div class=”ChapterContextInformation”>
10. Imaging Approaches for Aortic Disease
Keywords
Thoracic aortaAbdominal aortaMagnetic resonance angiography (MRA)Computed tomography (CT)Positron emission tomography (PET)Ultrasound (US)Acute aortic syndrome (AAS)AneurysmVasculitisIntroduction
Aortic diseases are a significant cause of morbidity and mortality that can potentially result in catastrophic clinical consequences if a diagnosis is delayed. Therefore, prompt and accurate assessment is paramount to safe patient care and appropriate clinical outcomes. Aortic diseases can broadly be divided into valvular, aneurysmal, dissection-related, atherosclerotic, trauma-related, and congenital. The goal of this chapter is to outline common disease processes that affect the aorta, with emphasis on the strengths and limitations of the different imaging modalities, which are currently in use.
Overview of Imaging Techniques
The imaging options available for assessment of the thoracic aorta include plain radiography, transthoracic echocardiography (TTE), transesophageal echocardiography (TEE), computed tomography (CT), computed tomography angiography (CTA), magnetic resonance imaging (MRI), and aortography. Imaging modalities used for the abdominal aorta include ultrasonography (US), CT, CTA, MRI, and catheter angiography.
Plain Radiography
Plain radiography has limited use for assessing the thoracic aorta and is mostly utilized as an initial screening tool. Signs such as an abnormal aortic contour or widening of the aortic silhouette in a suggestive clinical scenario raise the clinical suspicion for aortic dissection or other aortic pathologies. CXRs have a sensitivity of 64% and a specificity of 86% for acute aortic syndromes [1]. However, radiographs alone are not sensitive enough to exclude aortic pathology or blunt aortic injury when it is clinically suspected [2, 3].
Echocardiography
Transthoracic echocardiography is a noninvasive imaging technique that is widely used in clinical practice due to its rapid availability and portability. It is highly effective in the evaluation of the proximal aorta, the aortic valve, left ventricular morphology and function, and suspected pericardial effusions. However, due to limited acoustic windows and reduced anatomic coverage, it is not considered the first-line imaging technique for the entire aorta and often demands further evaluation with TEE, CT/CTA, and MRI. Compared to TTE, TEE is a more sensitive and specific modality, offering superior visualization of the posterior cardiac structures and is particularly effective at assessing diseases of the aortic root. TEE has high sensitivity in the evaluation of suspected acute aortic pathology (dissection, transection, and intramural hematoma), suspected prosthetic valve dysfunction (thrombus, pannus ingrowth, vegetation, or regurgitation), and screening for left atrial thrombus in patients with atrial fibrillation or atrial flutter [4–6]. However, since TEE provides limited anatomic coverage of the aortic arch and descending thoracic aorta, it is mainly reserved for evaluating diseases at the aortic root, such as aneurysms and dissections. Since TEE is a portable modality, it is particularly useful for evaluating sick patients in the intensive care unit or emergency department, who cannot be transferred for more advanced imaging with CT or MR. TEE is also an invasive procedure with possible morbidity [7], albeit small, and is therefore mostly used for exclusion of aortic root pathology.
Ultrasonography
Ultrasound is the examination of choice for initial workup of abdominal aortic pathologies. It has a sensitivity of nearly 100% in the diagnosis of abdominal aortic aneurysms (AAA) [8]. Ultrasonography is largely preferred for screening and monitoring because of its relatively low cost, widespread availability, and noninvasive nature. It is accurate to within 0.3 cm in estimating aneurysm diameter on serial scans with little interobserver variation [9]. However, limitations include suboptimal image quality in patients who are obese or who have excessive bowel gas. Furthermore, US cannot reliably identify the presence of periaortic disease or the proximal and distal extent of an aneurysm. It also has limited ability in determining the patency of the visceral vasculature, the relationship of an aneurysm with respect to renal vessels, or the presence of iliac aneurysms. As a result, US alone cannot fully provide the necessary information required in the preoperative evaluation of a patient undergoing elective aneurysm repair.
Contrast-enhanced ultrasound (CEUS) is an evolving complementary imaging technique useful for real-time characterization of macrovascular pathologies like aortic dissection, ruptures, and endoleaks following endovascular repair of AAA , as well as microvascular pathologies like aortitis. This technique uses microbubbles containing inert gases as ultrasonographic contrast agents, making them safe for patients with impaired renal function [10, 11].
Computed Tomography and Computed Tomography Angiography
The speed and ease of CT acquisitions render this technique of choice for diagnosing acute and chronic aortic pathologies, such as intramural hematomas, aneurysms, traumatic injuries, atherosclerosis, and dissections. Multi-detector row CT (MDCT) has become one of the most widely used imaging modalities for the assessment of aortic diseases. Its major advantages are excellent spatial and temporal resolution, widespread availability, and ability to image the entire aorta (i.e., thoracic and abdominal aorta) within seconds. By markedly shortening the scan time, respiratory motion artifacts are limited, and the dose of iodinated contrast can be reduced [12]. It also enables easy visualization of the aortic lumen and wall, resulting in precise and reproducible measurements of aortic diameter. Current scanners with higher rows of detectors allow acquisition of isotropic volumetric datasets, which can be reconstructed in any plane for optimal display and measurement [13].
ECG-gated thoracic CTA protocol
Pre-contrast (Y/N) | Yes | |
---|---|---|
CTA (contrast) | mAs | 120 care dose |
IV contrast (injection rate) | 70 cc (4 cc/s) | |
Slice thickness | 2 mm | |
Collimator | 192 × 0.6 | |
Rotation time | 0.25 | |
Pitch | 3.2 | |
kV | 80–120 kV (90 ref. care kV) | |
Delayed post-contrast | Indicated for assessment of vasculitis |
Newer approaches to CT imaging of the aorta include dual-energy CT (DECT) that uses both low-energy (i.e., 80–100 kVp tube potential) and high-energy (140 kVp) photons when acquiring data – this is becoming more widely available for aortic imaging. It can be used for quantifying aortic calcification, thus enabling identification of patients at risk for adverse cardiovascular events [15]. Alternatively, it can be employed to subtract calcification from the aortic wall to allow improved visualization of the mural contours, thereby facilitating more accurate measurements of aortic dimensions. Spectral detector CT (SDCT) is also a new technique in which multiple spectrally distinct attenuation datasets are acquired, enabling differentiation of tissues with varying attenuation at different photon energies. SDCT aids in artifact reduction, increasing intravascular contrast in suboptimally enhanced contrast studies and differentiating delayed slow flow from the thrombus [16]. Single-source dual-layer detector CT which involves the use of a single-source scanner that discriminates DECT data at the detector level and photon-counting detector CT that uses a polychromatic x-ray energy beam to acquire DECT data are other novel CT techniques that hold promise.
