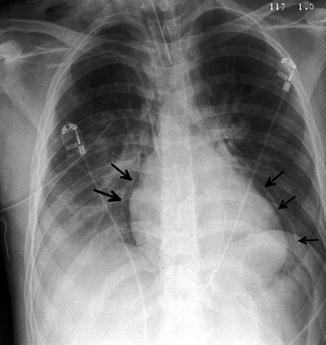
Fig. 12
Pneumomediastinum in AP chest radiogram (arrows)
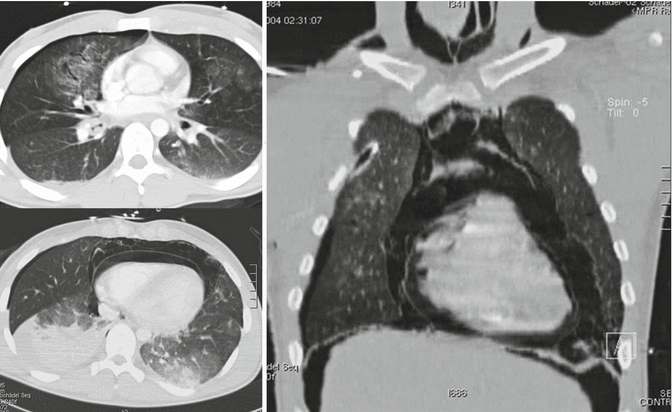
Fig. 13
Macklin effect. Axial and frontal CT reformations, lung window. A rib fracture (not shown) induced distal pulmonary laceration with air dissecting along the bronchovascular sheaths into the mediastinum. Same patient as Fig. 12
A high-energy deceleration during a car accident may result in a hematoma of the lower neck and central chest in approximately 16 % of the cases. The presence of such a haematoma, called ‘seatbelt sign’, may be an indication for neck CT with intravenous contrast to evaluate possible cervical blood vessel injuries (carotid artery injuries 3 %) (Mirvis et al. 2014, p. 179; Rozycki et al. 2002). Vertebral artery injuries occur in about 0.5 % of blunt trauma patients (Nedeltchev and Baumgartner 2005). Especially the combination of a ‘seatbelt sign’ with a Glasgow Coma Scale <14, an ISS >16 and a fracture of the clavicle and/or first rib should raise particular concern for vascular injuries (Mirvis et al. 2014, pp. 179–183; Rozycki et al. 2002).
5.4 Summary
Even small and unimpressive soft tissue injuries and subcutaneous emphysema can be a marker for serious underlying injuries (e.g. occult pneumothorax in 32 % of patients with subcutaneous emphysema).
Deep cervical emphysema, paratracheal air, pneumomediastinum and pneumothorax (especially persisting pneumothorax after chest tube placement) are markers for tracheobronchial injuries. These are highly life-threatening conditions (mortality rate 30 %) that mostly appear within a distance of 2.5 cm from the carina.
Tracheal lacerations can be identified with CT in 70–100 % of the cases. However, diagnosis should be confirmed by bronchoscopy.
A ‘seatbelt sign’ indicates possible cervical blood vessel injuries, which should be ruled out by contrast-enhanced neck CT.
6 Rib Fractures
6.1 Epidemiology of Rib Fractures
Rib fractures are the most common skeletal injury in blunt chest trauma patients. They appear in about 50 % of patients after such an event and in about 10 % of patients after trauma in general (Ziegler and Agarwal 1994; Mirvis et al. 2014, pp. 179–182; Shorr et al. 1987; Henry et al. 2014). Fractures of the first two ribs are associated with thoracic aorta and other thoracic vascular (about 13 %) injuries (Clark et al. 1988; Magu et al. 2009). According to Shalhub et al., arterial haemorrhage after blunt chest trauma is associated with rib fractures in up to 44 % of the cases (Shalhub et al. 2011). Up to 50 % of rib fractures are multiple and, out of these, 8 % are bilateral (Shorr et al. 1987).
