Bronchoscopic Evaluation of the Airways with CT Correlations
In parallel with advances in multidetector computed tomography (CT) technology, including the ability to acquire volumetric datasets with near isotropic resolution coupled with advanced reconstruction techniques, similar improvements have occurred in the field of bronchoscopy. These include both improved diagnostic capabilities with sophisticated bronchoscopes coupled with video monitors and striking progress in the ability to perform therapeutic bronchoscopic interventions, (1,1a). It cannot be overemphasized that accurate assessment of the status of these techniques to evaluate the airways requires direct comparison of their capabilities and limitations (2). For this purpose, following a brief review of basic bronchoscopic technology, both rigid and flexible, basic principles of CT interpretation are discussed supplemented by a series of representative cases emphasizing CT-bronchoscopic correlations. The role of CT in guiding interventional bronchoscopic techniques is also reviewed, with particular emphasis placed on transbronchial needle aspiration and biopsy (TBNA) for assessing mediastinal and hilar disease as well as transbronchial biopsy (TBBx) of peripheral lung nodules. Current indications for CT versus bronchoscopy in patients presenting with hemoptysis are discussed. Finally, the application of new technologies to bronchoscopy including autofluorescence and endobronchial ultrasound (EBUS) is addressed.
BRONCHOSCOPY
Since its introduction by Gustave Killian (3) in 1896, the field of bronchoscopy has evolved into an indispensable tool for evaluating, diagnosing, and treating a wide variety of pulmonary and airway disorders.
Rigid Bronchoscopy
The rigid bronchoscope, which underwent many adaptations in the past century, remains remarkably true to Killian’s original design. This technique used a hollow metal tube, inserted through the mouth with the patient’s neck fully extended, to directly visualize the trachea and mainstem bronchi. Adequate illumination was challenging but sufficiently provided by indirect reflected artificial light; when small electric light bulbs became available, they could be attached at the proximal or distal ends of the bronchoscope for better illumination.
The modern rigid bronchoscope has easily overcome the problem of illumination by means of a light transmitted to the distal tip via fiberoptic bundles. Viewing the airway may be accomplished simply by looking through the tube from the proximal end as Killian did or, more commonly, by means of an optical “telescope” inserted through the bronchoscope to transmit the image to the viewer. Subsequent technologic advances permitted digital images obtained to be transmitted to a digital monitor for exceptionally sharp images of the airways.
In viewing the airways, the rigid bronchoscope still suffers from one of its original drawbacks: its rigidity limits the extent to which it can be advanced into the airways. In practice, no further than the truncus basalis of the right lower lobe and left lower lobe can be reached by a standard
rigid bronchoscope. This allows the bronchoscopist to view the proximal lower lobe airways as well as the proximal right middle lobe directly thorough the bronchoscope. Because insertion of the bronchoscope bypasses the upper lobes of each lung, the ability to view these airways through the rigid bronchoscope is limited. This is overcome to some extent by the use of the telescope, even more so if a “sideviewing” telescope is used. The latter provides the view of objects 90 degrees to the long axis of the telescope, in contrast to the “straight ahead” view of the standard telescope. In practice, the rigid telescope can be replaced by the passage of a flexible bronchoscope through the rigid bronchoscope, allowing easy access to airways not in the axis of the rigid bronchoscope.
rigid bronchoscope. This allows the bronchoscopist to view the proximal lower lobe airways as well as the proximal right middle lobe directly thorough the bronchoscope. Because insertion of the bronchoscope bypasses the upper lobes of each lung, the ability to view these airways through the rigid bronchoscope is limited. This is overcome to some extent by the use of the telescope, even more so if a “sideviewing” telescope is used. The latter provides the view of objects 90 degrees to the long axis of the telescope, in contrast to the “straight ahead” view of the standard telescope. In practice, the rigid telescope can be replaced by the passage of a flexible bronchoscope through the rigid bronchoscope, allowing easy access to airways not in the axis of the rigid bronchoscope.
A major advantage of the rigid bronchoscope over its flexible counterpart is its large inner diameter relative to that of the instrument channel of the flexible bronchoscope. Rigid bronchoscopes range in diameter from 3 to 9 mm, whereas the instrument channel of standard flexible bronchoscopes range in diameter from 1.2 to 3.2 mm. The rigid bronchoscope thus permits the passage of larger instruments such as forceps. In addition, large-bore suction tubes to aspirate secretions and blood can be passed through the rigid bronchoscope, whereas the suction capability of the flexible bronchoscope is governed by the diameter of the instrument channel. Massive hemorrhage, pathologic or iatrogenic, is therefore more easily managed with a rigid bronchoscope. The ability (indeed, the necessity) to ventilate through the rigid bronchoscope affords greater control over the airway to the bronchoscopist than does the flexible bronchoscope, although flexible bronchoscopy (FB) through an endotracheal tube approximates this action. Some bronchoscopists argue that this characteristic may make the rigid bronchoscope more suitable for certain interventional procedures, which is the subject of some debate.
The discomfort of both the necessary degree of neck extension and the passage of the large-caliber rigid bronchoscope through the pharynx, vocal cords, and trachea requires general anesthesia so that the patient can tolerate the bronchoscopy, which adds to the risk of the procedure.
