Natural sources
Man-made sources
External radiation
Medical
Cosmic rays
Occupational
Terrestrial radiation (radioactive material in rocks, such as potassium-40)
Nuclear power
Internal radiation
Nuclear explosions
Inhalation (radon gas)
Nuclear accidents
Ingestion
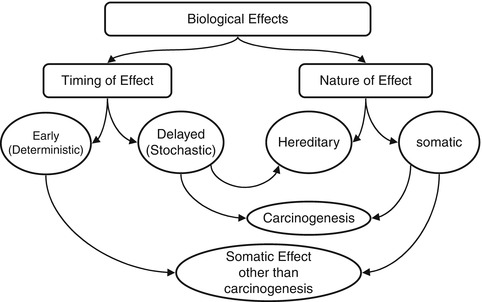
Fig. 21.1
The various biological effects of ionizing radiation. The effects can be classified into early or deterministic, which have a threshold, and delayed or stochastic, with no threshold. Effects are also classified into somatic and hereditary. The somatic include early and delayed effects (cancer)
Deterministic (non-stochastic) effects are nonrandom and have a highly predictable response to radiation. There is a threshold of radiation dose after which the response is dose related. Some of the known deterministic effects are radiation-induced lung fibrosis and cataract
21.2 Mechanisms of Radiation Effects
Ionizing radiation exerts its effects on biological targets through two major mechanisms [1, 2], direct and indirect (Fig. 21.2).
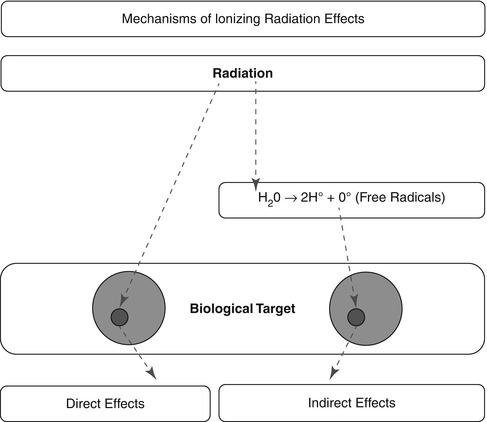
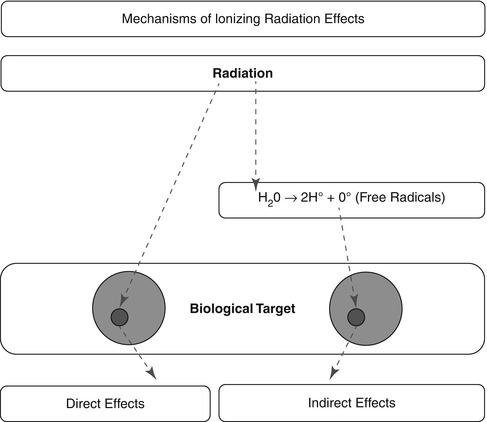
Fig. 21.2
The two mechanisms of ionizing radiation effects on biological tissue: the direct, or target, mechanism and the indirect, through the production of free radicals that consequently cause damage
21.2.1 Direct Effect
The direct effect theory or target theory proposes that ionizing radiation acts by direct hits on target atoms. All atoms or molecules within the cells, such as enzymatic and structural proteins and RNA, are vulnerable to radiation injury. DNA, however, is the principle target, in which ionizing radiation produces single- or double-stranded chromosomal breaks.
21.2.2 Indirect Effect
The direct mechanism theory was found to be inadequate in explaining cellular radiation injuries. The indirect theory proposes that ionizing radiation exerts its effect via radiolysis of cellular water, forming free radicals. These free radicals interact with atoms and molecules within the cells, particularly DNA, to produce chemical modifications and consequently harmful effects. When X-rays interact with water, two types of free radicals are formed:


The presence of an excess of oxygen during irradiation of cells allows the formation of additional free radicals:




It is worth noting that antioxidants block hydroxyperoxy free radical combination into the highly unstable hydrogen peroxide.