The rate of complications, such as pneumothorax, haemothorax, pulmonary contusion, flail chest, pneumonia and atelectasis, rises with the number of fractured ribs (16.4 % for 1–2 fractures, 33.6 % for 3–5 fractures, 52.7 % for 6 or more fractures), according to a study performed by Sirmali et al. (2003).
Flail chest is present in up to 7 % of blunt trauma patients. This condition may induce major haemorrhage or seriously impaired ventilation, and mortality rates – although varying in literature – can be as high as 33 % (Clark et al. 1988; Mirvis et al. 2014, pp. 179–183; Sirmali et al. 2003; Veysi et al. 2003; Kaewlai et al. 2008, p. 1567). Scapula fractures, when detected, should raise awareness of rib injuries, because their presence increases the likelihood of rib fractures (relative risk 3.1; p < 0.01) and flail chest (relative risk 8.8; p < 0.001) (Weening et al. 2005). Moreover, rib fractures are predictors for occult pneumothorax (OR = 2.65; 95 % CI = 1.34–5.24; p = 0.005) (Ball et al. 2005). The overall mortality rate for patients presenting to hospital with rib fractures is approximately 6 % (Sirmali et al. 2003).
6.2 Biomechanics of Rib Fractures
While rib fractures among young adults mainly result from high-energetic trauma, they are often caused by falls from standing height and everyday activities in older adults. For this second group, fall preventing strategies are appropriate and suitable to lower the number of future incidents. Due to the chest wall architecture and the decreased support from muscles and other soft tissue, ribs fracture most often at the lateral aspects (Mirvis et al. 2014, pp. 179–183). The pain experienced from fractures of the bony chest wall, as well as combined direct lung injuries (e.g. pulmonary contusion) or haemorrhage (e.g. from intercostal arteries) may compromise ventilation. These injuries induce shunting and dead space ventilation, which additionally impair oxygenation (Mancini et al. 2014).
6.3 Main Part
Blunt chest trauma is regularly associated with fractures of the bony thorax, which reflect a large amount of energy having been imparted to the chest (Shorr et al. 1987; Henry et al. 2014). Nevertheless, we have to keep in mind that generally and particularly in children, serious trauma can be present in the absence of rib fractures. Skinner et al. investigated the morbidity of children and adults after blunt chest trauma. Compared to adults, children suffered less frequently from rib fractures (20.2 % vs. 42 %) and flail chest (2.4 % vs. 26.3 %), but more often from pulmonary contusion (79.8 % vs. 65.6 %) or associated head injuries (61.9 % vs. 42.3 %) (Skinner et al. 2015).
Isolated rib fractures are rarely life threatening, but bilateral or multiple rib fractures are indicators of severe chest injury with significant morbidity and mortality (Ziegler and Agarwal 1994; Kaewlai et al. 2008, p. 1567). Rib fractures may affect ventilation through laceration of underlying lung parenchyma, paradoxical chest wall motion and pain (Shorr et al. 1987; Henry et al. 2014). A fracture of the first three ribs is an indicator of high-energy trauma since these ribs are short, broad and well-protected by the scapula, clavicles and musculature. The brachial plexus and subclavian blood vessels are located close by and may possibly be injured. On the other hand, fractures of the lower three ribs are associated with trauma of the liver, spleen (Fig. 14), kidneys and lungs (Kaewlai et al. 2008, p. 1567). According to a study performed by Lee et al. including 105,683 trauma patients having been admitted to hospitals in the United States during the years 1984–1986, the presence of three or more rib fractures increased the relative risk of haemothorax/pneumothorax (18.4), spleen injury (6.2) and liver injury (3.6) (Lee et al. 1990). Therefore, rib fractures might be indicators of coexisting thoracic or abdominal trauma (Ziegler and Agarwal 1994; Kaewlai et al. 2008, p. 1567). US and, if the circumstances require, abdominal imaging should be performed to rule out associated injuries if one of the lower three ribs is fractured. If the trauma has not significantly affected the lower three ribs, abdomen or pelvis, a suspicious result in physical exam, laboratory studies or urinalysis will be required to justify the use of advanced imaging of this region, as stated in current guidelines (Henry et al. 2014).