Flexible Bronchoscopy
Shigeto Ikeda introduced FB in 1968. The first flexible bronchoscopes used fiberoptic bundles to transmit light from an external source to the distal tip of the bronchoscope and allowed the resultant view of the illuminated airways at the eyepiece at the proximal end of the bronchoscope. FB permits examination of virtually all bronchi large enough to admit the bronchoscope. Standard flexible bronchoscopes have an outer diameter (OD) range of 4.9 to 6.4 mm, which permit, in adult patients, entry into the fifth- or sixth-generation bronchus. Although only one practitioner at a time can look through the eyepiece, as with the original rigid bronchoscopes, the attachment of a lecture bronchoscope or “teaching head” with its own fiberoptic bundle leading to a second eyepiece permits others to see the bronchoscopic image.
The advent of digital technology provided Ikeda with the means for the next major advance in the capability of FB to provide airway images. He replaced the fiberoptic bundle with a charge-coupled device (CCD) at the distal tip of the flexible bronchoscope, which was wired to a television monitor. This provided several advantages to the bronchoscopist. The monitor obviously allows assistants and others to view the procedure, enhancing performance and its educational value. Recording of images was also simplified, and the recording options increased (see later discussion). Furthermore, the absence of an eyepiece moved the bronchoscopist’s face further from the bronchoscope, its instrument channel orifice, and the face of the patient. This decreased the risk of blood, secretions, or aspirated material accidentally splashing the bronchoscopist, significantly reducing the risk of transmission of infections that can penetrate mucous membranes.
Recent advances in digital technology have led to the acquisition of extremely detailed and accurate endobronchial images. Although it is intuitive to believe that such sharply rendered images will enhance the diagnostic and therapeutic abilities of FB, this theory remains to be borne out by formal investigation. In fact, perhaps a greater benefit of current technology lies in its educational value; the ease with which detailed bronchoscopic images can now be reproduced in journals and textbooks, shown in lectures, and transmitted via the Internet around the world could hardly have been imaginable when Ikeda invented FB.
LIMITATIONS OF STANDARD BRONCHOSCOPY
The limitations of airway imaging by the various modalities of bronchoscopy are both shared and unique. Depth of field is a major limiting factor for all bronchoscopic imaging. It is determined by the optical characteristics of the viewing mechanism. Depth of field for standard flexible bronchoscopes and video bronchoscopes is 3 to 100 mm.
Other limitations are related to the diameter of the bronchoscope, which limits the size of the airway that can be entered. Furthermore, airways that are acutely angled may be difficult to enter with the bronchoscope; this is particularly true in more peripheral airways, including the apical segments of both upper lobes. Even the ultrathin bronchoscope (discussed later), which is of a small enough diameter to reach the extreme periphery of the lung, may be difficult to pass into relatively proximal airways if the route requires multiple angulations. Finally, even large, bronchiectatic airways are often not visualized because the bronchoscopic route involves a more proximal airway too small to admit the bronchoscope.
IMAGE RECORDING AND STORAGE
Older versions of rigid and flexible bronchoscopes could be attached to somewhat cumbersome still and movie cameras, which nonetheless produced excellent pictures of the bronchoscopic views. As noted earlier, the advent of digital technology has vastly simplified the acquisition, management, storage, and sharing of bronchoscopic images. Both analog and digital movies of entire bronchoscopic procedures can be readily obtained. Still images can be taken during the procedure with the touch of a button located near the fingers of the hand controlling the bronchoscope or when reviewing the movies made during the procedure. Computerized databases are now available to provide an electronic record of a procedure, into which digital images can be incorporated to generate printed or stored reports. With appropriate security precautions to protect patient privacy, the electronic records may be integrated into hospitalwide electronic medical records, allowing all privileged users to access the bronchoscopic images on-site or (via the Internet) off-site.
ULTRATHIN BRONCHOSCOPY
Examination of the airways by standard flexible bronchoscopes is generally limited to the fourth- to sixth-generation airways because of the OD of the bronchoscope (4.9 to 6.2 mm). Recently so-called ultrathin bronchoscopes have been developed with ODs ranging from 3.6 to 2.2 mm. The smallest of these bronchoscopes permits visual examination of airways as distal as the tenth generation and beyond.
Ultrathin bronchoscopes with ODs of 3.8 to 2.7 mm and with instrument channels of 1.2 mm are now commercially available. With the exception of one 3.8-mm model that uses a CCD at the tip, all of these bronchoscopes are fiberoptic. This is a reflection of current technology that limits the smallest CCD that can be placed in a functioning bronchoscope. A hybrid model with a 2.8-mm OD uses fiberoptics to obtain the image at the distal tip and transmit the image to a CCD in the body of the bronchoscope, enhancing the image and obviating the attachment of a videocamera to the bronchoscope to transmit images to a video monitor.
Although the size of ultrathin bronchoscopes allows the routine examination of airways in the periphery of the lung, practical limitations accompany the advantages of their small OD. Ultrathin bronchoscopes are less stiff than standard bronchoscopes, so they may be difficult to maneuver if a particularly tortuous route is taken in the peripheral airways. The necessarily small diameter of the instrument channel severely limits the suction capability of ultrathin bronchoscopes, so that even tiny amounts of blood or secretions in the airways can significantly impair visibility. This potential problem is compounded by the nature of peripheral airways to readily collapse when suction is applied within, further impairing visibility. The smallest size ultrathin bronchoscope (2.2 mm) achieves its narrowness by eliminating the instrument channel altogether, thereby providing the bronchoscopist with no means to deal with blood or secretions.