It has been estimated that about two thirds of biological damage caused by low linear energy transfer (LET) radiation is due to indirect action [3]. Biological damage by high LET is primarily by direct ionization action. Figure 21.3 illustrates how radiation leads to tissue damage.
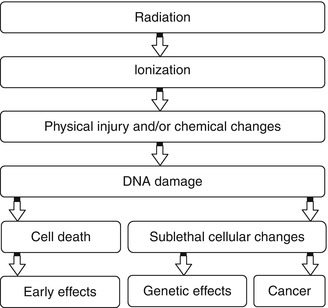
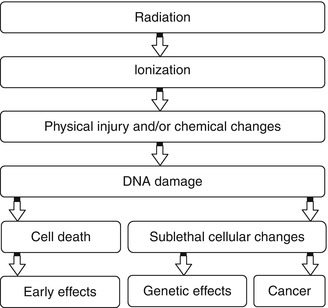
Fig. 21.3
Intracellular changes induced by ionizing radiation that lead to cell damage
Radiation effects have been observed in extents beyond that explained by effects exerted on directly irradiated cells. Cells in temporal or spatial distance from the initial radiation insult have been shown to have delayed effects of radiation. Two phenomenons are described: the bystander effect and genomic instability.
Bystander Effect
The cells in the vicinity of irradiated cells show effects that cannot be attributed to targeting by ionizing radiation tracks. Additionally, when cells are irradiated and later transferred to another medium, the cells in proximity in the new medium exhibit DNA damage, mutation, and carcinogenesis. Through cell-to-cell interaction, the directly irradiated cells communicate with adjacent cells and spread the effect of radiation to a larger number of cells. The mechanism is not clearly understood; however, gap junctional intercellular communication [4] or release of soluble factors (such as cytokines) [5] from irradiated cells is proposed. The bystander effect has been mainly described for densely ionizing radiation (such as alpha particles) [6], but also is seen in low LET radiation (such as gamma or X-rays).
Genomic Instability
Maximal radiation-induced genetic damage is formed shortly (minutes to hours) after radiation exposure. Nevertheless, it has been observed that not only the irradiated cells but also descendents may show delayed effects. Cells that sustain nonlethal DNA damage show increased mutation rate in descendent cells several generations after the initial exposure [7]. Delayed effects include delayed reproductive death up to six generations following the primary insult [8].
21.3 Factors Affecting Radiation Hazards
Radiation injury can be modified by factors related to the ionizing radiation and the target tissue.
21.3.1 Factors Related to Ionizing Radiation
Certain factors related to radiation itself determine the various effects for the same radiation dose to biological organs.
Type of Radiation. Various types of radiation differ in penetrability based on LET, which expresses energy loss per unit distance traveled (kiloelectron volts per micrometer). This value is high for alpha particles, lower for beta particles, and even less for gamma rays and X-rays. Thus, alpha particles penetrate a short distance but induce heavy damage, and beta particles travel a longer distance but much shorter than gamma rays.
Mode of Administration. The radiation dose is obviously an important factor. In addition, a single dose of radiation causes more damage than the same dose being divided (fractionated). Collectively these two factors are expressed as dose per fraction.
Dose Rate. Dose rate expresses the time for which dose is administered. The longer the duration for the same total dose, the better the chance of cellular repair and the smaller the damage.
21.3.2 Factors Related to Biological Target
Certain properties of tissues and cells can significantly modify the biological effects of ionizing radiation.
Radiosensitivity. Although all cells can be affected by ionizing radiation, normal cells and their tumors vary in their sensitivity to radiation. Slowly and rapidly growing cells have different radiosensitivity in relation to their movement through the cell cycle. Radiosensitivity varies with the rate of mitosis and cellular maturity. Blood-forming cells are very sensitive to radiation, while neurons, muscles, and parathyroid cells are highly radioresistant. Within a given cell, the nucleus in general is relatively more radiosensitive than the cytoplasm. When cells in G0\G1 phase of the cell cycle are exposed to radiation they tend to halt their progression into G2\M phase. G2 synchronization produces a cluster of radiosensitive cells. A second hit within a time frame of 5–12 h leads to higher proportion of deleterious effects. This is expected for radioisotopes with sequential alpha or beta decay as in 90Sr\90Y [9].