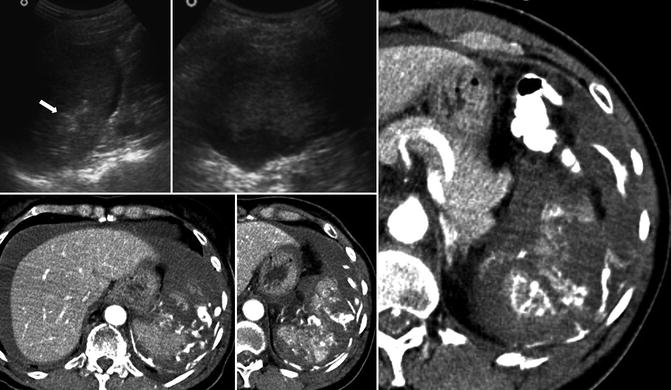
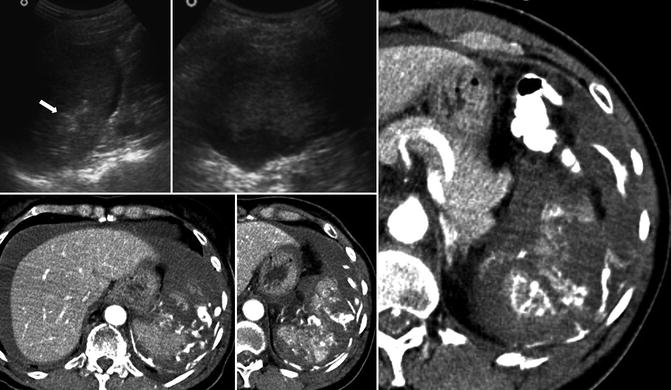
Fig. 14
Splenic haemorrhage (arrow) induced by laceration secondary to rib fracture in US and axial CT
Sirmali et al. developed a rule of thumb for blunt chest trauma patients: according to their findings, three or more rib fractures are an indication for the hospitalisation of patients. Elderly with six or more fractured ribs should be treated in intensive care units (Sirmali et al. 2003). Ball et al. found that 59 % of a group of trauma patients in whom an occult pneumothorax (Fig. 15) was evident had coexisting rib fractures, while only 27 % of patients without pneumothorax had rib fractures (Ball et al. 2005). Bulger et al. reported that, according to their findings, the clinician should pay close attention to the number of rib fractures to stratify the expected mortality and morbidity, as the odds ratio rose by 1.19 per fractured rib for death and by 1.16 per fractured rib for pneumonia (Bulger et al. 2000). Pain control is a vital part in patient management to prevent respiratory depression, as well as the development of atelectasis and pulmonary infection. If indicated, epidural analgesia may be superior to intravenous and oral analgesia. Pain from multiple rib fractures can also inhibit weaning a patient from mechanical ventilation (Bulger et al. 2000; Sirmali et al. 2003).
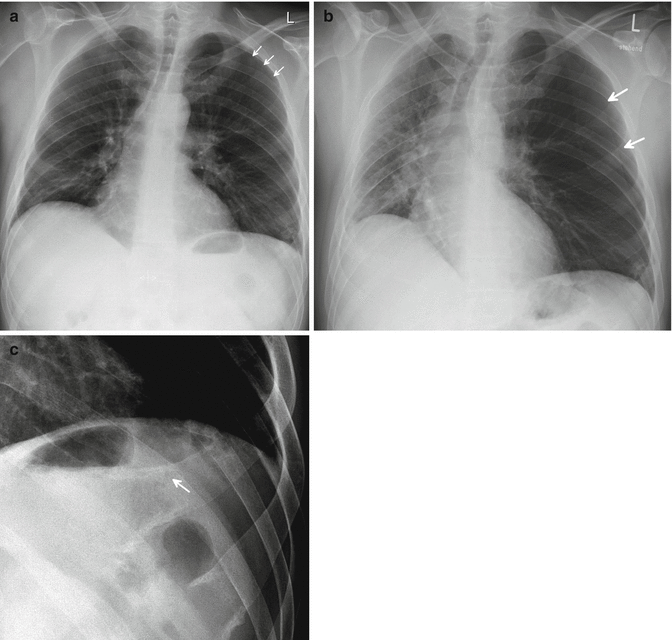
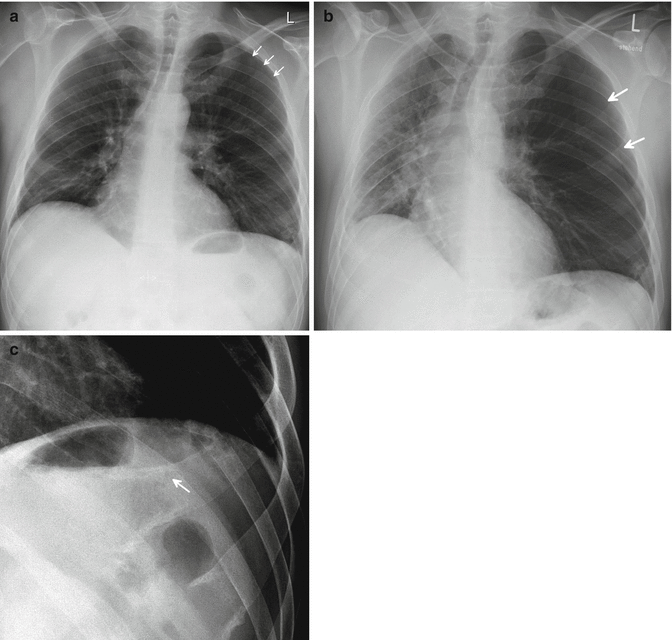
Fig. 15
Pneumothorax extension over the course of time. Initial chest radiogram of a patient with hardly visible pneumothorax (a, arrows). The patient developed a serious mediastinal shift indicating a tension component with now clearly visible border of the pneumothorax (b, arrows). A cutout from a lower rib-targeted radiogram revealed a rib fracture (c, arrow) as the most reasonable cause of the pneumothorax