Most early reports of the use of ultrathin bronchoscopes involved their use in the evaluation of large airways in a neonatal and pediatric population, in whom FB had not been feasible. Wood, (4,4a) found that the ultrathin bronchoscope was useful in facilitating endotracheal intubation, and Fan et al. (5) found it useful in evaluating sources of persistent wheezing. Monrigal and Granry (6) used a 2.2-OD bronchoscope to monitor the patency of the trachea and mainstem bronchi during the surgical removal of compressive subcarinal bronchogenic cysts in two very young children. The more recent introduction of an ultrathin bronchoscope with an instrument channel enhanced the diagnostic and therapeutic capabilities of ultrathin bronchoscopy in infants by allowing bronchoalveolar lavage (BAL), suctioning of mucus plugs causing atelectasis, and even selective bronchography (7). Stenotic central airways in adults present challenges to FB similar to those posed by normal airways in infants, so it is not surprising that the role of ultrathin bronchoscopy has been investigated in this setting. Schuurmans et al. (8) used a 2.8-mm ultrathin bronchoscope to examine 24 patients with central airway obstruction due to both benign and malignant causes, with a mean degree of occlusion of 84%. The ultrathin bronchoscope was able to pass through the obstruction in all but three patients, allowing assessment of distal airways and assisting in the planning of appropriate management in 87%.
Kikawada et al. (9) recently described their findings in the peripheral airways of patients with chronic obstructive pulmonary disease. Passage of an ultrathin bronchoscope to the eleventh- or twelfth-generation airway revealed frequent obstruction and granular mucosal changes in patients with chronic bronchitis and “net-like” changes and obstruction in patients with emphysema.
The excitement over the expansion of bronchoscopic capability to a new array of peripheral airways in adults is tempered by practical limitations imposed by the tracheobronchial tree itself. The examination of every branch of the tracheobronchial tree accessible by an ultrathin bronchoscope would surely test the patience of both the bronchoscopist and the patient. In addition, each subsequent branching of the airways makes it easier for the bronchoscopist to become confused as to the particular airway into which the bronchoscope is inserted. For these reasons most studies of the utility of ultrathin bronchoscopes in peripheral airways in adults have focused on the ability of the bronchoscope to access a particular airway, usually one
leading to a focal lung lesion, and the optimal means of guiding the bronchoscope to that airway.
leading to a focal lung lesion, and the optimal means of guiding the bronchoscope to that airway.
One study investigated the use of an ultrathin bronchoscope to identify airways leading to parenchymal lung lesions suspicious for malignancy to aid in immediately subsequent standard bronchoscopy with TBBx (10). The lesions ranged in size from 1.5 to 7.0 mm and were visible on fluoroscopy, which was used to guide the ultrathin bronchoscope to the lesion. Lesions were directly visualized by ultrathin bronchoscope in 4 of 17 patients. After removal of the ultrathin bronchoscope, a standard bronchoscope was inserted to follow the pathway of the ultrathin bronchoscope, and TBBx was performed. TBBx established a diagnosis in 11 of 17 patients (65%) (10). More recent studies investigated the use of ultrathin bronchoscopy in the diagnosis of nodules detected by CT that were too small to be visualized by fluoroscopy. As discussed in greater detail later, this approach is feasible only because of the introduction of virtual bronchoscopic evaluation of peripheral airways.
CT-BRONCHOSCOPIC CORRELATIONS
Diagnostic Correlations: Central Airway Disease
The accuracy of CT to evaluate the central airways was well established even before the introduction of multidetector CT scanners (11, 12, 13, 14, 15, 16). Its exceedingly high sensitivity, especially for lesions greater than 5 mm, is easily explicable because air represents a perfect natural contrast agent for CT: Combined with intrinsic high-contrast resolution provided by the interface between luminal air and adjacent mediastinal soft tissues, CT allows the precise location and extent of disease both within and surrounding the central airways.
Nonetheless, despite our current ability to obtain near isotropic images of the airways in a single breathhold period with submillimeter resolution, important limitations remain. CT is of limited value, for example, in the detection of endobronchial lesions smaller than 2 to 3 mm (Fig. 2-1). In a prospective study of 105 lesions in 98 patients with
radiographically occult squamous cell carcinomas identified on the basis of positive sputum cytology, Usuda et al. (17) showed that 27 lesions (26%) proved to be smaller than 2 mm, whereas 23 (22%) were endoscopically invisible, being identified by bronchial brushing alone. It is unlikely that CT would have been of value in detecting these lesions. That CT is limited in the detection of subtle endobronchial lesions is also documented by the results of low-dose CT screening trials. To date, CT has proved ineffective in diagnosing central endobronchial lesions in this population (18, 19, 20). Finally, subtle irregularities of the airway walls, often the result of prominent bronchial cartilage, or partial volume averaging in airways coursing obliquely also limit diagnostic accuracy.
radiographically occult squamous cell carcinomas identified on the basis of positive sputum cytology, Usuda et al. (17) showed that 27 lesions (26%) proved to be smaller than 2 mm, whereas 23 (22%) were endoscopically invisible, being identified by bronchial brushing alone. It is unlikely that CT would have been of value in detecting these lesions. That CT is limited in the detection of subtle endobronchial lesions is also documented by the results of low-dose CT screening trials. To date, CT has proved ineffective in diagnosing central endobronchial lesions in this population (18, 19, 20). Finally, subtle irregularities of the airway walls, often the result of prominent bronchial cartilage, or partial volume averaging in airways coursing obliquely also limit diagnostic accuracy.