Repair Capacity of Cells. Some cells are known to have a higher capacity than others to repair the damage caused by ionizing radiation; consequently, the biological effects of the same radiation dose are different. Significant repair is known to occur quickly, within 3 h.
Cell-Cycle Phase. The life cycle of the cell includes several phases: the pre-DNA synthetic phase (G1), the DNA synthetic phase (S), the post-DNA synthetic phase (G2), mitosis (M), and the more recently identified phase of no growth (0), which represents the time after mitosis to the start of the G1 phase. All phases of the cell cycle can be affected by ionizing radiation. The radiosensitivity of a given cell varies from one cell-cycle phase to another. Overall, sensitivity appears to be greatest in G2 phase; irradiation during this phase retards the onset of cell division. Irradiation during mitosis induces chromosomal aberrations, i.e., breaks, deletions, translocations, and others. The sensitivity of a given cell-cycle phase also differs from one cell type to another and by alteration of radiation injury [3]. For example, the reproductive cells are most sensitive during the M phase, while damage to DNA synthesis and chromosomes occurs mostly when the cell is in the G2 phase. Recovery from sublethal damage occurs in all phases of the cell cycle. However, this is most pronounced in the S phase, which is also the most radioresistant phase [3].
Degree of Tissue Oxygenation. Molecular oxygen is known to have the ability to potentiate the response to radiation; this is known as the oxygen effect. The amount of molecular oxygen rather than the rate of oxygen utilization by the cells is the most important factor for increasing the sensitivity of cells to radiation. The probable mechanism is the allowance of additional free radicals, which enhance the damage of cells [10].
21.4 Radiation-Induced Cell Injury
In general, an injury which has a high chance of repair is sublethal, that which can be repaired with treatment is potentially lethal, and that which is permanent is lethal. The nucleus is relatively more radiosensitive than the cytoplasmic structures. Nuclear changes after radiation include swelling of the nuclear membrane and disruption of chromatin materials. Cytoplasmic changes include swelling, vacuolization, disintegration of mitochondria and endoplasmic reticulum, and reduction in the number of polysomes [2, 10]. Depending on the dose of radiation and the subcellular changes, along with the previously described factors, the potential effects on the cell vary (Table 21.2). After ionizing radiation exposure, cellular injury occurs in one of the following forms [11]:
Table 21.2
Types of cellular damage in relation to approximate radiation dose
Dose grays (rads) | Type of damage | Comments |
---|---|---|
0.01–0.05 (10–50) | Mutation (chromosomal aberration, gene damage) | Irreversible chromosome breaks, may repair |
1 (100) | Mitotic delay, impaired cell function | Reversible |
3 (300) | Permanent mitotic inhibition, impaired cell function, activation and deactivation of cellular genes and oncogenes | Certain functions may repair; one or more divisions may occur |
> 4–10 (>400–1,000) | Interphase death | No division |
500 (50,000) | Instant death | No division |
Proteins coagulate |
1.
Division delay: After exposure to radiation in the range of 0.5–3 Gy, delayed mitosis is observed; however, near normal restoration of mitotic activity is achieved following several generations.
2.
Reproductive failure: The failed mitotic activity is permanent and eventually cell death ensues. This is observed in a linear fashion after exposure to more than 1.5 Gy. Below this level the reproductive failure is random in nature and nonlinear.
3.
Interphase death: Apoptosis, or programmed cell death, is defined as a particular set of microscopic changes associated with cell death. Radiation-induced apoptosis is highly related to the type of involved cell. Lymphocytes, for example, are highly susceptible to radiation by this mechanism.
21.5 Various Effects of Radiation
The biological effect of low-level radiation is extremely difficult to study in a controlled environment. The effects of high radiation exposure to populations during accidents or nuclear war have been the main source of information.