Various post-processing image visualization techniques can also be used for CTA including multiplanar reconstruction (MPR), maximum intensity projection (MIP), 3D volume rendering (3D-VR), and curved planar reformation (CPR).
The main disadvantages of CTA are the need for contrast administration and ionizing radiation exposure. Iodinated contrast agents (ICA) may cause allergic reactions and need cautious use in patients with nephropathy. The administration of ICA may cause contrast-induced nephropathy (CIN), especially in those with pre-existing renal impairment. The incidence of CIN in the general population is up to 6%; however, studies indicate that up to 50% of patients with pre-existing nephropathy are at risk of developing CIN [17]. Ionizing radiation exposure from CT may limit its use, especially in young patients who may require serial follow-up imaging [18]. Dramatic decreases in radiation exposure have been observed recently from using various dose reduction techniques, including prospective ECG triggering, ECG-based tube current modulation, lower peak kilovoltage (kVp), and iterative reconstruction algorithms [19, 20].
Compared to other modalities such as TTE and MRI, CT lacks flow assessment capabilities, which are useful in assessing aortic insufficiency and shunts. Cardiac motion artifacts mainly affect the aortic root in non-ECG-gated CTA and are more frequently present with heart rates of 65 bpm or more. ECG gating reduces motion artifacts from cardiac and aortic movement at the cost of an increase in radiation dose; however this technique is still preferentially used for imaging the thoracic aorta [21], whereas non-ECG-gated CTA is preferred for imaging the abdominal aorta [22]. Retrospective ECG gating is rarely used in the thoracic aorta due to greater radiation exposure [23] but may be used in clinical situations where there is concomitant aortic valve disease (e.g., infective endocarditis).
Magnetic Resonance Imaging
Clinical indications for different MRA sequences
Acute aortic syndromes | Thoracic aortic aneurysm | Congenital abnormalities | Vasculitis | |
---|---|---|---|---|
bSSFP | + | + | + | + |
Cine bSSFP | + (AV valve) | + (AV valve) | ||
TR-MRA | + | + | + | + |
CE-MRA | + | + | + | + |
PC-MRA | + (AV valve) | + (AV valve) | ||
Pre-contrast VIBE | + | + | ||
Post-contrast VIBE | + | + (delayed post-contrast for inflammation) | ||
NC-MRA | + | + | + | + |
MRI Techniques
Balanced Steady-State Free Precession
Balanced steady-state free precession (bSSFP) techniques are gradient echo techniques that are widely used for cine imaging of the heart. These sequences are primarily T2 weighted and produce a high signal from blood without the need for a contrast agent. The contrast-to-noise ratio depends on the T2/T1 relaxation differences of the tissues, which at short repetition times (TR) is high for blood and soft tissues, thus improving the overall image quality. There are different strategies for implementing the bSSFP technique: as a single shot (electrocardiographically (ECG)-triggered 2D acquisition), as cine (breath-hold ECG-triggered segmented k-space acquisition), as real-time cine (which does not require breath-holding or ECG triggering but with lower spatial and temporal resolution), or as a 3D sequence (nonselective radiofrequency pulse with segmented acquisition to obtain isotropic 3D data with very high spatial resolution) for imaging the coronary arteries and thoracic aorta.
Black-Blood Imaging
Black-blood imaging (BB) enables evaluation of blood vessel walls and is useful for the evaluation of a variety of pathologies, including acute aortic syndromes, atherosclerosis, vasculitis, and neoplasms. A flow-sensitive double inversion recovery (DIR) technique is used to null signal from flowing blood [24]. This technique uses two 180° inversion recovery pulses in close succession to null the signal of flowing blood, helping to visualize the walls of the blood vessels. The first 180° inversion recovery pulse is spatially nonselective which inverts the magnetization in the entire tissue volume, including the blood signal, while the succeeding reversion 180° pulse is spatially selective and regenerates the magnetization for imaging [25]. Vectorcardiogram (VCG)-gated proton density-weighted 2D fast spin-echo (FSE) sequence is the most commonly used pulse sequence. VCG gating increases imaging time; however, it significantly reduces motion artifact. Single-shot FSE (SSFSE) sequences are much faster and allow for image acquisition in either a single breath-hold or during free breathing. T1-weighted BB images with fat saturation are recommended in an acute setting, to help in the diagnosis of aortic wall hematomas [26]. T2-weighted BB imaging with fat saturation helps to evaluate edema in inflammatory disorders [27].
T1-Weighted Gradient Echo Fat-Saturated Imaging
T1-weighted gradient echo fat-saturated (GRE-FS) imaging before and after contrast injection is used routinely in evaluating several thoracic pathologies. Pre-contrast T1 GRE-FS is useful in demonstrating intramural hematomas, which appears as a bright T1-hyperintense region within the aortic wall. The entire thoracic aorta is imaged in axial and either coronal or sagittal orientations before and after contrast injection. Delayed post-contrast GRE-FS may be used to assess contrast enhancement of the aortic wall in conditions such as vasculitis.
Contrast-Enhanced MRA
Time-Resolved MRA (TR-MRA)
Advanced gradient strength with modern MRI systems allows shorter acquisition times per 3D acquisition volume, enabling contrast-enhanced MRA (CE-MRA) to be implemented with sub-second temporal resolution [28]. This is achieved using a combination of acceleration strategies, i.e., short TR, parallel imaging, and view sharing (e.g., TWIST). When these strategies are used in combination, the aorta can be imaged with acquisition speeds of 1–2 frames per second, allowing evaluation of high-flow vascular lesions, such as shunts and dissections. In its most basic implementation, TR-MRA can be used as a timing bolus acquisition for planning the higher-resolution CE-MRA.
Conventional CE-MRA
Conventional CE-MRA is the most widely used technique for a comprehensive evaluation of the thoracic aorta. It is a timed, single-phase acquisition, which provides better spatial resolution than TR-MRA, particularly in the z-direction, and produces better depiction of more subtle abnormalities, such as penetrating aortic ulcers. The basic pulse sequence for conventional CE-MRA is also a standard 3D GRE acquisition. Gadolinium contrast is injected through a cannula placed in an antecubital vein. The contrast transit time is calculated from the sub-second TR-MRA, which is used for bolus timing. Images are acquired during breath-holding. Image subtraction is employed to enhance contrast, and subtracted 3D sets are calculated from the raw data which are subjected to MIP and volume-rendering post-processing algorithms. ECG gating is used for CE-MRA of the thoracic aorta to provide artifact-free images of the aortic root in particular, similar to CTA [29, 30].