In addition, with rare exceptions CT is unable to distinguish between mucosal and submucosal disease (1). CT evidence of smooth airway narrowing, for example, may be associated bronchoscopically with endobronchial, submucosal, or peribronchial disease (Fig. 2-2). Exceptions may be found. Although specific identification of lesions restricted to the airway mucosa remains elusive, lesions that lie internal to tracheal or bronchial cartilages, for example, may be characterized as either mucosal and/or submucosal in location (Fig. 2-3). Other exceptions include the finding of a discrete calcific endobronchial filling defect, as may occur, for example, in patients with an aspirated foreign body (Fig. 2-4) or broncholiths (21). In the latter case, CT may prove more sensitive than bronchoscopy by identifying both intraluminal and peribronchial calcifications distal to inflamed and narrowed airways rendered inaccessible to bronchoscopy. Also included are endobronchial hamartomas within which fat may rarely be identified (22). However, these abnormalities are unusual.
In comparing CT findings with abnormalities identified at bronchoscopy, it is essential to use appropriate descriptive terminology. This requires that a specific CT classification be used to describe abnormalities identified on routine axial images or multiplanar reconstructions (Fig. 2-2) (15). CT-bronchoscopic correlation using virtual bronchoscopy, although promising, remains a specialized technique for which a specific terminologic classification has yet to be devised (see Chapter 1). With these caveats in mind, the advantages and limitations of CT-bronchoscopic correlations for identifying airway pathology are well illustrated in a series of cases presented in Figs. 2-4, 2-5, 2-6, 2-7, 2-8, 2-9, 2-10 and 2-11.
![]() Figure 2-2 CT classification of airway pathology. A to H: Diagrammatic representations of the appearance of various airway abnormalities as seen on CT sections. In each case, abnormalities are represented as they would appear in axial, oblique (A, B), and sagittal planes. Note that this classification makes no attempt to correlate CT findings with a diagnosis of mucosal or submucosal disease. With rare exception, this distinction is typically impossible to establish (See Fig. 2-3). |
![]() Figure 2-3 CT-bronchoscopic correlation: mucosal/submucosal tumor. A and B: Coronal and axial drawings, respectively, showing a schematic representation of endoluminal mucosal/submucosal tumor. Although CT does not typically allow a specific diagnosis of mucosal or submucosal disease (Fig. 2-1), one exception is the presence of tumor internal or central to calcified tracheobronchial cartilage. C, cartilage; L, lumen; M, endoluminal mass. C: Contrast-enhanced CT section shows extensive mediastinal tumor. In this case, tumor can be identified interior to the left mainstem bronchial cartilage (arrow), allowing accurate mucosa/submucosal localization, subsequently verified endoscopically. |
Diagnostic Correlations: TBNA
The use of CT in lymph node “mapping” to aid in the planning and performance of TBNA may be considered a paradigm for the integration and correlation of CT and bronchoscopic imaging.
The current state of TBNA is largely the result of the pioneering efforts of Ko-Pen Wang (23, 24, 25) in the development of both the technique and the instruments. Over the past two decades, numerous reports have documented the value of this procedure (26, 27, 28, 29, 30, 31, 32, 33, 34).
TBNA allows cytologic and/or histologic sampling, most often of mediastinal and hilar tissue, in select cases obviating more invasive surgical procedures including mediastinoscopy or thoracotomy. TBNA can access more lymph node stations than any other biopsy technique and has the lowest complication rate as well (35). CT may also be of particular value by identifying cases in which TBNA is not indicated. This includes patients with negative CT scans, for whom the yield has proved sufficiently low to obviate this procedure (28). As important, CT can also help to avoid potentially false-positive biopsies by identifying parenchymal tumors that abut the central airways and making TBNA staging unreliable, as may occur in patients with tumors in the medial portion of the right upper lobe simulating right paratracheal adenopathy (36).
To date, TBNA has most often been used to evaluate patients with suspected intrathoracic neoplasia. In most series, TBNA has resulted in a yield of 60% to 80% sensitivity for diagnosing and staging lung cancer. In one recent multicenter trial TBNA resulted in an overall yield of 48% in 279 patients with non-small cell lung cancer (NSCLC), and 62% in 81 patients with small cell carcinoma among four institutions. As important, TBNA also provided the only bronchoscopic specimen diagnostic for lung cancer in 18% of patients (37). In an assessment of 123 patients with documented malignancy evaluated by TBNA, Sharafkhaneh et al. (31) reported a sensitivity of 69%, with significant correlations noted between a positive TBNA and cell type (p <.001) and nodal size (p <.05). Consistent with similar previous reports, this study also found that TBNA was most often successful in diagnosing small cell carcinoma compared with NSCLC and least successful for diagnosing lymphoma. Similar results have been reported by Khoo et al. (33) and Cetinkaya et al. (34). In a study of 45 consecutive cases evaluated over a 2-year period by a group of researchers with relatively less experience, TBNA provided an overall diagnosis in 65% of cases (33). Similarly, Hermens et al. (32), in a study of 106 consecutive patients with suspected lung cancer considered eligible for TBNA that were evaluated by six different pulmonologists in a non-university teaching hospital, reported an overall sensitivity of 65%, with malignancy demonstrated in 63 (59%) of 106 cases. Importantly, in this same study, a total of 10 mediastinoscopies were avoided because TBNA demonstrated contralateral N3 disease, leading these investigators to conclude that TBNA is a cost-effective method for staging lung cancer.