At low doses, radiation can trigger only partially understood effects that can lead to cancer or genetic damage. These effects take years or generations to appear. At high doses, the effect may become evident within minutes, hours, or days. It is important for physicians to be familiar with the early effects of high radiation doses (1 Gy or more to the whole body), since the possibility that people may be exposed to such doses is increasing.
21.5.1 Dose-Response Models
There are many models predicting relationships between the radiation dose and the effect of such an exposure to a biological target. The differences between these models arise from different underlying assumptions. Figure 21.4 illustrates three models describing the response of a biological system to various radiation doses.
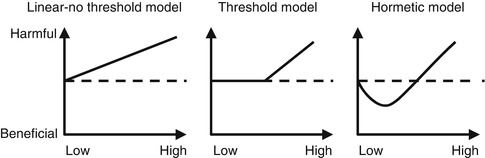
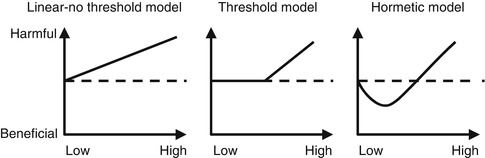
Fig. 21.4
Comparison between three different dose-response models. The dashed line represents health effects in the absence of radiation. y- and x-axes represent health effects and radiation dose, respectively (Reproduced from Ernest et al. [12] with permission)
1.
Linear No-Threshold Model: This model assumes that any level of radiation is harmful and that the risk increases linearly with increments of dose. This model is applied for radiation protection purposes and is meant to limit the risk to workers in radiation fields.
2.
Threshold Model: This model assumes that the risk of radiation is linearly related to the dose; however, this occurs only after a certain threshold level is exceeded. Below the threshold level, no risk is to be expected. The theory behind the threshold level is that some degree of cellular damage should accumulate and produce cell damage.
3.
Hormesis Model: In this model there is a bimodal effect of radiation, where below a certain threshold level radiation is protective, and harmful effects are seen only when this threshold is exceeded. The rationale is that radiation at low levels induces protective cellular mechanisms which prevent DNA damage occurring spontaneously or due to other stresses [13, 14] (Fig. 21.5).
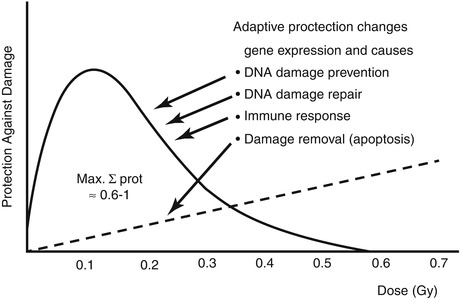
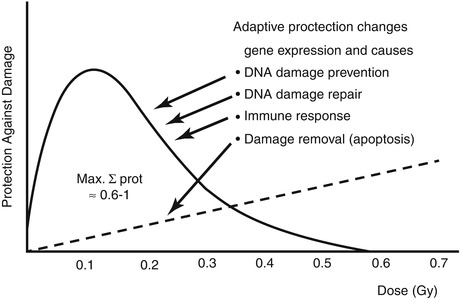
Fig. 21.5
Low-dose (low LET) induced adaptive protection. Scheme of dose-response function (Reproduced with permission from Feinenegen [15])
21.5.1.1 Early Radiation Effects
Acute Whole-Body Exposure Syndromes
Following exposure to a large, single, short-term whole-body dose of ionizing radiation, the resulting injury is expressed as a series of clinical symptoms. The sequence of events can be generally divided into four clinical periods.
1.