The standard initial, and often the only required, imaging test for disclosing rib fractures in patients after minor trauma is chest radiography in two planes (Henry et al. 2014). It is crucial to weigh up the pros and cons of the available radiological equipment in terms of additional information and specificity against time, radiation burden and costs. Although rib fractures can lead to significant morbidity, it is more important to detect associated complications such as haemothorax (Fig. 16), pneumothorax, pulmonary contusion, flail chest, atelectasis, cardiovascular injury and injuries to solid and hollow abdominal organs. According to the American College of Radiology, chest CT without or with contrast is appropriate for patients with rib or sternal fractures plus at least one of these associated complications identified by clinical examination or other imaging (Henry et al. 2014). When isolated, single fractures, multiple fractures or non-acute fractures have a relatively low morbidity and mortality and are not an indication for chest CT unless malignancy is suspected in the aetiology, and a gain of additional information is expected. Failure to identify isolated rib fractures in uncomplicated cases does not automatically change outcome or patient management. Thus, there is little data to support the use of advanced imaging, even if CT is superior to radiography for detection of these fractures because of its sensitivity of 80–100 % (Henry et al. 2014; Traub et al. 2007; Magu et al. 2009; Bier et al. 2015). However, physicians should always keep in mind that only up to 50 % of rib fractures can be detected with chest radiography (Bulger et al. 2000). CT provides significantly more information than chest radiography alone if the patient shows chest wall tenderness, abnormal respiratory effort or reduced air entry. Traub et al. suggest that intubated patients should receive a routine CT chest scan at their first evaluation, as the survey of clinical information is restricted. For a final decision about the use of chest CT, the clinician should consider all clinical features and prior radiological information (Traub et al. 2007).