Although TBNA is most often performed to diagnose and/or stage patients with suspected intrathoracic neoplasia, it cannot be overemphasized that TBNA is also of great utility for diagnosing benign diseases as well, especially tuberculosis (TB) and sarcoidosis (38,39). Cetinkaya et al. (34), for example, used a 22-gauge Wang cytology needle to evaluate 60 consecutive patients with mediastinal or hilar adenopathy. TBNA successfully diagnosed TB in 13 of 20 cases (65%) and provided the sole means of diagnosis in 8 (40%). In this same study, TBNA also provided the only means of diagnosis in 13 (62%) of 21 patients with documented sarcoidosis. Use of TBNA for establishing a diagnosis of TB may be of particular value in patients with AIDS (39). Especially in the setting of immunocompromised patients, active tuberculous nodes have been noted to characteristically appear as low density on CT with an enhancing rim following intravenous administration of contrast media (40). As documented by Harkin et al. (39), TBNA proved diagnostic in 23 (51%) of 45 procedures performed in 41 human immunodeficiency virus (HIV)-positive patients with mediastinal adenopathy, including identification of 21 of 23 documented cases of mycobacterial infection. In this latter group TBNA provided the only diagnostic specimen in 13 (57%). More recently, Bilaceroglu et al. (41) similarly found TBNA to have a sensitivity and specificity for TB of 83% and 100%, respectively, in 76 HIV-negative patients. A rapid diagnosis was obtained in 78%, with TBNA providing the exclusive diagnostic specimen in 68% of cases.
Nearly identical data have also been reported recently by Trisolini et al. (30). In this study of 55 patients with CT evidence of enlarged mediastinal or hilar nodes with clinically suspected sarcoidosis, TBNA showed nonnecrotizing granulomas in 23 (72%) of 32 patients with subsequently documented sarcoidosis. Importantly, of 15 patients undergoing both TBNA and TBBx, TBNA provided the sole means of diagnosis in 7, improving the diagnostic rate by 47%. Similar results have previously been reported by Morales et al. (38) who found that of 51 consecutive patients with suspected sarcoidosis undergoing both TBNA and TBBx, 23% of patients with stage 1 disease and 10% of patients with stage 2 disease had their diagnoses established only by TBNA. Overall, combined TBNA and TBBx yielded a diagnosis in 83% of documented cases.
Although most investigators have argued for routine use of TBNA, regrettably this procedure remains underused by most bronchoscopists. In a survey of 871 North American bronchoscopists, only 12% used TBNA routinely in bronchoscopies for malignancy and only 2% used TBNA in benign disease (42). One of the primary reasons cited for the grudging acceptance of TBNA is the low yield that many practitioners have experienced. This may be the result of two primary factors: unfamiliarity with the technique and the inability to localize unseen lymph nodes relative to bronchoscopically visualized airways. Although the technique generally requires some degree of hands-on training, relating lymph node location to endobronchial anatomy remains an ongoing concern. TBNA was originally designed
as a blind technique, that is, using imagination, the bronchoscopist had to visualize the location of enlarged lymph nodes using previously obtained CT images. Wang (43) carefully related lymph nodes in standard locations adjacent to the airways to standard endobronchial landmarks and correlated this to American Thoracic Society (ATS) lymph node staging of NSCLC. In our experience, intimate knowledge of the lymph node map proposed by Wang and its correlation to CT findings is essential for TBNA to be successful when performed as a blind procedure, as is true of the overwhelming majority of cases.
as a blind technique, that is, using imagination, the bronchoscopist had to visualize the location of enlarged lymph nodes using previously obtained CT images. Wang (43) carefully related lymph nodes in standard locations adjacent to the airways to standard endobronchial landmarks and correlated this to American Thoracic Society (ATS) lymph node staging of NSCLC. In our experience, intimate knowledge of the lymph node map proposed by Wang and its correlation to CT findings is essential for TBNA to be successful when performed as a blind procedure, as is true of the overwhelming majority of cases.