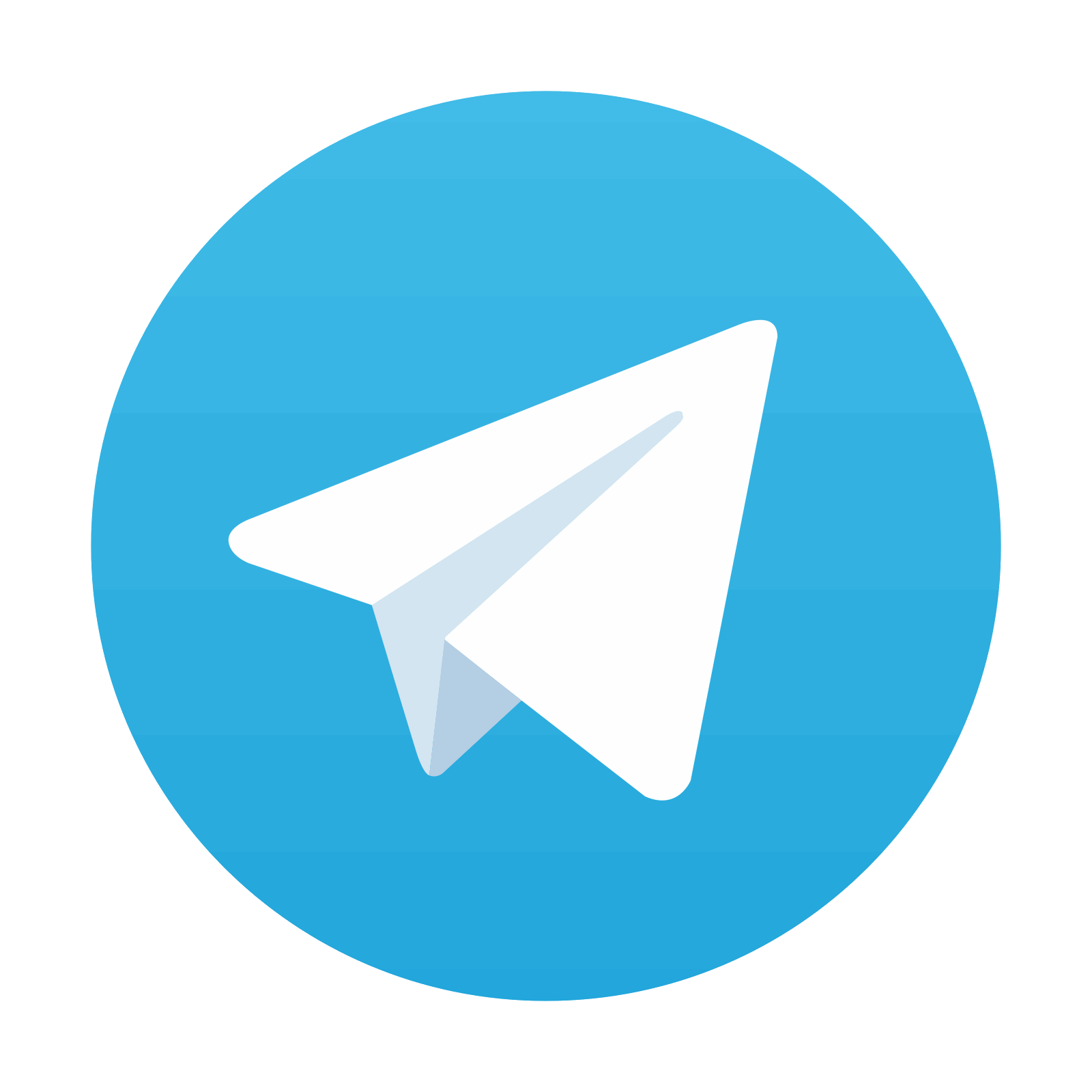
The prodromal period, up to 48 h, when the symptoms include anorexia, nausea, vomiting, and diarrhea.