Phase-Contrast MRA (PC-MRA)
Phase-contrast MRA (PC-MRA) is a velocity-sensitive imaging technique that is most useful where there is high suspicion for vascular stenoses, such as in coarctation. This technique depends on velocity differences or phase shifts in moving blood (i.e., protons) to produce image contrast in flowing blood. Phase shifts from moving spins are generated by applying magnetic field gradients with alternating opposing polarities. The velocity-encoding (VENC) variable controls the amplitude of the magnetic gradient and is adjusted up or down depending on the amplitude of the velocity being measured. PC-MRA can be implemented as a 2D or 3D acquisition. 2D PC-MRA is useful when the region to be assessed is clearly visible so that the imaging slice can be accurately placed. 4D flow MRI combines tri-directional VENC with a 3D spatial acquisition potentially allowing vascular flow patterns to be visualized and measured from a single 3D acquisition [31]. This technique allows assessment of complex vascular hemodynamics with MRI in large aneurysms or congenital heart diseases. Disadvantages of this technique include long acquisition times and complex time-consuming post-processing. This technique is described in greater detail in a subsequent chapter.
Non-contrast MRA
Non-contrast MRA (NC-MRA) techniques are increasingly being used routinely, particularly in “at risk” patients, such as those with renal failure or pediatric patients. NC-MRA of the thoracic aorta incorporates several techniques including bSSFP which produces bright blood signal determined by T2/T1 ratio and FSE which relies on an ECG-gated 3D partial-Fourier FSE sequence, which is triggered to systole and diastole [32]. bSSFP can be implemented as a 2D or 3D acquisition. 2D SSFP is a rapid free-breathing technique that is useful in acutely ill patients and reserved for excluding serious pathology such as aortic dissection [33]. 3D SSFP has a longer acquisition time (typically 3–4 minutes with respiratory gating) and facilitates accurate orthogonal measurement of aortic aneurysms. FSE approaches depend on the timing of acquisition to different parts of the cardiac cycle adding complexity and consequent error to the imaging protocol.
Positron Emission Tomography-Computed Tomography (PET-CT) or Nuclear Imaging
Although PET-CT has been established as a standard imaging modality in the evaluation of malignancy, growing evidence suggests that PET-CT images have many advantages in the evaluation of aortic disease as well, especially acute aortic syndromes, aortic aneurysms, atherosclerotic lesions, aortitis, and aortic tumors [34]. Elevated uptake of fluorine-2-deoxy-D-glucose (FDG) has been correlated with the development and progression of both thoracic and abdominal