![]() Figure 2-6 CT-bronchoscopic correlations: tracheobronchopathia osteochondroplastica (TO). A: Magnified view of the midtrachea imaged with narrow windows shows characteristic appearance of calcified nodules resulting in mild narrowing of the tracheal lumen. Note the absence of involvement of the posterior membranous tracheal wall. This proved to be an incidental finding. B: Corresponding flexible bronchoscopic evaluation demonstrates precise CT-endoscopic correlation with characteristic nodular excrescences protruding into airway, pathognomonic of this disease. Spirometry and flow-volume loop revealed minimal obstructive dysfunction (see, in addition, Fig. 3-33). |
CT-Bronchoscopic Reference Points for TBNA
The nomenclature and endobronchial landmarks for the nodal stations are oriented to the bronchoscopist’s perspective. The best use of this system is not to replace the standard ATS staging system (44), which is oriented to surgical and radiologic staging, but to identify those ATS nodal stations that are accessible from within the airways. In our opinion, familiarity with these CT-bronchoscopic correlations is essential for improving the diagnostic yield of TBNA. There are four bronchoscopic sites from which 11 lymph node stations can be sampled: the carina, the right upper lobe bronchus, the bronchus intermedius, and the left-sided secondary carina (Figs. 2-12, 2-13, 2-14 and 2-15) (35,43a). The reference point for the first six nodal stations is the carina (Fig. 2-12). Viewing the circumference of the trachea as a clockface, the carina is oriented in a 12 o’clock to 6 o’clock direction. The anterior carina lymph node is sampled by puncturing the carina at the 12 to 1 o’clock position and the posterior carina lymph node at the 5 to 6 o’clock position. The anterior carina nodes are commonly called pretracheal or precarinal by radiologists. The posterior carina position should be punctured only in the setting of adenopathy demonstrated on CT, because a pneumothorax may otherwise result. The right paratracheal lymph node corresponds
to ATS station 4R and is sampled through the second, third, or fourth intercartilaginous space of the lower trachea (counting proximally from the carina) at 1 o’clock. The left paratracheal lymph node is designated 4L in the ATS system and is punctured at the 9 o’clock position in the trachea at about the level of the carina or the tracheobronchial angle. The aorta or the pulmonary artery may be inadvertently punctured at this site, if the needle is placed too proximally or distally, respectively. The right and left main bronchus stations correspond to ATS stations 10R and 10L, respectively. These nodes may be sampled by puncturing the bronchus at 12 o’clock in the first intercartilaginous space distal to the carina. All these nodal stations are mediastinal in location, and a positive aspirate indicates at least N2 staging.
to ATS station 4R and is sampled through the second, third, or fourth intercartilaginous space of the lower trachea (counting proximally from the carina) at 1 o’clock. The left paratracheal lymph node is designated 4L in the ATS system and is punctured at the 9 o’clock position in the trachea at about the level of the carina or the tracheobronchial angle. The aorta or the pulmonary artery may be inadvertently punctured at this site, if the needle is placed too proximally or distally, respectively. The right and left main bronchus stations correspond to ATS stations 10R and 10L, respectively. These nodes may be sampled by puncturing the bronchus at 12 o’clock in the first intercartilaginous space distal to the carina. All these nodal stations are mediastinal in location, and a positive aspirate indicates at least N2 staging.
The next two stations use the right upper lobe bronchus as a reference. The right upper hilar node, ATS station 11R,
is punctured through the anterior part of the carina between the right upper lobe and the bronchus intermedius. The superior pulmonary vein may be punctured at this site, and reference to CT ensures that the node is anterior to the airway (Fig. 2-13). The subcarinal station, level 7 in the ATS system, is accessed at 9 o’clock within the right main bronchus proximal to the right upper lobe orifice.
is punctured through the anterior part of the carina between the right upper lobe and the bronchus intermedius. The superior pulmonary vein may be punctured at this site, and reference to CT ensures that the node is anterior to the airway (Fig. 2-13). The subcarinal station, level 7 in the ATS system, is accessed at 9 o’clock within the right main bronchus proximal to the right upper lobe orifice.
The next reference point is the bronchus intermedius (Fig. 2-14). The right lower hilar node is punctured at the 3 o’clock, and the sub-subcarina is sampled at 9 o’clock. These nodes are often extensions of those sampled higher in the right mainstem, although they can be solely enlarged. The right lower hilar and sub-subcarinal nodes may extend below the level of the right middle lobe orifice. Nonetheless, right lower hilar nodes represent N1 classification, while the sub-subcarinal nodes represent N2 classification.
The last node in the endobronchial map is the left hilar node—ATS station 11L—which is located at the carina
between the left upper and lower nodes (Fig. 2-15). This node is best approached from the origin of the lower lobe bronchus at about the 9 o’clock position.
between the left upper and lower nodes (Fig. 2-15). This node is best approached from the origin of the lower lobe bronchus at about the 9 o’clock position.
It is important to emphasize again that selection of the optimal puncture site is based on combining the knowledge of endobronchial landmarks with the location of enlarged lymph nodes on CT. In mentally planning the procedure, it is often helpful to flip the CT scan (which is imaged as if looking up from the patient’s feet) right to left, so that the view corresponds to the bronchoscopist’s viewpoint looking down from the patient’s head.
Alternative Methods for Performing TBNA
In an attempt to overcome dissatisfaction with the frequently blinded nature of standard TBNA, various imaging strategies have been used. These include direct CT guidance—with and without the use of CT fluoroscopy—as well as virtual bronchoscopic and endosonographic guidance (Fig. 2-16). Although the importance of these newer approaches may be limited for experienced bronchoscopists, for whom yields in the range of 85% to 90% have been reported, considerable promise for their use may result for less experienced bronchoscopists.