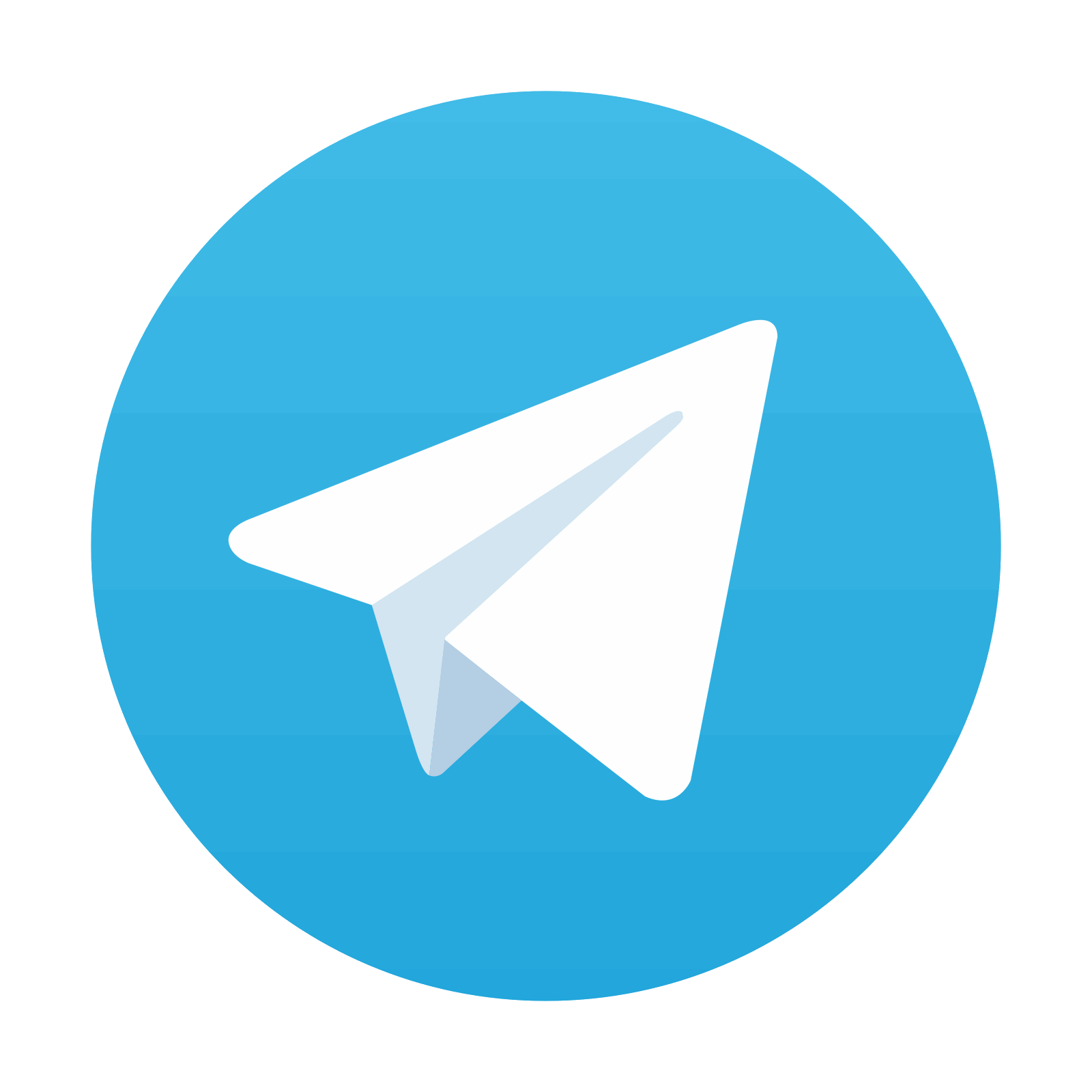
Stay updated, free articles. Join our Telegram channel

Full access? Get Clinical Tree
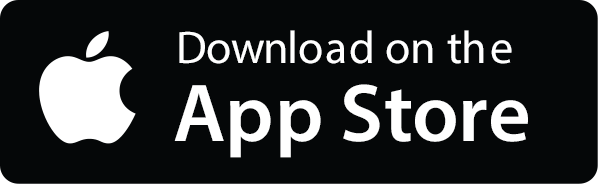
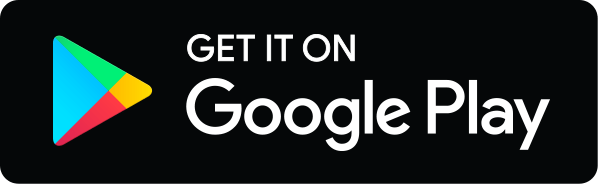