aneurysms in a number of clinical studies [35–39]. Other radiotracers targeting matrix metalloproteinases, mitochondrial translocator proteins, and endothelial cell adhesion molecules are being investigated for clinical utility in identifying the progression of disease in aortic aneurysms [40]. FDG PET/CT imaging of atherosclerosis allows for the in vivo visualization of vascular inflammation, which can help evaluate plaque vulnerability and predict clinical events. It is also useful in diagnosis, differential diagnosis, and the identification of target sites for biopsy in aortitis. Moreover, it is helpful for evaluating disease extent and activity, predicting prognostic outcomes, monitoring response to therapy, and evaluating therapeutic effectiveness [34].
Abnormalities of the Thoracic Aorta
Acute Aortic Syndromes
Acute aortic syndrome (AAS) comprises of three overlapping clinical entities: aortic dissection, intramural hematoma, and penetrating atherosclerotic ulcer [41].
Aortic Dissection
Aortic dissection is the most common type of AAS [42, 43]. It is a potentially life-threatening condition that occurs secondary to an aortic intimal tear which allows blood to propagate into the media and separate the layers of the aorta, forming a false lumen which is separated from the true lumen by an intimal flap [44]. Although the true incidence of aortic dissection is difficult to define, a recent population study has suggested that the incidence of aortic dissection is up to 4.6 cases per 100,000 people per year [45]. The most important risk factor for the development of aortic dissection is uncontrolled hypertension. Other risk factors include increased age, cocaine abuse, bicuspid aortic valve, connective tissue disorders, and aortic surgery or infection.
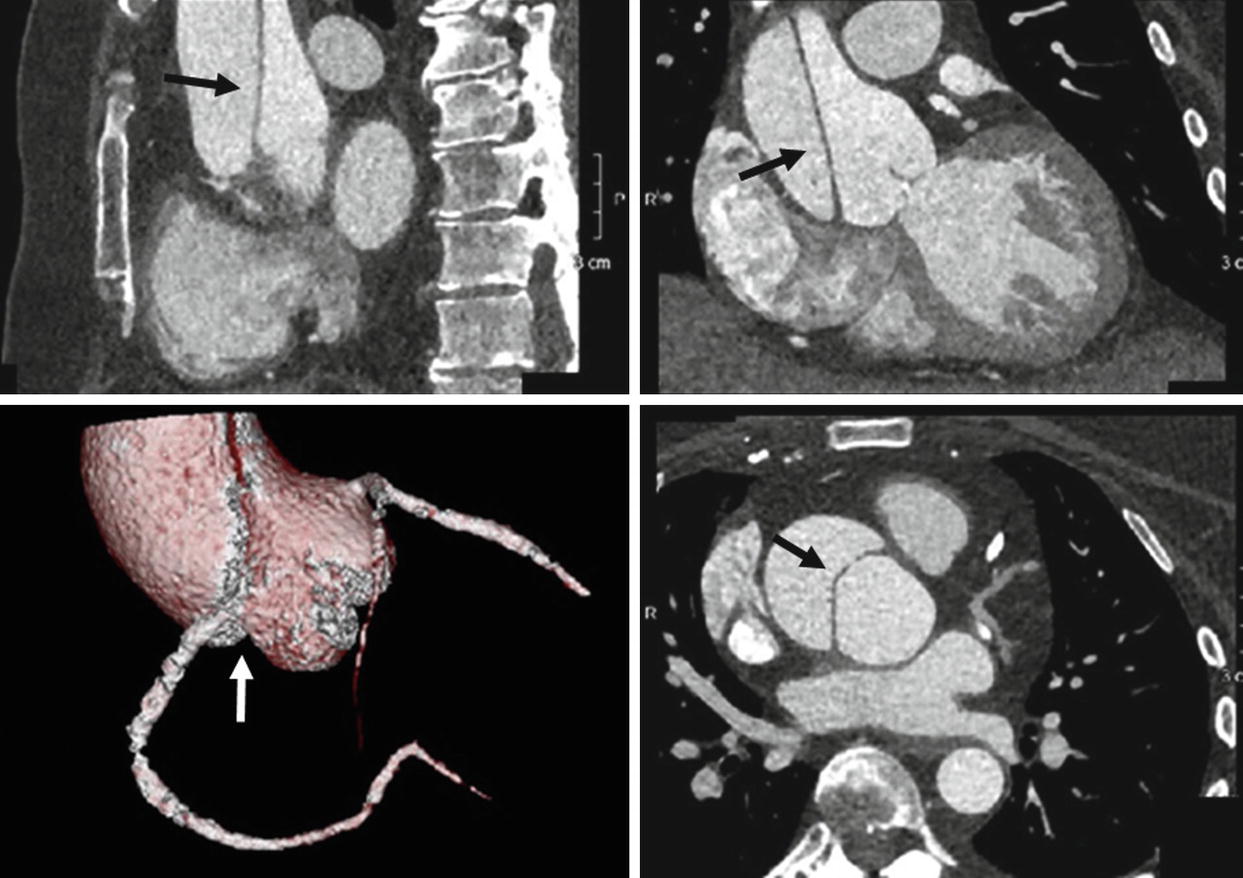
A 61-year-old female presenting with chest pain. ECG-gated CTA of the thoracic aorta depicting a Type A aortic dissection (black arrows) which extends from the root to the ascending aorta. Note the extension of the dissection flap into the ostium of the right coronary artery depicted on the volume-rendering image (white arrow)
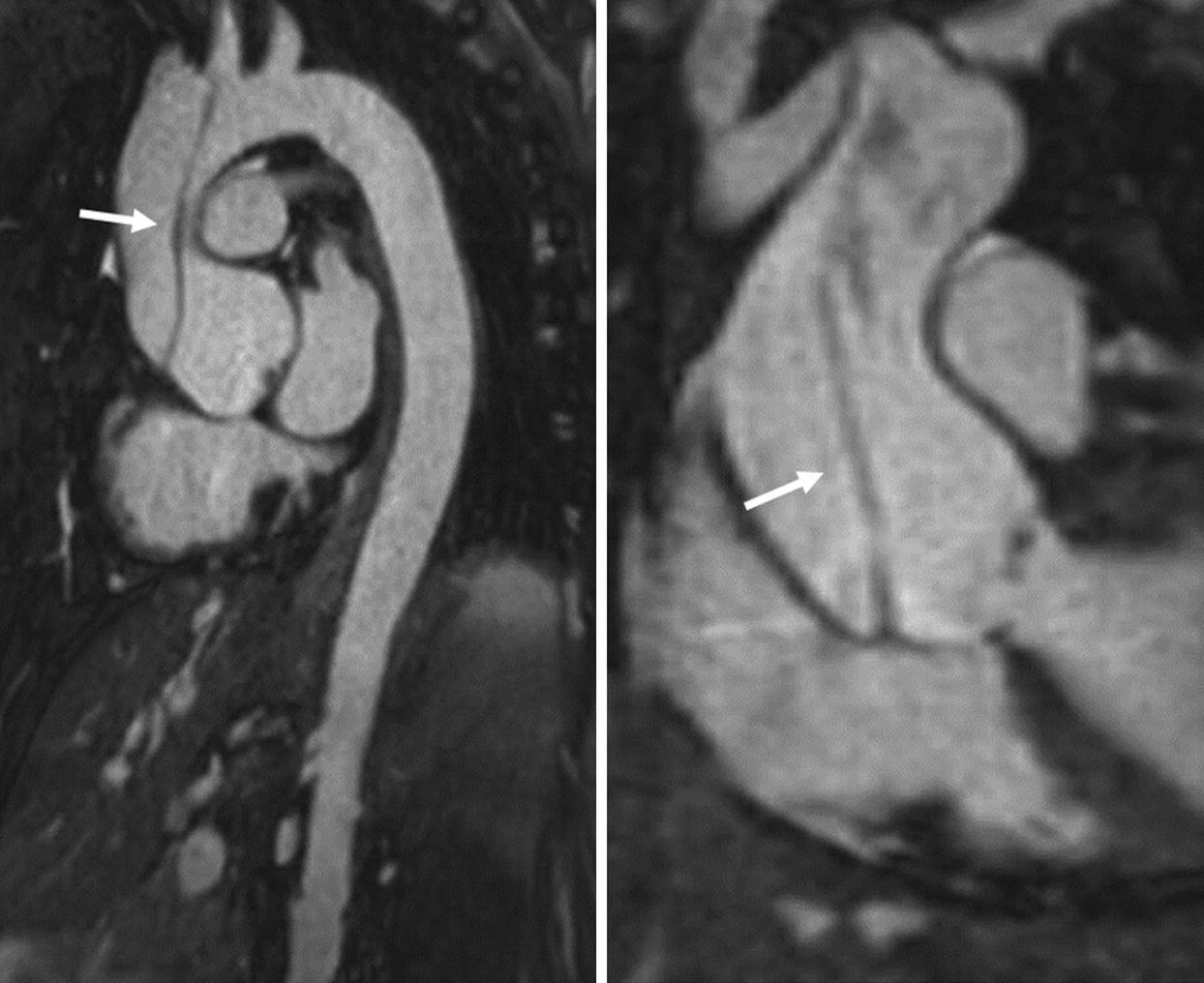
This is the same patient from above. The sagittal and coronal contrast-enhanced MRA of the aorta demonstrates a Type A dissection with the flap extending from the root into the mid-aortic arch (arrows)
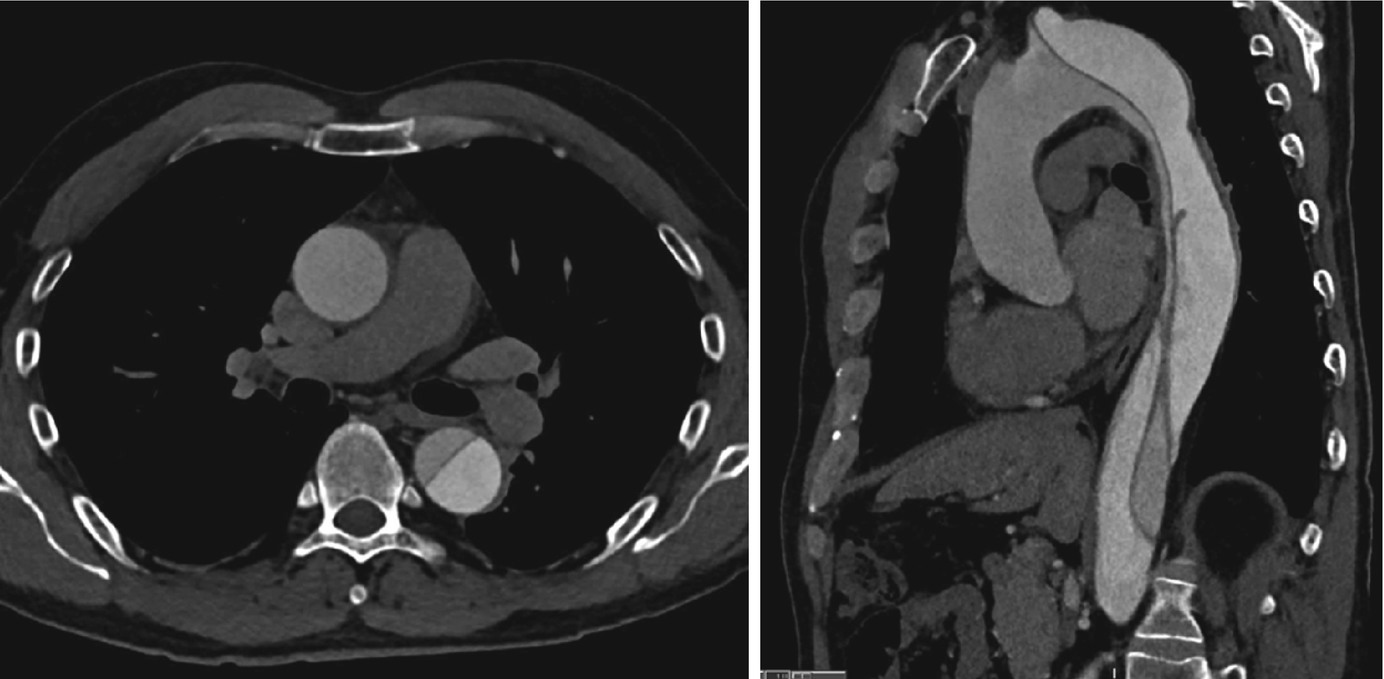
A 59-year-old man with history of Type B aortic dissection, conservatively managed to date. Axial and sagittal reformats of an ECG-gated thoracic CTA demonstrating a Type B aortic dissection arising from the lesser curvature extending to the origin of the left subclavian artery and extending to the common iliac arteries bilaterally (not shown)