One method that may aid in increasing the yield of TBNA is CT guidance, that is, performing TBNA with the patient in the CT scanner to confirm puncture of the targeted lymph node. Rong and Cui (45) found this technique improved their yield to 60%, compared with their prior experience of 20% yield using the blind technique. One drawback of the use of direct CT guidance is that it is not performed in real time: Using this technique, additional images must be obtained and reconstructed following placement of the needle, during which time the patient may move. In distinction, CT-fluoroscopy provides real-time cine visualization of the tip of the needle, allowing more confident localization. Cytologic and/or histologic material is then aspirated under direct visualization. It has been demonstrated by CT-fluoroscopy that 41% of 116 needle passes were improperly positioned, whereas subsequent passes established diagnoses in enlarged lymph nodes in all 12 patients studied (46). All patients in this group had previously undergone a nondiagnostic TBNA by the conventional method. Mean fluoroscopic exposure time was 20.5 ± 12.7 seconds. Another group reported a yield for CT-fluoroscopy guided TBNA of 83% in 12 patients, all of whom had also undergone a nondiagnostic TBNA by the conventional method (47). Although these are excellent results for patients with nondiagnostic TBNA by experienced bronchoscopists, CT-fluoroscopy is not readily available and adds considerable expense to the procedure. It has
not yet been compared head-to-head with conventional TBNA.
not yet been compared head-to-head with conventional TBNA.
More recently, it has been proposed that virtual bronchoscopy may be an aid in planning and performing TBNA (Fig. 2-16) (48,49). As reported by McAdams et al. (50), in a preliminary investigation of virtual bronchoscopic guidance, TBNA was performed in 17 patients, including 16 patients without prior TBNA and one with two previous nondiagnostic TBNAs (50). Using manual tracings of enlarged nodes on every third axial image, endoluminal simulations were created showing the position of nodes relative to virtual bronchoscopic images and displayed in green. These were available for review before TBNA. Altogether, 18 nodes ranging from 1.0 to 5.0 cm were aspirated in 17 patients: Of these, 14 (77%) were positive for malignancy, with diagnoses ranging from metastatic breast cancer to well-differentiated lymphocytic lymphoma (50). Two false-negative aspirates were also noted, including one patient with nodular sclerosing Hodgkin’s disease ultimately diagnosed surgically and one patient with metastatic melanoma.
In addition, virtual bronchoscopy has been reported to play a role in the preplanning stage to improve the yield of TBNA (51,52). In one recent study (53), volumetric
rendering was used to first highlight a total of 36 sites in 14 patients in whom enlarged nodes could be identified on routine 3-mm axial images: Two separate sets of virtual bronchoscopic images—one without and one with and without nodal highlighting—were subsequently created and viewed by three experienced pulmonologists. Although virtual bronchoscopy added little to the choice of biopsy site for subcarinal nodes and did not significantly alter the selection of optimal sites for sampling aorticopulmonary window nodes, use of virtual bronchoscopic imaging with nodal highlighting increased the successful choice of biopsy sites for hilar nodes (from 37% to 83%; p = .001) and for pretracheal nodes (from 59% to 85%; p = .07), respectively.
rendering was used to first highlight a total of 36 sites in 14 patients in whom enlarged nodes could be identified on routine 3-mm axial images: Two separate sets of virtual bronchoscopic images—one without and one with and without nodal highlighting—were subsequently created and viewed by three experienced pulmonologists. Although virtual bronchoscopy added little to the choice of biopsy site for subcarinal nodes and did not significantly alter the selection of optimal sites for sampling aorticopulmonary window nodes, use of virtual bronchoscopic imaging with nodal highlighting increased the successful choice of biopsy sites for hilar nodes (from 37% to 83%; p = .001) and for pretracheal nodes (from 59% to 85%; p = .07), respectively.
Although a variety of newer techniques are being developed to provide a real-time interface between virtual bronchoscopy and TBNA, including the use of internal and external fiduciary markers and external electromagnetic guidance, among others, these currently remain largely experimental (54, 55, 56, 57). The role of endoscopic ultrasonography for performing transbronchial needle aspiration is discussed separately in a later section.
Diagnostic Correlations: Peripheral Disease-Pulmonary Nodules
Clinical assessment of patients with parenchymal nodules presents an important problem in pulmonary diagnosis. It is estimated that nearly 130,000 individuals, or 52 per 100,000 people present yearly with radiographic evidence of focal parenchymal disease, nearly half of which will prove to be malignant (58). Despite the efficacy of flexible FB to identify and diagnose central endobronchial lesions, bronchoscopy is generally not considered as indicated for assessing peripheral nodules. It has been shown that in those cases in which a bronchus can be identified leading to a nodule—a “positive bronchus sign”—the yield of routine FB can be significantly improved (Fig. 2-17) (59, 60, 61, 62). Still better results have been reported when detailed bronchographic road maps are first acquired (60,63,64).
Although standard FB is of documented utility in assessing central endobronchial lesions within the visual range of the bronchoscope, it has proved less valuable in assessing peripheral lung nodules. Overall the yield of bronchoscopic biopsy of peripheral nodules has generally been reported to range between 40% and 80% (58). A number of factors account for this discrepancy, including small size, location (central vs. peripheral), benign versus malignant disease, the type of procedure performed (BAL, bronchial brushing, or bronchial needle vs. forceps biopsy), whether FB is performed under fluoroscopic guidance, and interbronchoscopist variability (65). Numerous studies have shown that size is the most important determinant of success of TBBx. The poorest results are consistently obtained in patients with peripheral lesions smaller than 2 cm (66, 67, 68). In a recent survey of 30 studies evaluating the performance characteristics of bronchoscopy for diagnosing peripheral lung nodules, Schreiber et al. (69) noted that FB proved 62% sensitive for lesions larger than 2 cm but only 33% sensitive for lesions smaller than 2 cm. Although the results of TBBx for lung nodules may be improved when performed under ultrasonographic guidance, this technique is not generally available, is expensive, and still requires clinical validation (70,71). Although the yield of FB is greater for malignant nodules, this likely reflects the fact that benign lesions tend to be smaller (67). It should be noted that there is wide variation in the manner in which “peripheral” is defined (66,72, 73, 74, 75, 76). Using CT criteria, Baaklini et al. (67) reported significant differences in FB yield when lesions of less than 2 cm were further divided into those in the peripheral one third of the lung compared with those in the middle third.