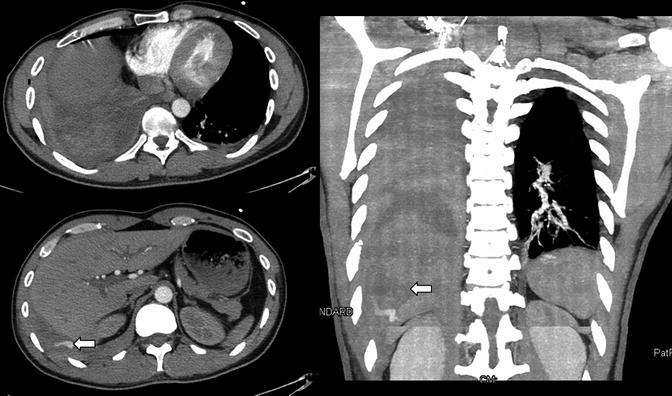
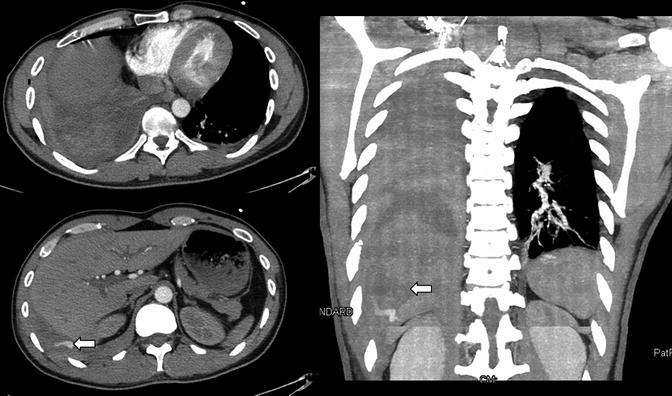
Fig. 16
Active arterial bleeding secondary to a rib fracture, which itself is not shown. The axial images of the chest CT in arterial phase reveal active contrast agent extravasation (arrows) leading to a haemothorax of the right side (left axial slices; right coronal MIP)
Fractured ribs may possibly produce a delayed haemothorax (DHX) due to intercostal artery haemorrhage (Fig. 16). DHX is described to appear in 2.1–7.4 % of blunt chest trauma patients. Curfman et al. reported a case of a patient who lost 6500 ml of blood out of one single intercostal artery, 10 days after a traumatic rib fracture while still being in the hospital (Curfman et al. 2015). Pleura-covered parts of the lung may extrude through the chest wall at a locus of traumatic defect, like a rib fracture, causing a traumatic lung herniation. Treatment should be taken into consideration before intubation because positive pressure ventilation can increase this condition (Kaewlai et al. 2008, p. 1567). In case of pneumothorax and haemothorax caused by rib fractures, tube thoracostomy can be sufficient as treatment as the pressure from the expanding lungs may possibly stop the bleeding from small loci. Bleeding from major intrathoracic vessels or intercostal arteries usually requires balloon occlusion and thoracotomy (Sirmali et al. 2003).
The infantile chest wall is more elastic than the adult chest wall.
In children, ribs consequently need a higher level of energy transferred to the thorax to fracture. Therefore, children with rib fractures suffer more often from associated haemothorax, pneumothorax, brain injuries, spleen injuries and liver injuries than adults with rib fractures (Kessel et al. 2014). Bulger et al. reported a doubled mortality and thoracic morbidity after blunt chest trauma for elderly patients (65 years and older) in comparison to younger patients, regardless of similar injury patterns and injury severity (Bulger et al. 2000).
Detecting rib fractures in CT can be a quite challenging task. Each rib has a complex shape and extends across numerous sections, while twisting along its longitudinal axis. The sequential rib-by-rib evaluation traversing the whole thorax is tedious and time consuming. Furthermore, fractures can be hard to identify, especially if they are parallel to the section or not dislocated (Ringl et al. 2015). Coronal imaging is more sensitive to detect fractures parallel to axial sections (Cho et al. 2012). Fractures of the ribs are periodically overlooked in radiologic imaging and their detection can be of medical-legal relevance. The major part of the rib fractures missed in CT is located at the anterior arc and over 50 % of missed rib fractures have a buckled shape. They appear equally on both sides of the thoracic cage. Frequently, missed fractures are on the same rib or the neighbouring rib of fractures that have been detected (Cho et al. 2012; Ringl et al. 2015).