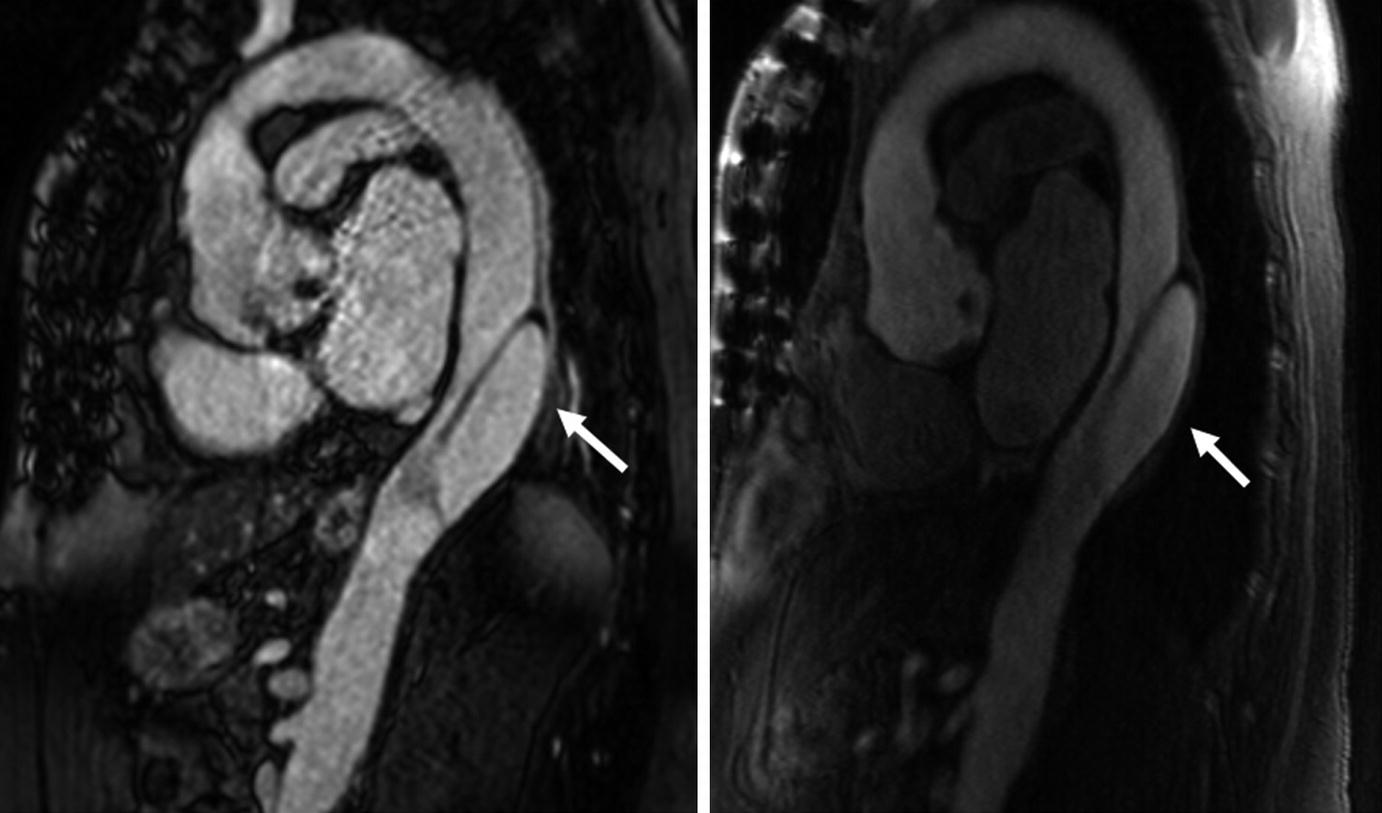
A 61-year-old female with a history of chronic type dissection following a motor vehicle accident 2 years prior. Sagittal bSSFP MRA (a) and CE-MRA (b) of the thoracic aorta demonstrating a Type B aortic dissection involving the mid-descending thoracic aorta and extending to the bilateral common iliac arteries (not shown). The false lumen is enlarged and partially thrombosed proximally
The American College of Radiology (ACR) imaging recommendations for aortic dissection
Imaging modality | Comments |
---|---|
CXR | Done mainly to rule out other pathology. Non-specific findings, low negative predictive value |
CTA chest and abdomen with contrast | Rapid, cost-effective, safe, and minimally invasive modality. Information provided on the intimal flap, entry/reentry site, branch vessel involvement, and other diagnoses. Considered the gold standard at many centers |
Angiography | Invasive, high contrast, and radiation burden. Used rarely when the branch vessel involvement is not determined from CTA. Still required in the definitive preoperative evaluation of coronaries if other examinations were suboptimal |
MRA chest and abdomen with or without contrast | Used in hemodynamically stable patients, those with contraindications to contrast, those with uncertain diagnosis or suspicion for a chronic dissection. Newer imaging sequences may allow quicker imaging. Information provided by non-contrast ECG- and respiratory-gated MRA is comparable to contrast-enhanced MRA, making it an appealing alternative in those with nephropathy |
Echocardiography | Bedside, rapid, widely available. TEE is recommended if skilled operator and reader are present and if the hemodynamic stability of patient precludes CTA or MRA. Sensitivity is similar to that of MRA and CTA. Can assess coexisting coronary artery involvement. TTE not recommended due to low sensitivity in detecting distal dissections |
FDG-PET/CT skull base to mid-thigh | May be recommended in distinguishing acute from chronic dissection in certain clinical scenarios, if available |
CTA is considered the first-line test for assessing aortic dissection as it provides an accurate assessment of the entire aorta in the chest and abdomen within seconds and at high spatial resolution. CTA can accurately distinguish the true from the false lumen and assesses the extent of the dissection and involvement of aortic branches. A pre-contrast scan of the upper thorax is considered an essential part of the clinical scan protocol in order to exclude intramural hematoma, which may be a precursor for dissection. Additionally, a delayed venous-phase CT acquisition (40–60 seconds post-contrast injection) is usually included to allow visualization of a slow filling false lumen in aortic dissection.