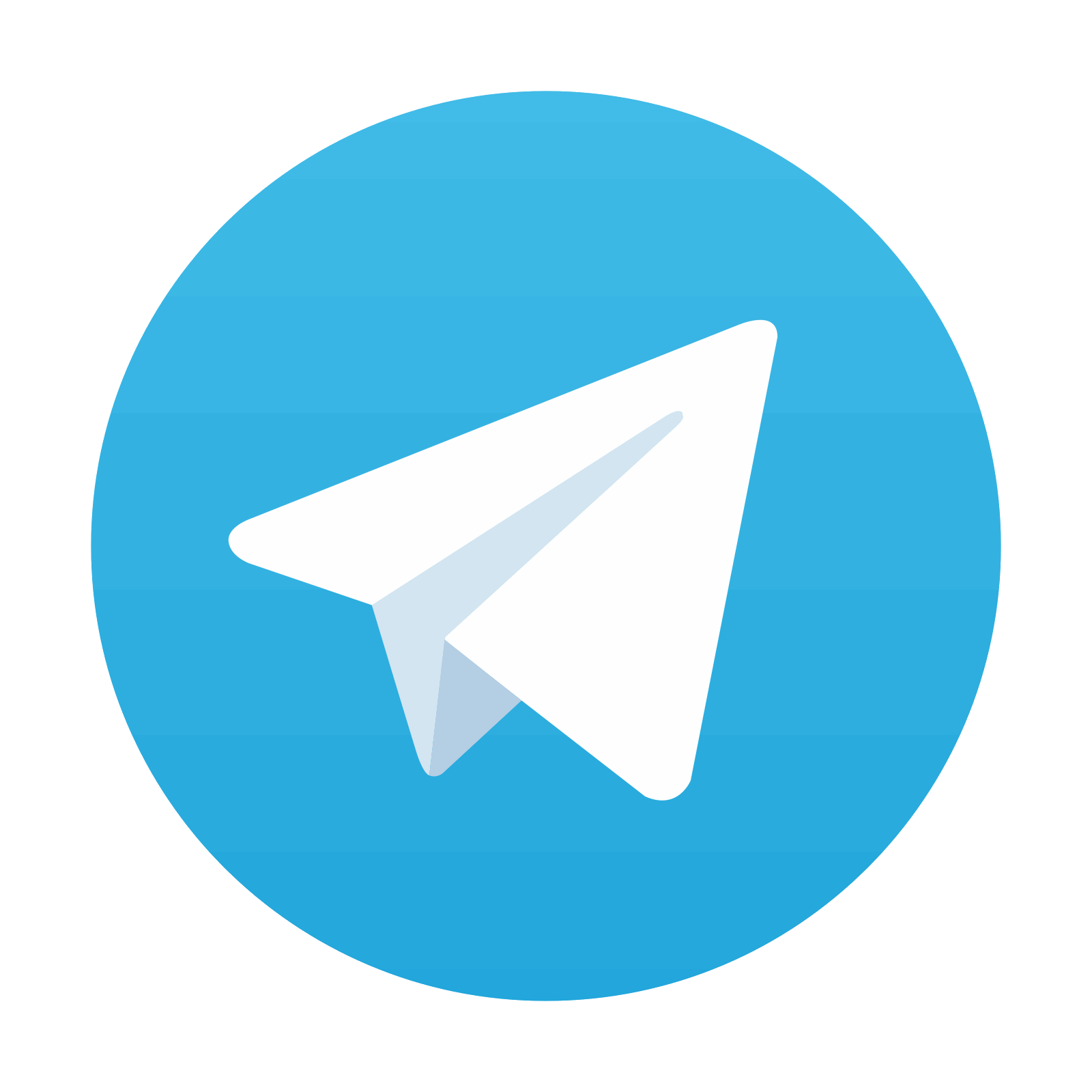
Stay updated, free articles. Join our Telegram channel

Full access? Get Clinical Tree
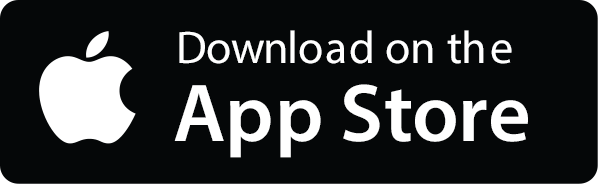
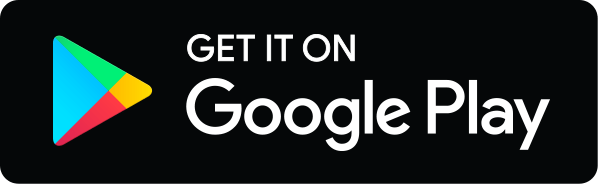