Due to often being associated with more severe injuries, the evaluation of rib fractures should take as less time as possible in acute trauma management. Post-processing software for CT data provides an automated recognition and numbering of all ribs, which are displayed – unfolded and rotatable – in one single plane (Fig. 17). ‘The unfolded rib display significantly reduces reading time for detection of rib fractures in acute thoracic trauma and does not significantly compromise the diagnostic accuracy’ (direct citation Bier et al. 2015). Moreover, the diagnostic accuracy of inexperienced readers (approximately 80 % for multiplanar reformatted images vs. approximately 92 % for unfolded rib images) may even be increased (Bier et al. 2015). Corresponding results were published for the detection of multiple myeloma and metastases from lung cancer (Homann et al. 2015a, b). Bier et al. documented a possible reduction of reading time by approximately 67–80 % (Bier et al. 2015) and a significant enhancement of inter-reader agreement. Manual correction is necessary in cases of incomplete or inaccurate recognition of ribs (Ringl et al. 2015). Furthermore, the utilisation of this technology involves the risk of false-positive findings because of two reasons: first, motion artefacts are harder to identify due to the missing correlation with surrounding tissue and, second, artificial curves caused by the software can be mistaken for buckled fractures (Bier et al. 2015; Homann et al. 2015a). However, these may be easily recognised after completing a short training (Ringl et al. 2015). If included in the standard calculation process of CT imaging, the unfolded rib display may be noticeably useful in the evaluation of the acute trauma patient when saving time is crucial.
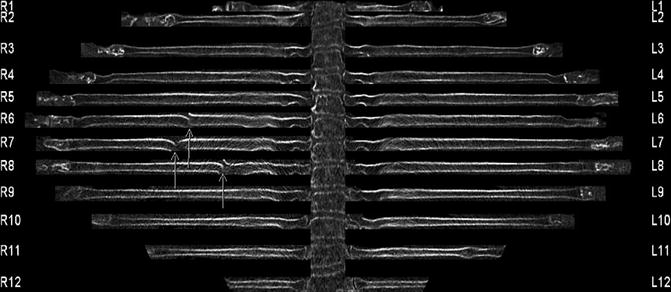
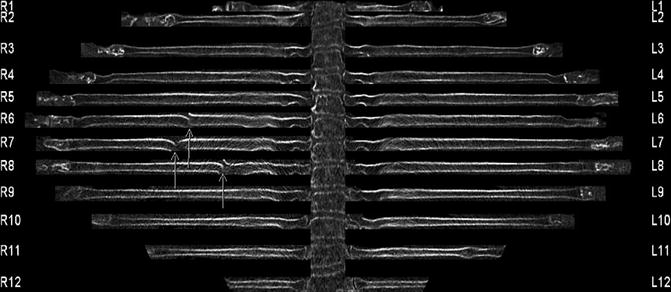
Fig. 17
Unfolded rib display (R1-12 = right ribs, L1-12 = left ribs) of an 88-year-old male patient with traumatic fractures of the sixth, seventh and eighth right rib (arrows)
Intercostal arteries are regularly exposed between the ribs within the first 6 cm lateral to the spinous process. From this point on, 97 % of intercostal arteries proceed in close local relation to the lower margin of the superior ribs. These ribs serve as shields for intercostal arteries due to their proximity but constitute a threat in case of a fracture (Helm et al. 2013). Rib fractures may tear intercostal, subclavian, axillary and internal mammary arteries and may cause life-threatening intrathoracic bleeding (Fig. 18) with a possible compression of heart and lungs. As a consequence of the increment of the blood density during the clotting process, active haemorrhage usually presents in CT as layers of different attenuation (Mirvis et al. 2014, p. 178). An extravasation of contrast material (attenuation value close to that of an adjacent artery) in CT is evidence of active bleeding (Fig. 19) (Kaewlai et al. 2008, pp. 1561–1565). Treatment can be endovascular (balloon occlusion/covered stent) or open repair. Shalhub et al. found endovascular therapy in case of blunt traumatic injuries to the thoracic outlet arteries to be superior to open repair in terms of operation time (149 min vs. 230 min, p = 0.03), blood loss (50 ml vs. 1.225 ml, p = 0.03) and morbidity (not statistically significant) (Shalhub et al. 2011).