MRA is usually reserved for patients with a contraindication to CT. MRA has high sensitivity and specificity for detection of dissection and is used as a first-line test or alternative to CTA [28]. The MRA protocol for dissection includes bSSFP, pre- and post-contrast GRE-FS, TR-MRA, CE-MRA, and delayed-phase post-contrast GRE-FS. bSSFP has been shown to be highly accurate for diagnosis and classification of aortic dissection in sick patients who cannot hold their breath [33]. Breath-hold cine SSFP or non-ECG-triggered real-time SSFP can be used to evaluate aortic insufficiency and hemopericardium in Type A dissections. In chronic aortic dissections which become aneurysmally dilated, 4D flow MRI may be very useful for identifying the site of fenestration between true and false lumen [49].
Intramural Hematoma (IMH)
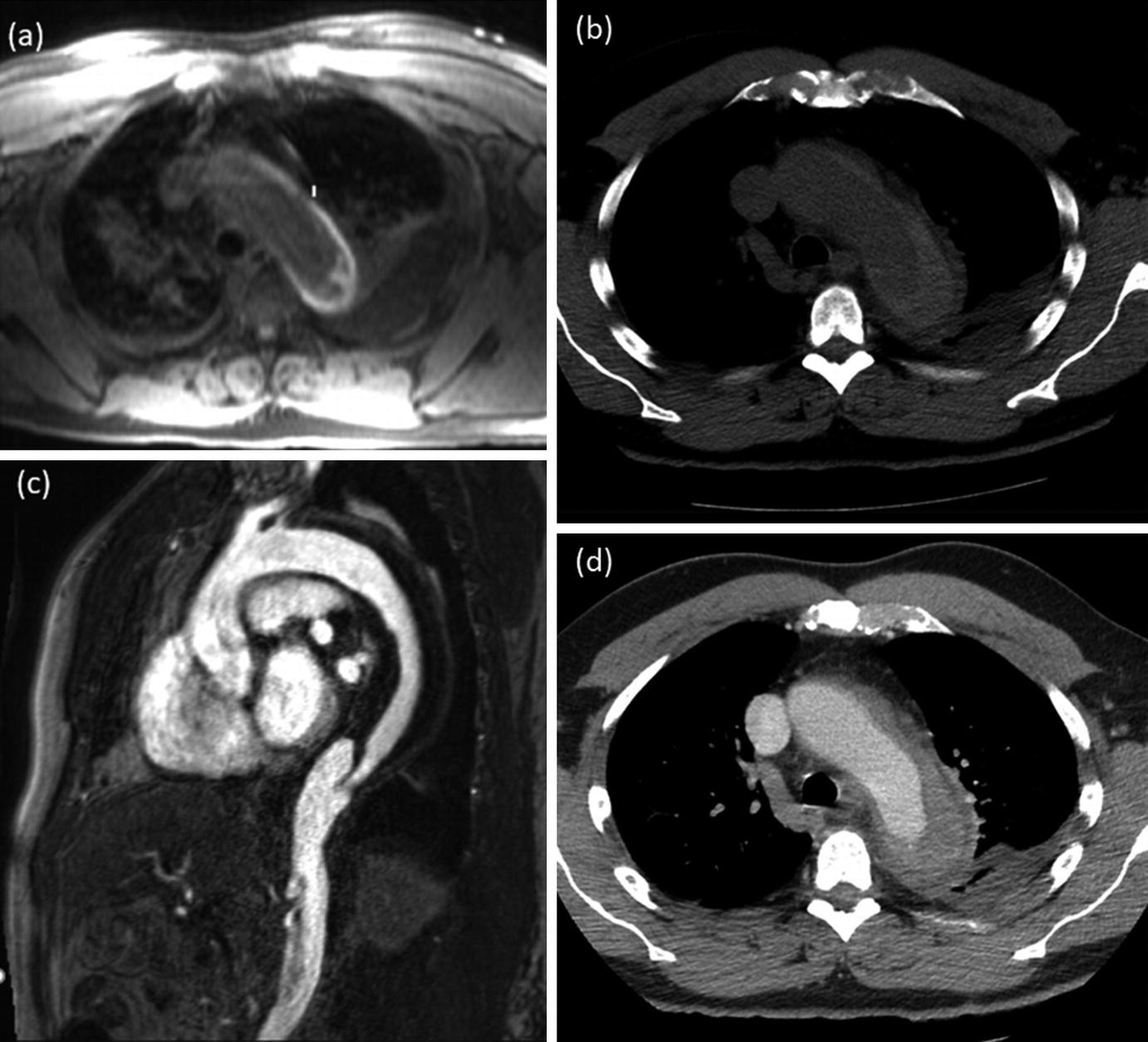
MRA and CTA images of a 59-year-old male patient with intramural hematoma. Axial T1 VIBE image (a) demonstrates a crescent-shaped hyperintensity in the distal aortic arch with corresponding hyperdensity on the non-contrast CT image (b). The intramural hematoma demonstrates hypodensity on the sagittal contrast-enhanced MRA (c) and the axial post-contrast CT scan (d)
Penetrating Atherosclerotic Ulcer
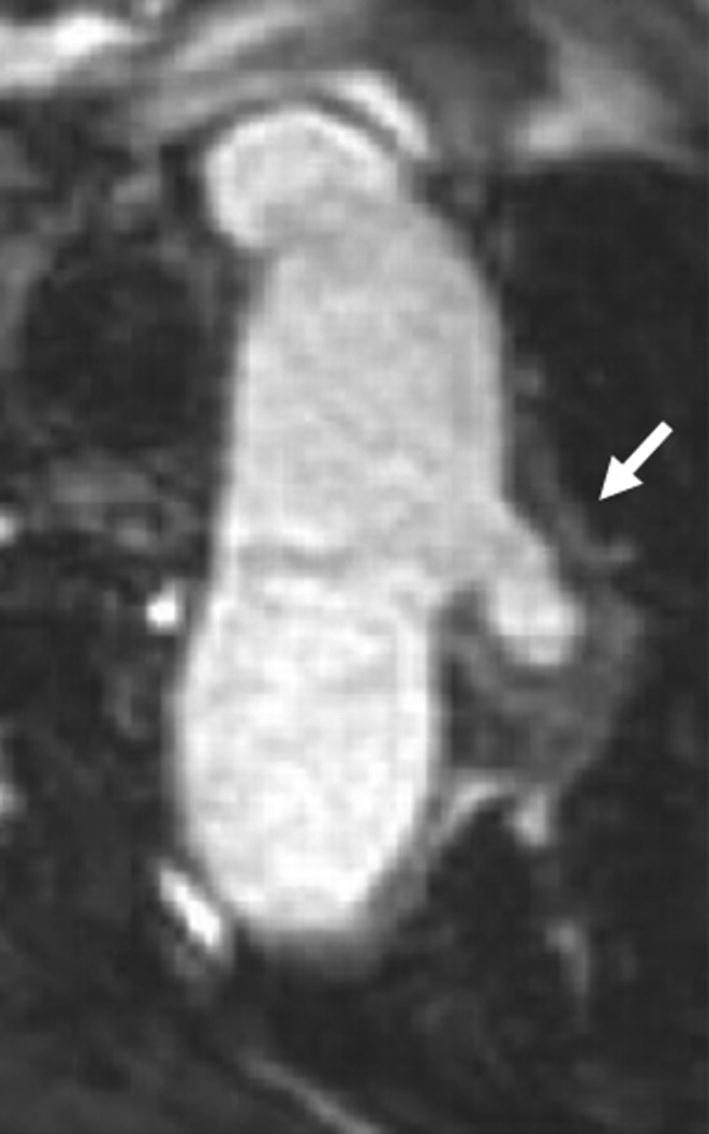
Non-contrast MRA (navigator balanced SSFP) in a 55-year-old male patient referred for arch abnormality identified on transesophageal echocardiogram; axial slice demonstrates the outpouching from the lateral aspect of the arch with partial thrombus consistent with a penetrating atherosclerotic ulcer (arrow)
Thoracic Aortic Aneurysm
Thoracic aortic aneurysms (TAA) are a relatively common and important pathologic entity which predispose to lethal clinical consequences such as rupture or dissection if left undiagnosed [52]. The incidence of TAA is estimated to be up to 7.6 cases per 100,000 person-years [45]. Many risk factors have been implicated in the development of TAA, the most common being chronic atherosclerosis, hypertension, smoking, and hyperlipidemia [53–55]. TAAs are clinically silent and most commonly discovered as an incidental finding when conducting thoracic imaging for other unrelated conditions. When present, symptoms may be subtle and include stridor or hoarseness due to the compressive effect of an enlarging aneurysm or dyspnea and fatigue secondary to aortic root involvement and resulting aortic regurgitation causing heart failure.
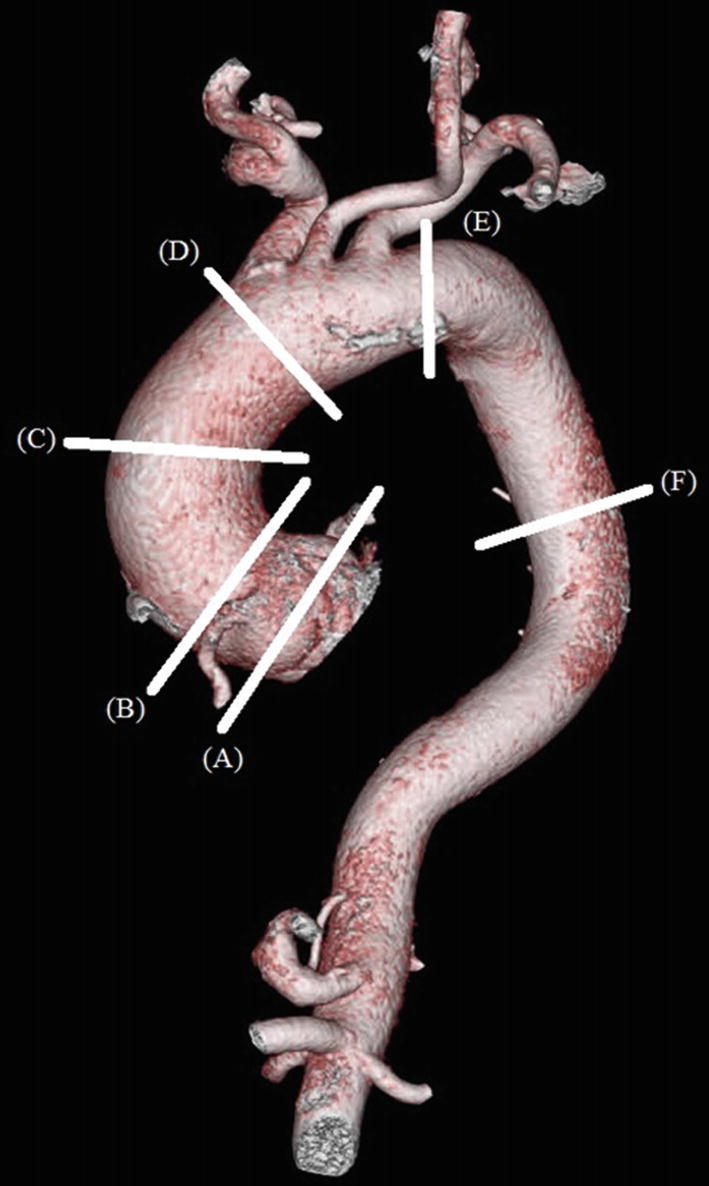
Volume-rendered CTA of a thoracic aortic aneurysm. (A) Sinus of Valsalva: 47 × 44 × 41 mm. (B) Sino-tubular junction: 43 × 41 mm. (C) Mid-ascending aorta: 49 × 47 mm. (D) Proximal aortic arch: 41 × 40 mm. (E) Distal aortic arch: 31 × 29 mm. (F) Lower descending thoracic aorta: 28 × 27 mm
Guidelines for thoracic aortic aneurysm surveillance and recommended management
Aneurysmal description | Size and frequency of monitoring |
---|---|
Aortic root/ascending aortic aneurysm (sporadic/degenerative) | 3.5–4.5 cm: annual CTA or MRA 4.5–5.4 cm: biannual CTA or MRA Over 5.4 cm: surgical intervention -If aortic valvular disease present concomitant TTE is recommended |
Aortic root/ascending aortic aneurysm (genetically mediated/connective tissue disease) | 3.5–4.0 cm: annual CTA or MRA 4.0–5.0 cm: biannual CTA or MRA Over 5.0 cm: surgical intervention -If aortic valvular disease present concomitant TTE is recommended |
Descending aortic aneurysm | 4.0–5.0 cm: annual CTA or MRA 5.0–6.0 cm: annual CT or MRA Over 6.0 cm: surgical intervention |
The American College of Radiology recommendations for imaging thoracic aortic aneurysms
Imaging modality | Comments |
---|---|
CXR | Helpful to exclude other thoracic pathology, but findings such as a widened mediastinum or tortuous aorta on CXR are not sensitive or specific to TAA |
CTA chest with contrast | Provides an excellent anatomic assessment including branch involvement and pre- and postoperative assessment. ECG-gated CTA has provided the ability to minimize cardiac motion artifacts, particularly at the aortic root. More appropriate than MRA if the patient is hemodynamically unstable. Limited in follow-up by high radiation and contrast burden |
MRA chest with or without contrast | Allows for excellent assessment and follow-up of TAA size, shape, extent, and diameters with no radiation exposure. Although ECG- and navigator-gated CE-MRA is currently the gold standard to evaluate TAA, an NC-MRA using bSSFP is an acceptable alternative to CE-MRA in those with nephropathy. More appropriate than CT for aneurysms involving the aortic sinus and in patients who required follow-up for known TAAs to limit radiation exposure |
Echocardiography | Rapid, widely available modality which is particularly useful in unstable patients, may be useful for initial workup for TAA. Transthoracic echocardiography allows for adequate assessment of the aortic root; however, it provide limited assessment of the ascending and descending aorta. It also may be limited in obese patients or patients with an abnormal thoracic wall secondary to surgery or comorbid chronic obstructive pulmonary disease. Therefore, should be followed up with CTA or MRA. |
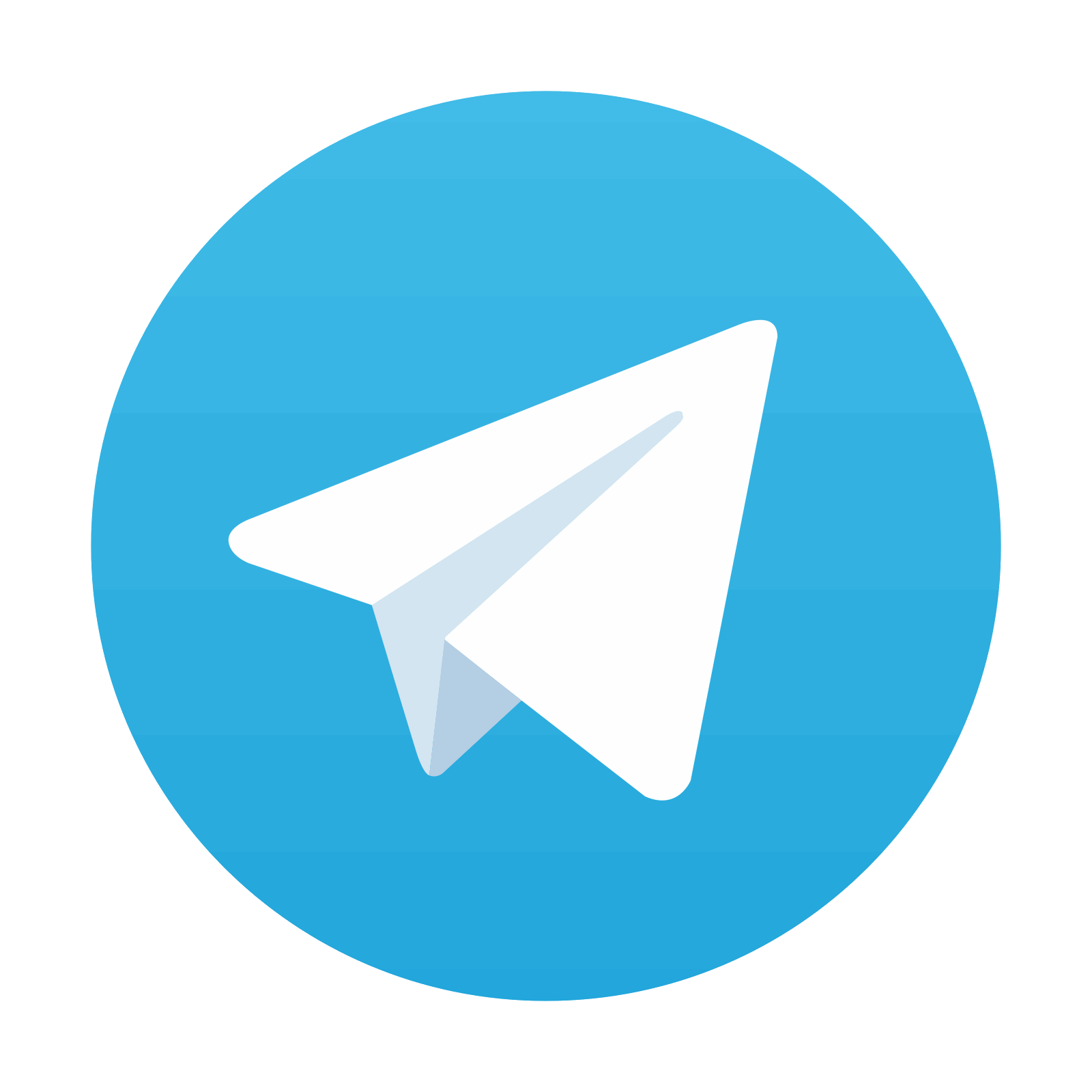
Stay updated, free articles. Join our Telegram channel

Full access? Get Clinical Tree
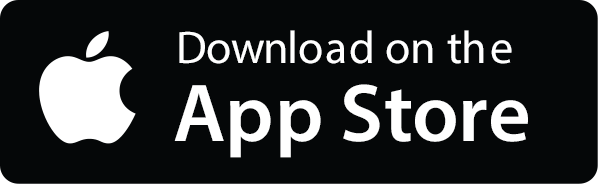
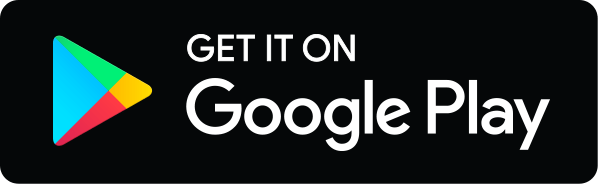